DOI:
10.1039/C4RA15590D
(Paper)
RSC Adv., 2015,
5, 19552-19569
[Et3NH][HSO4]-catalyzed eco-friendly and expeditious synthesis of thiazolidine and oxazolidine derivatives†
Received
1st December 2014
, Accepted 10th February 2015
First published on 10th February 2015
Abstract
The present study reports a facile and green approach for the synthesis of thiazolidine/oxazolidine derivatives 4(a–u) in excellent yields (92–98%) with high purity. The protocol involves a one-pot three-component reaction of substituted 1,3-diketones 1(a–g), cyanates 2(a–c) and ethylchloroacetate (3) in ionic liquid [Et3NH][HSO4] under solvent-free conditions. The notable feature of this pathway is that the ionic liquid possesses both catalytic as well as medium engineering capability in this protocol. Use of [Et3NH][HSO4] as a catalyst and an environmentally benign solvent eliminates the need for a volatile organic solvent and additional catalyst. This ionic liquid is air and water stable and easy to prepare from cheap amine and acid. The present synthetic route is a green protocol offering several advantages, such as excellent yield of products, mild reaction conditions, minimizing chemical waste, shorter reaction time, simple operational procedure, easy preparation of the catalyst and its recyclability up to five cycles without any noticeable loss in catalytic activity. The protocol is applicable to a broad substrate scope. The optimization of conditions carried out in the present study revealed that 20 mol% of ionic liquid catalyst under solvent-free conditions at 120 °C are the best reaction parameters for the synthesis of thiazolidine/oxazolidine derivatives in excellent yields.
Introduction
Over the past 20 years, there has been an upsurge in the field of green chemistry.1 Most of the efforts in this direction have been focused on replacing the profusely used toxic volatile organic solvents (VOS) by suitable alternate solvent systems for easy chemical transformations with the minimum of chemical waste and environmental pollution. In this regard, ionic liquids have attracted significant research interest in the context of green synthesis due to their adjustable physical and chemical properties.2 They have been introduced as an alternative green reaction medium due to their exceptional features such as the advantages of optimization of compound characteristics through a broad choice of anion and cation combinations, low vapor pressure, non-volatility, low flammability, good solvating ability, high thermal and chemical stability, controlled miscibility and ease of recyclability.3–12 The report of 1,3-dialkylimidazolium-based chloroaluminate ionic liquids by Wilkes et al., that possess favorable physical and electrochemical properties, provided the impetus for a remarkable increase in activity in this field.13,14 Ionic liquids have been used as environmentally benign solvents or catalysts,15 thermal fluids,16 sensors,17 fuel cells,18 capacitors,19 lubricants,20 batteries,21 plasticizers,22 and extractants.23 The first involvement of ionic liquids in 2003 for first industrial process by BASF (BASIL10 process) opened new avenues for the application of ionic liquids in new chemical processes.24 A number of ionic liquids (ILs) viz. [BHP-OMe][Br],25 [TMG][Ac],26 1-ethyl-3-methylimidazolium hydrogen sulphate,27 [Bmim][PF6],28 [Hbbim][BF4],29 [Bmim][BF4],30 and [Cmmim][BF4]31 have been documented in the literature employed for the synthesis of biologically active molecules (Fig. 1).
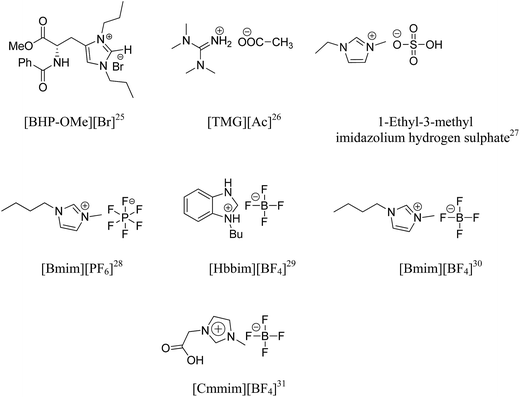 |
| Fig. 1 Ionic liquids (ILs) in synthetic transformations. | |
Although ionic liquids have been used as alternative reaction media and catalyst,15 their high cost, difficulty in separation and toxicity confine their applicability. Therefore, there is a demand to explore the cheap and easily available ionic liquids in organic synthesis. Bronsted acid ionic liquids (BAILs) are of exceptional significance as they possess simultaneously the proton acidity and the characteristic properties of an ionic liquid.32 These ionic liquids have been proved to be very efficient catalysts as well as solvents for many organic transformations.33–37
It is pertinent to mention that C–N/C–S bond is of significant importance, as it opens new avenues for the introduction of nitrogen/sulfur in organic molecules. Despite significant advancement in this field, the construction of the C–N/C–S bond is still a major challenge for organic chemists, due to the involvement of harsh reaction conditions or the use of expensive catalysts.38–40 In this regard, thiazolidine/oxazolidine derivatives constitute an important class of compounds in organic chemistry due to their promising biological activities.41 A plethora of medicinally important compounds bearing thiazolidine/oxazolidine moiety in their structural framework have been reported possessing anti-proliferative,42 analgesic,43 anti-HIV,44a,b anti-malarial,45 anti-convulsant,46 anti-microbial,47 COX-2 inhibitory,48 anti-histaminic,49 anti-parasitic50 and anti-oxidant51 activities (Fig. 2). The synthetic efforts for this class of compounds are very well studied and generally entail the reaction of carbonyl compounds with amine and mercaptoacetic acid/glycolic acid in organic solvents.52
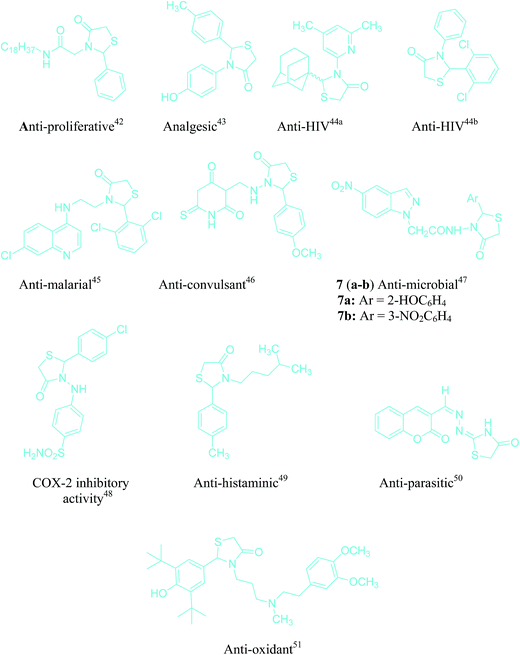 |
| Fig. 2 A few examples of pharmacologically active molecules bearing thiazolidine/oxazolidine moiety in their structural framework. | |
The catalyst promoted organic synthesis has become one of the hot areas in organic synthesis in the last few decades. At present, a wide range of methods for synthesizing thiazolidine/oxazolidine derivatives in the presence of catalysts are available viz. solid-phase/activation cycloelimination (SP/ACE) process,53 bisphosphine catalyzed,54 Bronsted acids,55 Pd-catalyzed,56 zeolite,57 sulfated tungstate,58 ZnCl2,59 SmI2,60 PBu3,61 LiBr,62 PPh3AuNTf2,63 AuPPh3Cl,64 NiI2,65 L-proline,66 PPh3.67 Although, these protocols reported by others find certain merits of their own, still they suffer from a number of shortcomings including prolonged reaction times, unsatisfactory yields, harsh reaction conditions, high temperature, use of hazardous organic solvents and expensive non-reusable catalysts.60,63,68
Thus, it is a challenge to develop alternative greener, milder, cheap and efficient methodologies for the construction of C–N/C–S bonds. To address the lack of convergent synthetic methods for the production of desired motifs, in this regard, ionic liquids (ILs) offer promising efficiency over other catalyzed reactions.69–71 Till now, a very few ionic liquids have been reported so far for the synthesis of thiazolidine/oxazolidine derivatives such as [Bmim][PF6],72,73 [TMG][Lac],74 (C3[min]2[Br]2),75 [Bnmim][Cl],76 2-HEAP,77 however these ionic liquids are of high cost as compared to simple ammonium ionic liquids. The first report documented so far for the synthesis of thiazolidinones involving [Et3NH][HSO4] was recently published by Maryam Kalantari78 involving reaction between thiosemicarbazide, dimethylacetylene dicarboxylate (DMAD) and carbonyl compounds (Scheme 1).
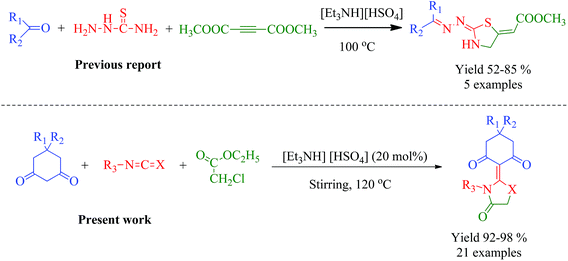 |
| Scheme 1 Strategy for the synthesis of C–N/C–X (O, S) bonds. | |
In continuation of our previous work on the progress of designing green synthetic methodologies for organic transformations,79,80 herein we report for the first time the development of an efficient, economical and recyclable Bronsted acid ionic liquid, [Et3NH][HSO4] promoted synthesis of thiazolidine/oxazolidine derivatives in excellent yields. The notable feature of this pathway is that, the ionic liquid possesses both catalytic as well as medium engineering capability in this protocol. In comparison with the current methods of thiazolidine/oxazolidine formation, our approach displays specific advantages: (i) it proceeds faster and gives excellent yields (92–98%); (ii) it requires an inexpensive catalyst; (iii) gives cleaner reaction profile with high purity (iv) it is applicable to a broader substrate scope (electron-rich and electron-deficient).
Results and discussions
Characterization of ionic liquid [Et3NH][HSO4]
The ionic liquid [Et3NH][HSO4] employed in the present study has been characterized on the basis of 1H NMR and 13C NMR spectral analysis. 1H NMR spectrum displayed a triplet at around δ 1.29 integrating for nine protons has been attributed to methyl group (3 × CH3) protons. Similarly a multiplet resonating at δ 3.13 for six protons has been assigned to methylene (3 × CH2) protons. A sharp singlet at δ 8.85 for one proton has been assigned to –NH (D2O-exchangeable) proton (Fig. S1†). 13C NMR spectrum of the [Et3NH][HSO4] showed a pair of signals resonating at around δ 10.27 and 52.09 assigned to methyl (CH3) and methylene (CH2) carbons, respectively (Fig. S2†). These spectral analysis suggested the formation of desired ionic liquid (IL) [Et3NH][HSO4].
Chemistry
The synthetic pathway for the synthesis of thiazolidine/oxazolidine derivatives 4(a–u) has been depicted in Table 1. Herein, a series was typically accessed via a facile condensation reaction between appropriately substituted 1,3-diketones 1(a–g), cyanates 2(a–c) and ethylchloroacetate (3) in [Et3NH][HSO4]. The present “one-pot synthesis” protocol provides easy access to the synthesis of desired products in excellent yields (92–98%) with high purity and was investigated to establish the feasibility and scope for broader substrate choice.
Table 1 Synthetic pathway for the synthesis of thiazolidine/oxazolidine derivatives 4(a–u)
The structural elucidation of the synthesized compounds 4(a–u) has been established on the basis of elemental analysis, IR, 1H NMR, 13C NMR and mass spectral study. The analytical results for C, H and N were within ±0.3% of the theoretical values and were found to be in conformity with the proposed molecular structures. IR spectrum of all the synthesized compounds displayed characteristic signals for carbonyl (C
O) and lactam (CO–N) linkage at around 1730–1749 and 1622–1647 cm−1, respectively. 1H NMR spectrum (see ESI†) of each compound exhibited a sharp singlet resonating at around δ 2.65–4.93 for two protons, has been assigned to methylene (H-5′) protons of the thiazolidine/oxazolidine ring. This downfield shift is attributed to the electron withdrawing nature of the adjacent carbonyl group. 13C NMR spectrum (see ESI†) was also in good agreement with the proposed structures displaying characteristic signals for carbonyl and lactam carbonyl at around δ 189.5–199.6 and 155.7–178.9, respectively. Similarly signals resonating at around δ 32.6–74.9 have been assigned to methylene carbon (–CH2) of the thiazolidine/oxazolidine ring. Moreover characteristic signals for aromatic ring carbons have been discussed in Experimental section. The mass spectral analysis of the synthesized compounds was also in good conformity with the proposed structures.
In our present study, a series of thiazolidine/oxazolidine derivatives 4(a–u) of substituted 1,3-diketones were synthesized by reaction between substituted 1,3-diketones 1(a–g), cyanates 2(a–c) and ethylchloroacetate (3) in DMF in the absence of ionic liquid [Et3NH][HSO4]. The reaction took stretched time period (5–7 hours) for completion with a moderate yield (65–75%) of the products (Table 2). The use of DMF as a solvent of choice for the comparative studies (Table 2) has been selected in view of its aprotic nature. Since, we believe that using common protic solvents (EtOH, MeOH) may react with the reactants in the reaction media that will eventually affect our reaction in terms of yield as there is the probability of by-product formation.81,82 Moreover, there are reports in the literature where almost analogues of thiazolidine/oxazolidine compounds have been synthesized in excellent yields using DMF as a solvent of choice.83,84
Table 2 [Et3NH][HSO4] catalyzed synthesis of thiazolidine/oxazolidine derivatives 4(a–u)
Products |
Structure |
Reaction in presence of DMF |
Reaction in presence of [Et3NH][HSO4] |
M.P (°C) |
Time (h) |
Yield (%) |
Time (min) |
Yield (%) |
4a |
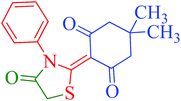 |
5 |
74 |
25 |
98 |
128–129 |
4b |
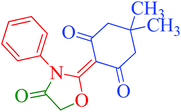 |
5.5 |
70 |
28 |
95 |
125–126 |
4c |
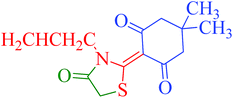 |
5 |
72 |
26 |
96 |
145–146 |
4d |
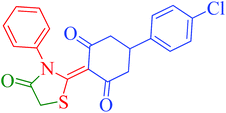 |
6 |
73 |
30 |
94 |
198 |
4e |
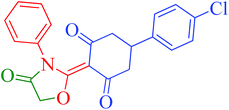 |
5.3 |
69 |
28 |
97 |
175 |
4f |
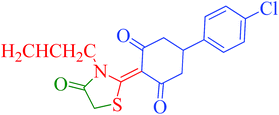 |
5.5 |
71 |
35 |
92 |
182 |
4g |
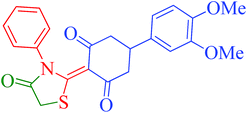 |
6 |
68 |
35 |
93 |
201 |
4h |
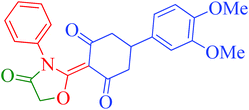 |
5.7 |
73 |
32 |
96 |
215 |
4i |
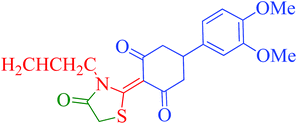 |
6.5 |
70 |
29 |
94 |
173 |
4j |
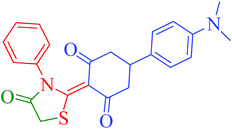 |
7 |
65 |
34 |
92 |
156 |
4k |
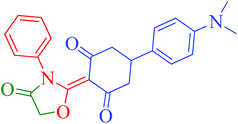 |
5 |
75 |
27 |
98 |
167 |
4l |
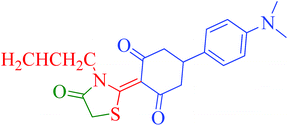 |
5.8 |
71 |
30 |
95 |
163 |
4m |
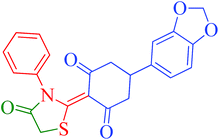 |
6 |
73 |
28 |
96 |
204 |
4n |
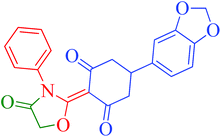 |
6.4 |
68 |
27 |
93 |
219 |
4o |
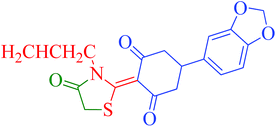 |
6.2 |
71 |
32 |
97 |
196 |
4p |
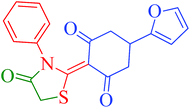 |
5.8 |
68 |
35 |
93 |
137 |
4q |
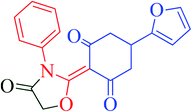 |
5.5 |
66 |
28 |
95 |
147 |
4r |
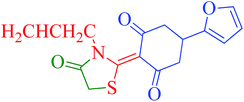 |
5.2 |
72 |
26 |
97 |
153 |
4s |
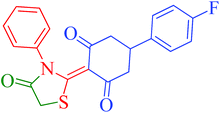 |
5.0 |
74 |
27 |
98 |
186 |
4t |
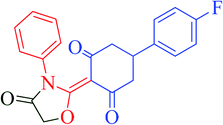 |
5.8 |
70 |
32 |
93 |
171 |
4u |
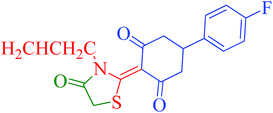 |
6.0 |
68 |
34 |
95 |
167 |
In order to develop an eco-friendly and efficient approach for the synthesis of biologically active thiazolidine/oxazolidine derivatives, we explored the efficiency of ionic liquid [Et3NH][HSO4] by carrying out one-pot condensation reaction of appropriately substituted 1,3-diketones, cyanates and ethylchloroacetate in equimolar ratio (2 mmol each). To explore the generality and scope of this reaction, different 1,3-diketones were examined under the optimized conditions and the results are summarized in Table 2. To our delight, this method was found to be very general for a wide range of 1,3-diketones with various substituents on the aromatic ring. In our experiments, we investigated the optimum reaction conditions regarding the choice of solvent, temperature of reaction and loading of catalyst on a model reaction using 5,5-dimethyl-cyclohexane-1,3-dione (1a), phenylisothiocyanate (2a) and ethylchloroacetate (3) to establish best reaction conditions in terms of yield and reaction time.
To achieve the optimum concentration of [Et3NH][HSO4], the model reaction was investigated initially in absence of [Et3NH][HSO4], it was found that reaction took prolonged time period (8 h) with impure products (Table 3, entry 1). The model reaction was then subsequently tested for different concentrations 5, 10, 15, 20 and 25 mol% (Table 3, entries 2–6) of [Et3NH][HSO4] at 120 °C under solvent-free condition. It is evident from (Table 3, entry 5) that 20 mol% of the [Et3NH][HSO4] is adequate to gain the optimum yield in the shortest reaction time (25 min). Using less than 20 mol% of catalyst, moderate yields of the product (57–85%) were obtained with extended reaction times, while with an excess mol% of catalyst (25 mol%) there was no further increase in the yield of the product, possibly due to the saturation of the [Et3NH][HSO4]. The above results signify that 20 mol% of [Et3NH][HSO4] is optimum dose in terms of efficient yield and reduced reaction time.
Table 3 Effect of catalyst loading on the yield and time period of model reaction (4a)a
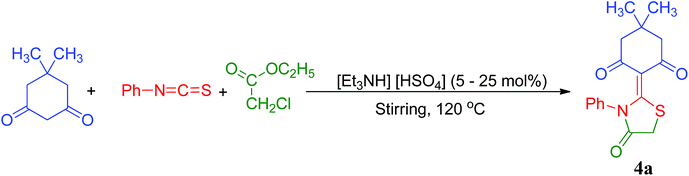
|
Entry |
Catalyst (mol%) |
Timeb (h) |
Yieldc (%) |
Reaction conditions: 5,5-dimethylcyclohexane-1,3-dione (1a, 2 mmol), phenylisothiocyanate (2a, 2 mmol) and ethylchloroacetate (3, 2 mmol), solvent free, 120 °C. Reaction progress monitored by TLC (entry 1–4 h). Isolated yield of products. Reaction progress monitored by TLC (entry 5–6 min). |
1 |
No catalyst |
8 |
Impure |
2 |
5 |
4 |
57 |
3 |
10 |
2.5 |
72 |
4 |
15 |
1 |
85 |
5 |
20 |
25d |
98 |
6 |
25 |
25d |
98 |
In order to study the effect of solvents, the model reaction was carried out in various conventional organic solvent systems. The model reaction was first investigated in DMSO and EtOH (Table 4, entries 1–2) the reaction took a longer time period (6–7 h) with moderate yields of 55% and 52%, respectively, whereas in DMF (Table 4, entry 3), the product 4a was obtained in better yield (74%) after refluxing for 4 h. In CH3CN and CH3NO2, moderate yields (61% and 63%) of the product 4a were obtained after a stretched reaction periods (Table 4, entries 4–5). On the other hand, toluene (C6H5CH3) gave comparable results in terms of yield 85% (Table 4, entry 6) with drop in reaction time (4 h). It is quite evident from the data reported in Table 4, that nature of solvent has a prominent effect on the kinetics as well as on the yield of the products. This variation in results (% yield of products) is believed to be due to polar/protic nature of the solvent systems used in the study. The results justify that nonpolar solvents such as toluene endowed excellent selectivity about 85% yield of the desired product 4a (Table 4, entry 6) with substantial dip in reaction time. However, toluene is not a solvent of choice for sustainable chemistry as it poses a serious threat to the environment.85 The variation of solvent system from nonpolar to polar (DMF, CH3NO2, CH3CN, DMSO and EtOH) endorses a reasonable fall in selectivity of product 4a as well as stretched reaction times (Table 4, entry 1–5). The results suggest that the yield of product 4a in different solvent systems follow the order as: nonpolar solvent (85%) > polar-aprotic solvent (55–74%) > polar-protic solvent (52%). The significant dip in the yield of product 4a (52%) (Table 4, entry 2) in polar-protic solvent (EtOH) is believed to be its protic nature that may react with the phenylisothiocyanate, rendering it unavailable (by-product) for the reaction with 1,3-diketones eventually will lower the yield of product.81 The selectivity would decrease largely, because of the formation of by-product O-ethyl phenylcarbamothioate. The percent of by-product formation can be minimized by choosing nonpolar solvent as a reaction media. However, toluene being environmentally toxic can allocate DMF as an optional solvent in the present study in absence of ionic liquid. On the basis of these findings it can be concluded that [Et3NH][HSO4] is the solvent of choice in comparison to conventional solvent systems.
Table 4 Effect of various solvents and temperature on the model reactiona
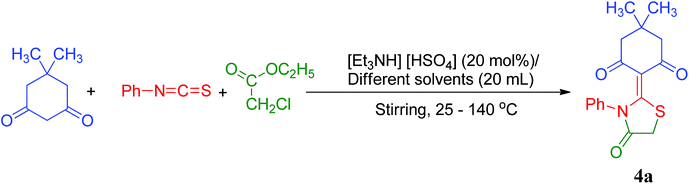
|
Entry |
Solvent |
Temp (°C) |
Timeb (h) |
Yieldc (%) |
Reaction conditions: 5,5-dimethylcyclohexane-1,3-dione (1a, 2 mmol), phenylisothiocyanate (2a, 2 mmol) and ethylchloroacetate (3, 2 mmol), different solvents (20 mL, entry 1–6, refluxing temperature), [Et3NH][HSO4] (20 mol%, entry 7–12, temperature 25–140 °C). Reaction progress monitored by TLC (entry 1–10 h). Isolated yield of products. Reaction progress monitored by TLC (entry 11–12 min). |
1 |
DMSO |
Reflux |
6 |
55 |
2 |
EtOH |
Reflux |
7 |
52 |
3 |
DMF |
Reflux |
5 |
74 |
4 |
CH3CN |
Reflux |
6.5 |
61 |
5 |
CH3NO2 |
Reflux |
6.5 |
63 |
6 |
Toluene |
Reflux |
4 |
85 |
7 |
[Et3NH][HSO4] |
Room temp. |
4 |
82 |
8 |
[Et3NH][HSO4] |
60 |
3 |
85 |
9 |
[Et3NH][HSO4] |
80 |
2 |
88 |
10 |
[Et3NH][HSO4] |
100 |
1.5 |
90 |
11 |
[Et3NH][HSO4] |
120 |
25d |
98 |
12 |
[Et3NH][HSO4] |
140 |
25d |
98 |
To optimize the reaction temperature, the model reaction was carried out at different temperatures in ionic liquid [Et3NH][HSO4] (Table 4, entries 7–12). It was observed that the increase in temperature from 25 °C to 120 °C, has a noteworthy effect on the model reaction in terms of yield and reaction time. The yield of the product increased from 82–98% during the course of reaction (Table 4, entries 7–11). However, no further enhancement in the yield of product 4a was observed when the reaction temperature was raised from 120 °C to 140 °C (Table 4, entry 12). In view of the above results, it was concluded that 20 mol% of ionic liquid [Et3NH][HSO4] mediated synthesis of 4a at 120 °C are the best reaction parameters for the synthesis of present thiazolidine/oxazolidine derivatives in excellent yields.
A comparative study of a variety of other Bronsted acid ionic liquid catalysts was conducted to probe the superiority of [Et3NH][HSO4]. It is apparent from (Table 5) that the catalytic activity was strongly affected by the anionic part of the ionic liquids. In case of [HSO4] anion, higher yields were obtained (Table 5, entries 1–3). However, when [H2PO4] and [CH3COO] anions were probed for their efficiency, lower yields (74–85%) were obtained as compared to [HSO4] anion (89–98%), probably due to the weaker acidity of the phosphate and acetate anions in comparison to [HSO4] anion. The results obtained in Table 5 validate that the anions of the ionic liquid (IL) have a notable effect than the cations on the reaction rates and selectivity. This can be presumably attributed to the pKa values (acid dissociation constant) of their respective anions (HSO4, H2PO4 and CH3COO) which offers a quantitative measurement of the strength of an acid, smaller the pKa value, stronger is the acid. The results (% yield of products) obtained in Table 5 are in good agreement with the pKa values of their respective anions which follow the order as: HSO4 (pKa = −3) > H2PO4 (pKa = 2.15) > CH3COO (pKa = 4). The size of the alkyl chain tethered to the cationic part of ionic liquid (IL) endorse no significant effect on the yield and selectivity of the model reaction in the present study (Table 5). However, triethylammonium cation [Et3NH]+ in each respective series i.e., [Et3NH][HSO4], [Et3NH][H2PO4] and [Et3NH][CH3COO] enhances the yield of the desired product 4a to a reasonable extent. Tuning the ionic liquid in a particular series in the present study, by replacing ethyl group's by methyl group's results in a modest decrease in the yield of the desired product 4a. This fluctuation in yield can be presumably attributed to the extent of stabilization of ammonium cation by the attached electron releasing alkyl groups (+Inductive effect), the more stabilization effect being offered by ethyl moiety followed by methyl group. It is obvious that tuning of triethylammonium cation by HSO4 anion in the present study provides excellent yields (92–98%) of the desired products. These results imply that [Et3NH][HSO4] is the best ionic liquid catalyst/promoter for the synthesis of present thiazolidine/oxazolidine derivatives.
Table 5 Comparison of the efficiency of [Et3NH][HSO4] for the synthesis of (4a)a
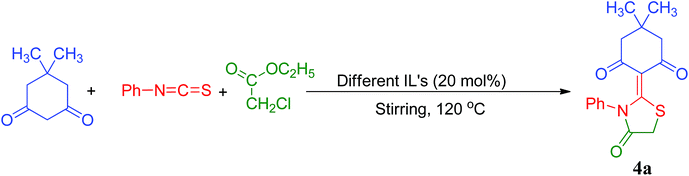
|
Entry |
Catalyst |
Timeb (min) |
Yieldc (%) |
Reaction conditions: 5,5-dimethylcyclohexane-1,3-dione (1a, 2 mmol), phenylisothiocyanate (2a, 2 mmol) and ethylchloroacetate (3, 2 mmol), solvent free, 120 °C, different ionic liquids (20 mol%). Reaction progress monitored by TLC. Isolated yield of products. |
1 |
[Et3NH][HSO4] |
25 |
98 |
2 |
[Me3NH][HSO4] |
48 |
89 |
3 |
[Et2NH2][HSO4] |
43 |
93 |
4 |
[Et3NH][H2PO4] |
51 |
85 |
5 |
[Me3NH][H2PO4] |
55 |
81 |
6 |
[Et2NH2][H2PO4] |
49 |
76 |
7 |
[Et3NH][CH3COO] |
54 |
78 |
8 |
[Me3NH][CH3COO] |
59 |
74 |
The reusability of the catalyst was also explored for the selected model reaction. The catalyst was reused five times and the results demonstrate that the catalyst can be reused without a significant reduction in the yield (Table 6). After the first fresh run with 98% yield, cold water was added to the reaction mixture and the products were isolated by filtration. The ionic liquid was recovered from the filtrate by removing the water under reduced pressure. The recovered ionic liquid was further tested up to four more reaction cycles. Recycling and reuse of the ionic liquid showed minimal decreases in yields. The product 4a was obtained in 92%, 89%, 85%, 80% yields after successive cycles. (Table 6, entries 2–5), thus proving the catalyst's reusability. The results demonstrate that our ionic liquid is almost stable towards moisture. Although the reaction rate get decreased gradually with repetition of the reaction cycle, however we succeeded in getting the desired product 4a in satisfactory yield (80%) even after 5th repetition of the model reaction without any addition of the fresh catalyst (Table 6, entry 5). This gradual but consistent dip in reaction rate is assumed to be due to remains of traces of water in ionic liquid during reaction work-up process. The traces of water in the ionic liquid is believed to react with active reaction reactants (phenylisothiocyanate) leading to by-product formation, consequently lowering the yields of desired product 4a in the subsequent cycles.86
Table 6 Reusability of [Et3NH][HSO4] in the synthesis of (4a)a
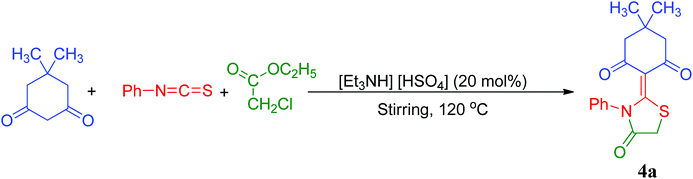
|
Entry |
Reaction cycle |
Isolated yieldb (%) |
Reaction conditions: 5,5-dimethylcyclohexane-1,3-dione (1a, 2 mmol), phenylisothiocyanate (2a, 2 mmol) and ethylchloroacetate (3, 2 mmol), [Et3NH][HSO4] (20 mol%), 120 °C. Isolated yield of products. |
1 |
1st (fresh run) |
98 |
2 |
2nd cycle |
92 |
3 |
3rd cycle |
89 |
4 |
4th cycle |
85 |
5 |
5th cycle |
80 |
To ascertain the intermediate involved in the reaction pathway, the reaction of 5,5-dimethyl-cyclohexane-1,3-dione (1a) and phenylisothiocyanate (2a) was ceased without the addition of ethylchloroacetate (3) (Scheme 2). Water was added and the reaction mixture was further stirred for 5 min. The solid obtained was removed by filtration, washed with appropriate solvents and then crystallized from methanol to yield block shaped crystals of intermediate compound 5 (Fig. S3†). The structural authentication of intermediate compound 5 was confirmed on the basis of 1H and 13C NMR spectral analysis (Fig. S4 and S5†), finally verified by single crystal X-ray crystallographic studies (Fig. 3). Pertinent crystallographic data for compound 5 is summarized in Table S1 (see ESI†).
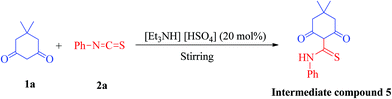 |
| Scheme 2 Synthetic route for the synthesis of intermediate compound 5. | |
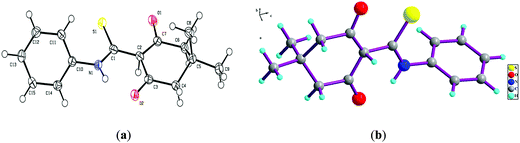 |
| Fig. 3 Asymmetric unit showing (a) thermal ellipsoid (50%) plot and (b) ball and stick model of intermediate compound (5). | |
In light of the above results, a plausible mechanistic pathway (Scheme 3) is proposed to illustrate the synthesis of thiazolidine/oxazolidine derivatives catalyzed by [Et3NH][HSO4]. The initial step is believed to be the protonation of the cyanates (I) by protic ionic liquid [Et3NH][HSO4] to form intermediate (II), which facilitates the nucleophilic attack of substituted 1,3-diketone to promote the formation of C–C bond to yield intermediate (III). The subsequent elimination of HCl by the reaction of intermediate (III) with ethylchloroacetate expedite the formation of C–X bond to yield compound (IV). The final step involves the sequential expulsion of ethanol (EtOH) molecule by the nucleophilic attack of nitrogen to the carbonyl group to promote C–N bond formation, accelerated by ionic liquid [Et3NH][HSO4] eventually leads to the formation of cyclized target products 4(a–u).
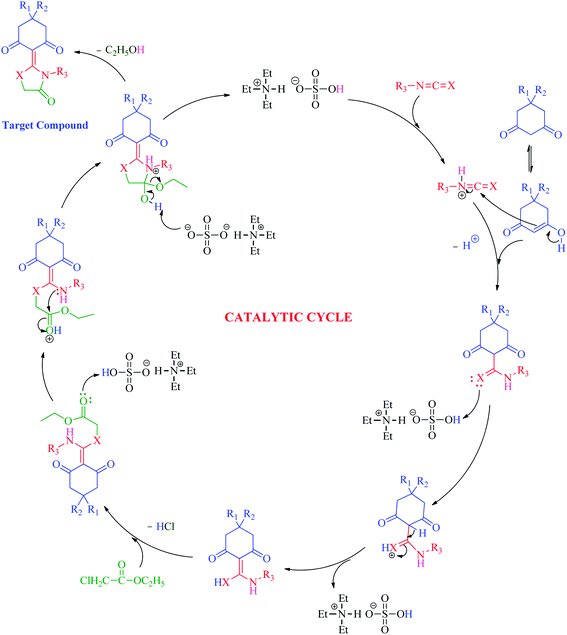 |
| Scheme 3 Plausible mechanistic catalytic cycle for the synthesis of target thiazolidine/oxazolidine derivatives 4(a–u). | |
Experimental section
Materials and general methods
Chemicals and reagents were purchased from Merck and Sigma-Aldrich (India) as ‘synthesis grade’ and used without further purification. Melting points were determined on a Kofler apparatus and are uncorrected. Elemental analysis (C, H, N) was conducted using Carlo Erba analyzer model 1108. The IR spectra were recorded with a Shimadzu IR-408 Perkin-Elmer1800 instrument (FTIR) and the values are given in cm−1. 1H NMR and 13C NMR spectra were run in DMSO-d6 on a Bruker Avance-II 400 MHz instrument with TMS as an internal standard and J values were measured in Hertz (Hz). Chemical shifts are reported in ppm (δ) relative to TMS. Mass spectra were recorded on a JEOL D-300 mass spectrometer. Thin layer chromatography (TLC) glass plates (20 × 5 cm) were coated with silica gel G (Merck) and exposed to iodine vapors to check the homogeneity as well as the progress of the reaction.
Synthesis of ionic liquids
The simple ammonium ionic liquids of general type [amine][HSO4] were synthesized by the known standard literature methods87 in the following way.
Triethylammonium sulfate [Et3NH][HSO4]. The synthesis of ionic liquid was carried out in a 250 mL round-bottomed flask, which was immersed in a recirculating heated water-bath and fitted with a reflux condenser. Sulfuric acid (49 g, 0.5 mol) 98% solution in water was added drop wise into triethylamine (50.5 g, 0.5 mol) at 60 °C for 1 hour. After the addition, the reaction mixture was stirred for an additional period of 1 hour at 70 °C to ensure the reaction had proceeded to completion. The traces of water were removed by heating the residue at 80 °C in high vacuum (5 mm Hg) until the weight of the residue remained constant. The yield of [Et3NH][HSO4] was 95%. 1H NMR (400 MHz, DMSO-d6, δ, ppm): 1.29 (t, 9H), 3.13 (m, 6H), 8.85 (s, 1H, D2O exchangeable). 13C NMR (100 MHz, DMSO-d6, δ, ppm): 10.27 (CH3), 52.09 (CH2).The following ionic liquids were synthesized by the same procedure.87
Trimethylammonium sulfate [Me3NH][HSO4]. 1H NMR (400 MHz, DMSO-d6, δ, ppm): 2.52 (s, 9H), 2.70 (s, 1H, D2O exchangeable).
Diethylammonium sulfate [Et2NH2][HSO4]. 1H NMR (400 MHz, DMSO-d6, δ, ppm): 1.13 (t, 6H), 2.97 (m, 4H), 8.24 (s, 2H, D2O exchangeable).
Triethylammonium dihydrogen phosphate [Et3NH][H2PO4]. 1H NMR (400 MHz, DMSO-d6, δ, ppm): 1.16 (t, 9H), 3.23 (m, 6H), 8.73 (s, 1H, D2O exchangeable).
Trimethylammonium dihydrogen phosphate [Me3NH][H2PO4]. 1H NMR (400 MHz, DMSO-d6, δ, ppm): 2.51 (s, 9H), 2.73 (s, 1H, D2O exchangeable).
Diethylammonium dihydrogen phosphate [Et2NH2][H2PO4]. 1H NMR (400 MHz, DMSO-d6, δ, ppm): 1.15 (t, 6H), 2.94 (m, 3H), 8.11 (s, 2H, D2O exchangeable).
Triethylammonium acetate [Et3NH][CH3COO]. 1H NMR (400 MHz, DMSO-d6, δ, ppm): 1.12 (t, 9H), 2.10 (s, 3H), 3.14 (m, 6H), 8.81 (s, 1H, D2O exchangeable).
Trimethylammonium acetate [Me3NH][CH3COO]. 1H NMR (400 MHz, DMSO-d6, δ, ppm): 2.57 (s, 9H), 2.15 (s, 3H), 2.73 (s, 1H, D2O exchangeable).
General procedure for the synthesis of thiazolidine/oxazolidine derivatives
To a mixture of substituted 1,3-diketone 1(a–g) (2 mmol) and cyanate 2(a–c) (2 mmol), 20 mol% of [Et3NH][HSO4] was added and the reaction mixture was allowed to stir at room temperature for 5 min. After 5 min, ethylchloroacetate (2 mmol) was added and the reaction mixture was heated at 120 °C with stirring. During the reaction process, the reaction mixture spontaneously solidified. After completion of the reaction as evident from thin layer chromatography (TLC), the reaction mixture was allowed to cool at room temperature. Water was added and the reaction mixture was further stirred for 5 min. The solid obtained was removed by filtration, washed with appropriate solvents and then recrystallized from ethanol. The water was removed from filtrate under reduced pressure to recover [Et3NH][HSO4], which was then reused in subsequent cycles.
Spectral characterization
5,5-Dimethyl-2-(4-oxo-3-phenyl-thiazolidin-2-yl)-cyclohexane-1,3-dione (4a). Compound 4a crystallized from CHCl3–MeOH as colorless solid; yield: 98%; m.p. 128–129 °C; anal. calc. for C17H17NO3S: C, 64.74; H, 5.43; N, 4.44; found: C, 64.70; H, 5.41; N, 4.39%. IR νKBrmax cm−1: 1744 (C
O), 1640 (–CON), 1613 (C
C), 2874 (CH2 str.). 1H NMR (400 MHz, DMSO-d6, δ, ppm): 1.12 (s, 6H, 2 × CH3), 1.57 (s, 2H, CH2), 2.48 (s, 2H, CH2), 2.65 (s, 2H, CH2, thiazolidine ring), 7.26–7.47 (m, 5H, phenyl ring). 13C NMR (100 MHz, DMSO-d6, δ, ppm): 27.9 (2 × CH3), 30.22 (C-5), 46.9 (C-5′), 52.5 (C-4 and C-6), 108 (C-2), 125.7, 127.3, 128.9, 131.5 (phenyl ring), 137.0 (C-2′), 180.0 (C
O, C-4′), 191.5 (C
O), 199.3 (C
O). MS (ESI) m/z: 315 [M + H]+˙.
5,5-Dimethyl-2-(4-oxo-3-phenyl-oxazolidin-2-yl)-cyclohexane-1,3-dione (4b). Compound 4b crystallized from CHCl3–EtOH as white solid; yield: 95%; m.p 125–126 °C; anal. calc. for C17H17NO4: C, 68.21; H, 5.72; N, 4.68; found: C, 68.24; H, 5.70; N, 4.63%. IR νKBrmax cm−1: 2945 (CH2 str), 1744 (C
O), 1649 (–CON), 1597 (C
C). 1H NMR (400 MHz, DMSO-d6, δ, ppm): 1.12 (s, 6H, 2 × CH3), 1.57 (s, 2H, CH2), 2.48 (s, 2H, CH2), 2.65 (s, 2H, CH2, oxazolidine ring), 7.0–7.38 (m, 5H, phenyl ring). 13C NMR (100 MHz, DMSO-d6, δ, ppm): 22.2 (2 × CH3), 29.9 (C-5), 36.4 (C-5′), 48.3 (C-4 and C-6), 108 (C-2), 119.8, 121.9, 128.8, 131.5 (phenyl ring), 139.1 (C-2′), 155.7 (C
O, C-4′), 192.2 (C
O), 195.5 (C
O). MS (ESI) m/z: 299 [M + H]+˙.
2-(3-Allyl-4-oxo-thiazolidin-2-yl)-5,5-dimethyl-cyclohexane-1,3-dione (4c). Compound 4c crystallized from CHCl3–MeOH as brownish solid; yield: 96%; m.p. 145–146 °C. anal. calc. for C14H17NO3S: C, 60.19; H, 6.13; N, 5.01; found: C, 60.15; H, 6.15; N, 5.04%. IR νKBrmax cm−1: 1739 (C
O), 1645 (–CON), 1592 (C
C), 29
631 (CH2 str). 1H NMR (400 MHz, DMSO-d6, δ, ppm): 1.07 (s, 6H, 2 × CH3), 1.59 (s, 2H, CH2), 2.57 (s, 2H, CH2), 2.70 (s, 2H, CH2, thiazolidine ring), 2.98 (d, 2H, N–CH2), 4.53 (dd, 2H,
CH2), 6.03 (m, 1H,
CH–). 13C NMR (100 MHz, DMSO-d6, δ, ppm): 26.5 (2 × CH3), 31.6 (C-5), 46.9 (N–CH2), 52.5 (C-4 and C-6), 105.2 (C-2), 116.4 (
CH2), 137 (C-2′), 139.2 (
CH–), 172.3 (C
O, C-4′), 189.5 (C
O), 190.1 (C
O). MS (ESI) m/z: 279 [M + H]+˙.
5-(4-Chlorophenyl)-2-(4-oxo-3-phenylthiazolidin-2-ylidene)-cyclohexane-1,3-dione (4d). Compound 4d crystallized from CHCl3–MeOH as colorless solid; yield: 94%; m.p. 198 °C; anal. calc. for C21H16ClNO3S: C, 63.39; H, 4.05; N, 3.52; found: C, 63.37; H, 4.06; N, 3.53%. IR νKBrmax cm−1: 1746 (C
O), 1630 (–CON), 1611 (C
C), 2870 (CH2 str.). 1H NMR (400 MHz, DMSO-d6, δ, ppm): 3.20 (m, 1H, C-5), 3.38 (m, 4H, C-4 and C-6), 4.20 (s, 2H, C-5′ thiazolidine ring), 7.10–7.45 (m, 9H, phenyl ring). 13C NMR (100 MHz, DMSO-d6, δ, ppm): 31.9 (C-5), 33.9 (C-2′), 43.9 (C-5′), 46.8 (C-4 and C-6), 106.3 (C-2), 126.5 (C-2′′ and C-6′′), 129.5 (C-3′′ and C-5′′), 132.1 (C-4′′), 133.5, 127.8, 124.9, 118.8 (phenyl ring), 140.5 (C-1′′), 166.6 (C-4′), 199.0 (C-1 and C-3). MS (ESI) m/z: 397.05 [M + H]+˙.
5-(4-Chlorophenyl)-2-(4-oxo-3-phenyloxazolidin-2-ylidene)-cyclohexane-1,3-dione (4e). Compound 4e crystallized from CHCl3-acetone as orange solid; yield: 97%; m.p. 175 °C; anal. calc. for C21H16ClNO4: C, 66.06; H, 4.22; N, 3.67; found: C, 66.01; H, 4.25; N, 3.69%. IR νKBrmax cm−1: 1736 (C
O), 1635 (–CON), 1619 (C
C), 2878 (CH2 str.). 1H NMR (400 MHz, DMSO-d6, δ, ppm): 3.24 (m, 1H, C-5), 3.31 (m, 4H, C-4 and C-6), 4.82 (s, 2H, C-5′ oxazolidine ring), 7.22–7.40 (m, 9H, phenyl ring). 13C NMR (100 MHz, DMSO-d6, δ, ppm): 33.5 (C-5), 46.82 (C-4 and C-6), 48.9 (C-2′), 73.5 (C-5′), 106.7 (C-2), 126.2 (C-2′′ and C-6′′), 128.8 (C-3′′ and C-5′′), 131.5 (C-4′′), 133.1, 126.8, 125.9, 117.8 (phenyl ring), 140.7 (C-1′′), 165.6 (C-4′), 197.8 (C-1 and C-3). MS (ESI) m/z: 381.08 [M + H]+˙.
2-(3-Allyl-4-oxothiazolidin-2-ylidene)-5-(4-chlorophenyl)-cyclohexane-1,3-dione (4f). Compound 4f crystallized from CHCl3–MeOH as white solid; yield: 92%; m.p. 182 °C; anal. calc. for C18H16ClNO3S: C, 59.75; H, 4.46; N, 3.87; found: C, 59.72; H, 4.47; N, 3.89%. IR νKBrmax cm−1: 1746 (C
O), 1639 (–CON), 1616 (C
C), 2870 (CH2 str.). 1H NMR (400 MHz, DMSO-d6, δ, ppm): 3.22 (m, 1H, C-5), 3.35 (m, 4H, C-4 and C-6), 3.98 (d, 2H, N–CH2), 4.13 (dd, 2H,
CH2), 4.89 (s, 2H, C-5′ thiazolidine ring), 6.13 (m, 1H,
CH–), 7.27 (d, 2H, C-2′′ and C-6′′), 7.36 (d, 2H, C-3′′ and C-5′′). 13C NMR (100 MHz, DMSO-d6, δ, ppm): 14.5 (C-4′′), 31.9 (C-5), 33.8 (C-5′), 45.5 (C-2′), 46.8 (C-4 and C-6), 46.9 (N–CH2), 106.1 (C-2), 116.4 (
CH2), 126.9 (C-2′′ and C-6′′), 127.8 (C-3′′ and C-5′′), 139.2 (
CH–), 141.7 (C-1′′), 166.6 (C-4′), 197.6 (C-1 and C-3). MS (ESI) m/z: 361.05 [M + H]+˙.
5-(3,4-Dimethoxyphenyl)-2-(4-oxo-3-phenylthiazolidin-2-ylidene)-cyclohexane-1,3-dione (4g). Compound 4g crystallized from CHCl3–EtOH as reddish solid; yield: 93%; m.p. 201 °C; anal. calc. for C23H21NO5S: C, 65.23; H, 5.00; N, 3.3; found: C, 65.20; H, 5.03; N, 3.3%. IR νKBrmax cm−1: 1732 (C
O), 1639 (–CON), 1620 (C
C), 2888 (CH2 str.). 1H NMR (400 MHz, DMSO-d6, δ, ppm): 3.11 (m, 4H, C-4 and C-6), 3.20 (m, 1H, C-5), 3.53 (s, 6H, 2 × –OCH3), 4.72 (s, 2H, C-5′ thiazolidine ring), 7.12–7.70 (m, 8H, phenyl ring). 13C NMR (100 MHz, DMSO-d6, δ, ppm): 31.9 (C-5), 33.5 (C-5′), 46.62 (C-4 and C-6), 57.0 (2 × –OCH3), 106.9 (C-2), 112.0 (C-6′′), 113.8 (C-3′′), 121.2 (C-2′′), 133.8, 126.2, 124.9, 118.8 (phenyl ring), 143.5 (C-4′′), 145.7 (C-1′′), 149.6 (C-5′′), 165.7 (C-2′), 178.9 (C-4′), 196.8 (C-1 and C-3). MS (ESI) m/z: 423.11 [M + H]+˙.
5-(3,4-Dimethoxyphenyl)-2-(4-oxo-3-phenyloxazolidin-2-ylidene)-cyclohexane-1,3-dione (4h). Compound 4h crystallized from CHCl3–acetone as white solid; yield: 96%; m.p. 215 °C; anal. calc. for C23H21NO6: C, 67.80; H, 5.20; N, 3.44; found: C, 67.80; H, 5.22; N, 3.42%. IR νKBrmax cm−1: 1733 (C
O), 1632 (–CON), 1614 (C
C), 2858 (CH2 str.). 1H NMR (400 MHz, DMSO-d6, δ, ppm): 3.10 (m, 1H, C-5), 3.21 (m, 4H, C-4 and C-6), 3.69 (s, 6H, 2 × –OCH3), 4.79 (s, 2H, C-5′ oxazolidine ring), 7.32–7.72 (m, 8H, phenyl ring). 13C NMR (100 MHz, DMSO-d6, δ, ppm): 31.54 (C-5), 46.35 (C-4 and C-6), 56.2 (2 × –OCH3), 73.5 (C-5′), 104.9 (C-2), 112.1 (C-3′′), 112.5 (C-6′′), 120.6 (C-2′′), 132.9, 126.8, 124.1, 118.5 (phenyl ring), 143.0 (C-4′′), 147.1 (C-1′′), 147.9 (C-2′), 148.2 (C-5′′), 165.1 (C-4′), 195.1 (C-1 and C-3). MS (ESI) m/z: 407.14 [M + H]+˙.
2-(3-Allyl-4-oxothiazolidin-2-ylidene)-5-(3,4-dimethoxyphenyl)-cyclohexane-1,3-dione (4i). Compound 4i crystallized from CHCl3–MeOH as yellowish solid; yield: 94%; m.p. 173 °C; anal. calc. for C20H21NO5S: C, 62.00; H, 5.46; N, 3.62; found: C, 62.04; H, 5.42; N, 3.60%. IR νKBrmax cm−1: 1738 (C
O), 1622 (–CON), 1620 (C
C), 2852 (CH2 str.). 1H NMR (400 MHz, DMSO-d6, δ, ppm): 3.14 (m, 1H, C-5), 3.26 (m, 4H, C-4 and C-6), 3.79 (s, 6H, 2 × –OCH3), 4.21 (s, 2H, C-5′ thiazolidine ring), 4.93 (dd, 2H,
CH2), 4.98 (d, 2H, N–CH2), 6.03 (m, 1H,
CH–), 6.13 (d, 1H, C-2′′), 7.17 (s, 1H, C-6′′), 7.37 (d, 1H, C-3′′). 13C NMR (100 MHz, DMSO-d6, δ, ppm): 31.59 (C-5), 32.6 (C-5′), 45.1 (N–CH2), 46.31 (C-4 and C-6), 56.4 (2 × –OCH3), 108.9 (C-2), 112.4 (C-3′′), 112.8 (C-6′′), 116.8 (
CH2), 120.9 (C-2′′), 135.2 (
CH–), 143.6 (C-4′′), 146.7 (C-2′), 147.8 (C-1′′), 148.7 (C-5′′), 165.6 (C-4′), 193.9 (C-1 and C-3). MS (ESI) m/z: 387.11 [M + H]+˙.
5-(4-(Dimethylamino)phenyl)-2-(4-oxo-3-phenylthiazolidin-2-ylidene)-cyclohexane-1,3-dione (4j). Compound 4j crystallized from CHCl3–EtOH as colorless solid; yield: 92%; m.p. 156 °C; anal. calc. for C23H22N2O3S: C, 67.96; H, 5.46; N, 6.89; found: C, 67.93; H, 5.47; N, 6.91%. IR νKBrmax cm−1: 1739 (C
O), 1634 (–CON), 1627 (C
C), 2878 (CH2 str.). 1H NMR (400 MHz, DMSO-d6, δ, ppm): 3.11 (m, 4H, C-4 and C-6), 3.19 (s, 6H, 2 × CH3), 3.25 (m, 1H, C-5), 4.22 (s, 2H, C-5′ thiazolidine ring), 7.05–7.50 (m, 9H, phenyl ring). 13C NMR (100 MHz, DMSO-d6, δ, ppm): 31.9 (C-5), 33.9 (C-5′), 42.6 (2 × –CH3), 46.6 (C-4 and C-6), 106.3 (C-2), 108.9 (C-2′), 116.1 (C-3′′ and C-5′′), 128.9 (C-2′′ and C-6′′), 133.1, 126.5, 124.7, 119.7 (phenyl ring), 139.5 (C-1′′), 147.1 (C-4′′), 165.7 (C-4′), 196.5 (C-1 and C-3). MS (ESI) m/z: 406.14 [M + H]+˙.
5-(4-(Dimethylamino)phenyl)-2-(4-oxo-3-phenyloxazolidin-2-ylidene)-cyclohexane-1,3-dione (4k). Compound 4k crystallized from CHCl3–methanol bluish solid; yield: 98%; m.p. 167 °C; anal. calc. for C23H22N2O4: C, 70.75; H, 5.68; N, 7.17; found: C, 70.74; H, 5.66; N, 7.20%. IR νKBrmax cm−1: 1737 (C
O), 1636 (–CON), 1625 (C
C), 2875 (CH2 str.). 1H NMR (400 MHz, DMSO-d6, δ, ppm): 3.16 (m, 4H, C-4 and C-6), 3.19 (m, 1H, C-5), 3.20 (s, 6H, 2 × CH3), 4.86 (s, 2H, C-5′ oxazolidine ring), 7.28–7.48 (m, 9H, phenyl ring). 13C NMR (100 MHz, DMSO-d6, δ, ppm): 31.9 (C-5), 37.3 (C-5′), 42.8 (2 × –CH3), 46.67 (C-4 and C-6), 106.4 (C-2), 108.6 (C-2′), 139.5, 126.9, 118.1, 147.1, 133.1, 126.5, 124.7, 118.2 (phenyl ring), 165.9 (C-4′), 196.2 (C-1 and C-3). MS (ESI) m/z: 390.16 [M + H]+˙.
2-(3-Allyl-4-oxothiazolidin-2-ylidene)-5-(4-(dimethylamino)phenyl)-cyclohexane-1,3-dione (4l). Compound 4l crystallized from CHCl3–acetone as white solid; yield: 95%; m.p. 163 °C; anal. calc. for C20H22N2O3S; C, 64.84; H, 5.99; N, 7.56; found: C, 64.82; H, 5.99; N, 7.58%. IR νKBrmax cm−1: 1734 (C
O), 1627 (–CON), 1625 (C
C), 2858 (CH2 str.). 1H NMR (400 MHz, DMSO-d6, δ, ppm): 3.09 (s, 6H, 2 × –CH3), 3.16 (m, 4H, C-4 and C-6), 3.17 (m, 1H, C-5), 4.19 (s, 2H, C-5′ thiazolidine ring), 4.95 (d, 2H, N–CH2), 5.03 (dd, 2H,
CH2), 6.00 (m, 1H,
CH–), 6.12 (dd, 2H, C-2′′ and C-6′′), 7.34 (dd, 1H, C-3′′ and C-5′′). 13C NMR (100 MHz, DMSO-d6, δ, ppm): 32.5 (C-5), 32.6 (C-5′), 42.5 (2 × –CH3), 45.4 (N–CH2), 46.31 (C-4 and C-6), 108.2 (C-2), 112.7 (C-3′′ and C-5′′), 116.7 (C-2′), 117.7 (
CH2), 128.9 (C-2′′ and C-6′′), 132.8 (C-1′′), 134.2 (
CH–), 145.6 (C-4′′), 165.6 (C-4′), 193.6 (C-1 and C-3). MS (ESI) m/z: 370.14 [M + H]+˙.
5-(Benzo[d][1,3]dioxol-5-yl)-2-(4-oxo-3-phenylthiazolidin-2-ylidene)-cyclohexane-1,3-dione (4m). Compound 4m crystallized from CHCl3–MeOH as light yellowish solid; yield: 96%; m.p. 204 °C; anal. calc. for C22H17NO5S: C, 64.85; H, 4.21; N, 3.44; found: C, 64.88; H, 4.20; N, 3.42%. IR νKBrmax cm−1: 1730 (C
O), 1640 (–CON), 1632 (C
C), 2872 (CH2 str.). 1H NMR (400 MHz, DMSO-d6, δ, ppm): 3.16 (m, 4H, C-4 and C-6), 3.27 (m, 1H, C-5), 4.17 (s, 2H, C-5′ thiazolidine ring), 6.12 (s, 2H, –CH2), 7.23–7.47 (m, 8H, phenyl ring). 13C NMR (100 MHz, DMSO-d6, δ, ppm): 31.9 (C-5), 33.5 (C-5′), 46.6 (C-4 and C-6), 102.0 (–CH2), 105.9 (C-2), 109.8 (C-3′′), 112.2 (C-6′′), 120.2 (C-2′′), 133.1, 126.8, 124.3, 118.3 (phenyl ring), 143.2 (C-1′), 145.5 (C-4′′), 149.6 (C-5′′), 166.7 (C-2′), 168.9 (C-4′), 196.8 (C-1 and C-3). MS (ESI) m/z: 407.08 [M + H]+˙.
5-(Benzo[d][1,3]dioxol-5-yl)-2-(4-oxo-3-phenyloxazolidin-2-ylidene)-cyclohexane-1,3-dione (4n). Compound 4n crystallized from CHCl3–benzene as colorless solid; yield: 93%; m.p. 219 °C; anal. calc. for C22H17NO6: C, 67.51; H, 4.38; N, 3.58; found: C, 67.50; H, 4.36; N, 3.61%. IR νKBrmax cm−1: 1732 (C
O), 1647 (–CON), 1636 (C
C), 2879 (CH2 str.). 1H NMR (400 MHz, DMSO-d6, δ, ppm): 3.17 (m, 4H, C-4 and C-6), 3.29 (m, 1H, C-5), 4.93 (s, 2H, C-5′ oxazolidine ring), 6.16 (s, 2H, –CH2), 7.21–7.48 (m, 8H, phenyl ring). 13C NMR (100 MHz, DMSO-d6, δ, ppm): 32.9 (C-5), 46.6 (C-4 and C-6), 72.5 (C-5′), 102.9 (–CH2), 105.9 (C-2), 109.6 (C-3′′), 112.3 (C-6′′), 121.2 (C-2′′), 133.9, 126.5, 124.6, 118.2 (phenyl ring), 144.1 (C-1′′), 145.8 (C-4′′), 149.7 (C-5′′), 154.9 (C-2′), 165.7 (C-4′), 194.3 (C-1 and C-3). MS (ESI) m/z: 391.11 [M + H]+˙.
2-(3-Allyl-4-oxothiazolidin-2-ylidene)-5-(benzo[d][1,3]dioxol-5-yl)-cyclohexane-1,3-dione (4o). Compound 4o crystallized from CHCl3–acetone as orange solid; yield: 97%; m.p. 196 °C; anal. calc. for C19H17NO5S: C, 61.44; H, 4.61; N, 3.77; found: C, 61.45; H, 4.60; N, 3.77%. IR νKBrmax cm−1: 1736 (C
O), 1632 (–CON), 1617 (C
C), 2854 (CH2 str.). 1H NMR (400 MHz, DMSO-d6, δ, ppm): 3.14 (m, 4H, C-4 and C-6), 3.21 (m, 1H, C-5), 4.19 (s, 2H, C-5′ thiazolidine ring), 4.93 (d, 2H, N–CH2), 5.00 (dd, 2H,
CH2), 6.03 (m, 1H,
CH–), 6.12 (s, 2H, –CH2), 7.25–7.45 (m, 3H, phenyl ring). 13C NMR (100 MHz, DMSO-d6, δ, ppm): 32.7 (C-5′), 33.9 (C-5), 45.9 (N–CH2), 46.6 (C-4 and C-6), 95.6 (O–CH2–O), 105.9 (C-2), 109.6 (C-3′′), 112.9 (C-6′′), 117.1 (
CH2), 120.6 (C-2′′), 133.2 (
CH–), 144.8 (C-1′′), 145.5 (C-4′′), 149.8 (C-5′′), 154.9 (C-2′), 167.4 (C-4′), 196.2 (C-1 and C-3). MS (ESI) m/z: 371.08 [M + H]+˙.
5-(Furan-2-yl)-2-(4-oxo-3-phenylthiazolidin-2-ylidene)-cyclohexane-1,3-dione (4p). Compound 4p crystallized from CHCl3–MeOH as creamy solid; yield: 93%; m.p. 137 °C; anal. calc. for C19H15NO4S: C, 64.58; H, 4.28; N, 3.96; found: C, 64.55; H, 4.27; N, 3.98%. IR νKBrmax cm−1: 1749 (C
O), 1637 (–CON), 1618 (C
C), 2876 (CH2 str.). 1H NMR (400 MHz, DMSO-d6, δ, ppm): 3.37 (m, 4H, C-4 and C-6), 3.40 (m, 1H, C-5), 4.18 (s, 2H, C-5′ thiazolidine ring), 6.12 (d, 1H, C-3′′), 6.45 (dd, 1H, C-4′′), 7.24–7.46 (m, 5H, phenyl ring), 7.52 (d, 1H, C-5′′). 13C NMR (100 MHz, DMSO-d6, δ, ppm): 27.1 (C-5), 34.9 (C-5′), 48.8 (C-4 and C-6), 105.3 (C-2), 108.1 (C-3′′), 112.1 (C-4′′), 132.6, 127.1, 124.7, 118.1 (phenyl ring), 154.4 (C-5′′), 156.9 (C-2′′), 166.9 (C-2′), 167.9 (C-4′), 198.0 (C-1 and C-3). MS (ESI) m/z: 353.07 [M + H]+˙.
5-(Furan-2-yl)-2-(4-oxo-3-phenyloxazolidin-2-ylidene)-cyclohexane-1,3-dione (4q). Compound 4q crystallized from CHCl3–MeOH as silver-white solid; yield: 95%; m.p. 147 °C; anal. calc. for C19H15NO5: C, 67.65; H, 4.48; N, 4.15; found: C, 67.62; H, 4.49; N, 4.17%. IR νKBrmax cm−1: 1743 (C
O), 1632 (–CON), 1619 (C
C), 2878 (CH2 str.). 1H NMR (400 MHz, DMSO-d6, δ, ppm): 3.35 (m, 4H, C-4 and C-6), 3.48 (m, 1H, C-5), 4.08 (s, 2H, C-5′ oxazolidine ring), 6.16 (d, 1H, C-3′′), 6.49 (dd, 1H, C-4′′), 7.23–7.47 (m, 5H, phenyl ring), 7.50 (d, 1H, C-5′′). 13C NMR (100 MHz, DMSO-d6, δ, ppm): 25.1 (C-5), 48.3 (C-4 and C-6), 74.9 (C-5′), 90.3 (C-2), 108.7 (C-3′′), 111.1 (C-4′′), 132.1, 127.5, 124.9, 118.4 (phenyl ring), 150.4 (C-5′′), 156.9 (C-2′′), 161.2 (C-2′), 178.9 (C-4′), 198.3 (C-1 and C-3). MS (ESI) m/z: 337.10 [M + H]+˙.
2-(3-Allyl-4-oxothiazolidin-2-ylidene)-5-(furan-2-yl)-cyclohexane-1,3-dione (4r). Compound 4r crystallized from CHCl3–acetone as white solid; yield: 97%; m.p. 153 °C; anal. calc. for C16H15NO4S: C, 60.55; H, 4.76; N, 4.41; found: C, 60.53; H, 4.79; N, 4.40%. IR νKBrmax cm−1: 1743 (C
O), 1634 (–CON), 1619 (C
C), 2877 (CH2 str.). 1H NMR (400 MHz, DMSO-d6, δ, ppm): 3.32 (m, 4H, C-4 and C-6), 3.49 (m, 1H, C-5), 4.15 (s, 2H, C-5′ thiazolidine ring), 4.97 (d, 2H, N–CH2), 5.18 (dd, 2H,
CH2), 6.01 (m, 1H,
CH–), 6.15 (d, 1H, C-3′′), 6.41 (dd, 1H, C-4′′), 7.50 (d, 1H, C-5′′). 13C NMR (100 MHz, DMSO-d6, δ, ppm): 26.1 (C-5), 34.2 (C-5′), 45.0 (N–CH2), 48.8 (C-4 and C-6), 105.3 (C-2), 109.1 (C-3′′), 111.5 (C-4′′), 117.8 (
CH2), 133.9 (
CH–), 142.4 (C-5′′), 147.9 (C-2′), 156.7 (C-2′′), 165.8 (C-4′), 196.2 (C-1 and C-3). MS (ESI) m/z: 317.07.
5-(4-Fluorophenyl)-2-(4-oxo-3-phenylthiazolidin-2-ylidene)-cyclohexane-1,3-dione (4s). Compound 4s crystallized from CHCl3–MeOH as brownish solid; yield: 98%; m.p. 186 °C; anal. calc. for C21H16FNO3S: C, 66.13; H, 4.23; N, 3.67; found: C, 66.10; H, 4.25; N, 3.68%; IR νKBrmax cm−1: 1745 (C
O), 1636 (–CON), 1615 (C
C), 2878 (CH2 str.). 1H NMR (400 MHz, DMSO-d6, δ, ppm): 3.21 (m, 1H, C-5), 3.36 (m, 4H, C-4 and C-6), 4.18 (s, 2H, C-5′ thiazolidine ring), 7.20–7.46 (m, 9H, phenyl ring). 13C NMR (100 MHz, DMSO-d6, δ, ppm): 32.9 (C-5), 43.2 (C-5′), 46.8 (C-4 and C-6), 106.6 (C-2), 128.7 (C-2′′ and C-6′′), 133.1, 127.5, 124.2, 118.7 (phenyl ring), 139.0 (C-1′′), 152.4 (C-3′′ and C-5′′), 161.1 (C-4′′), 166.6 (C-2′), 168.9 (C-4′), 199.6 (C-1 and C-3). MS (ESI) m/z: 381.08 [M + H]+˙.
5-(4-Fluorophenyl)-2-(4-oxo-3-phenyloxazolidin-2-ylidene)-cyclohexane-1,3-dione (4t). Compound 4t crystallized from CHCl3–EtOH as colorless solid; yield: 93%; m.p. 171 °C; anal. calc. for C21H16FNO4: C, 69.04; H, 4.41; N, 3.83; found: C, 69.03; H, 4.42; N, 3.80%; IR νKBrmax cm−1: 1742 (C
O), 1633 (–CON), 1617 (C
C), 2880 (CH2 str.). 1H NMR (400 MHz, DMSO-d6, δ, ppm): 3.26 (m, 1H, C-5), 3.38 (m, 4H, C-4 and C-6), 4.82 (s, 2H, C-5′ oxazolidine ring), 7.23–7.49 (m, 9H, phenyl ring). 13C NMR (100 MHz, DMSO-d6, δ, ppm): 32.5 (C-5), 46.5 (C-4 and C-6), 73.2 (C-5′), 90.5 (C-2), 128.8 (C-2′′ and C-6′′), 133.2, 127.6, 124.8, 118.1 (phenyl ring), 139.7 (C-1′′), 152.1 (C-3′′ and C-5′′), 161.1 (C-4′′), 156.8 (C-2′), 170.9 (C-4′), 198.5 (C-1 and C-3). MS (ESI) m/z: 365.11 [M + H]+˙.
2-(3-Allyl-4-oxothiazolidin-2-ylidene)-5-(4-fluorophenyl)-cyclohexane-1,3-dione (4u). Compound 4u crystallized from CHCl3–MeOH as reddish solid; yield: 95%; m.p. 167 °C; anal. calc. for C18H16FNO3S; C, 62.59; H, 4.67; N, 4.06; found: C, 62.56; H, 4.69; N, 4.07%. IR νKBrmax cm−1: 1749 (C
O), 1638 (–CON), 1619 (C
C), 2882 (CH2 str.). 1H NMR (400 MHz, DMSO-d6, δ, ppm): 3.26 (m, 1H, C-5), 3.37 (m, 4H, C-4 and C-6), 4.19 (s, 2H, C-5′ thiazolidin ring), 4.95 (d, 2H, N–CH2), 5.17 (dd, 2H,
CH2), 6.09 (m, 1H,
CH–), 7.27–7.49 (m, 4H, phenyl ring). 13C NMR (100 MHz, DMSO-d6, δ, ppm): 32.3 (C-5), 43.7 (C-5′), 45.7 (N–CH2), 46.2 (C-4 and C-6), 106.9 (C-2), 117.1 (
CH2), 128.5 (C-2′′ and C-6′′), 133.4, 127.7, 124.9, 118.7 (phenyl ring), 133.5 (
CH–), 139.8 (C-1′′), 152.6 (C-3′′ and C-5′′), 158.4 (C-2′), 161.4 (C-4′′), 168.5 (C-4′), 198.2 (C-1 and C-3). MS (ESI) m/z: 345.08 [M + H]+˙.
4,4-Dimethyl-2,6-dioxo-N-phenylcyclohexanecarbothioamide (5). It was crystallized from CHCl3–acetone as colorless block shaped crystals; yield: 98%; m.p 120 °C [lit.88]; anal. calc. for C15H17NO2S: C, 65.43; H, 6.22; N, 5.09; O, 11.62; found: C, 65.40; H, 6.21; N, 5.03; O, 11.65%. IR νKBrmax cm−1: 2961 (CH2 str.), 1739 (C
O), 1613 (C
C), 1153 (C
S), 1074 (C–N). 1H NMR (400 MHz, DMSO-d6, δ, ppm): 1.12 (s, 6H, 2 × CH3), 2.71 (s, 4H, 2 × CH2), 4.35 (s, 1H), 7.32–7.58 (m, 5H, aromatic), 13.96 (s, 1H, NH, D2O-exchangeable). 13C NMR (100 MHz, DMSO-d6, δ, ppm): 28.2 (2 × CH3), 30.7 (C-4), 56.2 (C-3 and C-5), 86.8 (C-1), 131.2, 128.6, 127, 125.7 (phenyl ring), 164.6 (C
S), 191.5 (C
O), 199.3 (C
O). MS (ESI) m/z: 275.10.
Single crystal X-ray crystallographic studies of intermediate compound (5)
Single crystal X-ray data of intermediate compound (5) was collected at 100 K on a Bruker SMART APEX CCD diffractometer using graphite monochromated MoKα radiation (λ = 0.71073 Å). The linear absorption coefficients, scattering factors for the atoms and the anomalous dispersion corrections were taken from the International Tables for X-ray Crystallography.89 The data integration and reduction were carried out with SAINT90 software. Empirical absorption correction was applied to the collected reflections with SADABS91 and the space group was determined using XPREP.92 The structure was solved by the direct methods using SHELXTL-97 (ref. 93) and refined on F2 by full-matrix least-squares using the SHELXL-97 (ref. 94) program package. All non-hydrogen atoms were refined anisotropically.
Conclusions
The present protocol reports one-pot convenient and eco-friendly approach for the synthesis of thiazolidine/oxazolidine derivatives in excellent yields in ionic liquid [Et3NH][HSO4]. This solvent-free, green synthetic procedure eliminates the use of toxic solvents and thus makes it distinctive one in organic synthesis. The notable feature of this synthetic strategy is that, the ionic liquid possesses both catalytic as well as medium engineering potential in this protocol. The protocol not only offers substantial yield of products and shorter reaction time but also affords high purity, mild reaction conditions, operational simplicity, cleaner reaction profile, enhanced reaction rates and easy workup procedure. The ionic liquid [Et3NH][HSO4] employed in the present study exhibits stability towards water and air and is easy to prepare from cheap amine and acid. The present protocol possesses wide substrate tolerance. We believe that this synthetic approach provides a better scope for the synthesis of thiazolidine/oxazolidine analogues and will be a more practical alternative to the other existing methods.
Acknowledgements
A. M. Malla thanks the Chairman, Department of Chemistry, AMU, Aligarh, for providing the necessary research facilities, University Sophisticated Instrumentation Facility (USIF), AMU, Aligarh and Sophisticated Analytical Instrumentation Facility (SAIF), Chandigarh, is credited for spectral analysis. Indian Institute of Technology (IIT), Kanpur, is thanked for single crystal X-ray analysis. UGC is also gratefully acknowledged for research fellowship to A. M. Malla.
References
- R. Ballini, Eco-Friendly Synthesis of Fine Chemicals, Royal Society of Chemistry, 2009 Search PubMed.
- V. I. Parvulescu and C. Hardacre, Chem. Rev., 2007, 107, 2615 CrossRef CAS PubMed.
- A. Kumar and S. S. Pawar, J. Org. Chem., 2004, 69, 1419 CrossRef CAS PubMed.
- P. Wasserscheid and T. Welton, Ionic Liquids in Synthesis, Wiley-VCH, Weinheim, 2003, p. 41 Search PubMed.
- H. M. Zerth, N. M. Leonard and R. S. Mohan, Org. Lett., 2003, 5, 55 CrossRef CAS PubMed.
- J. Dupont, R. F. de Souza and P. A. Z. Suarez, Chem. Rev., 2002, 102, 3667 CrossRef CAS PubMed.
- J. S. Wilkes, Green Chem., 2002, 4, 73 RSC.
- H. Matsumoto, M. Yanagida, K. Tanimoto, K. Nomura, Y. Kitagawa and Y. Miyazaki, Chem. Lett., 2000, 29, 922 CrossRef.
- M. Kosmulski, J. Gustafsson and J. B. Rosenholm, Thermochim. Acta, 2004, 412, 47 CrossRef CAS PubMed.
- P. Wasserscheid and W. Keim, Angew. Chem., Int. Ed., 2000, 39, 3772 CrossRef CAS.
- T. Welton, Chem. Rev., 1999, 99, 2071 CrossRef CAS PubMed.
- Q. Yao, Org. Lett., 2002, 4, 2197 CrossRef CAS PubMed.
- J. S. Wilkes, J. A. Levisky, R. A. Wilson and C. L. Hussey, Inorg. Chem., 1982, 21, 1263 CrossRef CAS.
- J. S. Wilkes and M. J. Zaworotko, J. Chem. Soc., Chem. Commun., 1992, 965 RSC.
- T. Welton, Chem. Rev., 1999, 99, 2071 CrossRef CAS PubMed.
- M. E. V. Valkenburg, R. L. Vaughn, M. Williams and J. S. Wilkes, Thermochim. Acta, 2005, 425, 181 CrossRef PubMed.
- D. S. Silvester, Analyst, 2011, 136, 4871 RSC.
- R. F. de Souza, J. C. Padilha, R. S. Goncalves and J. Dupont, Electrochem. Commun., 2003, 5, 728 CrossRef CAS.
- T. Sato, G. Masuda and K. Takagi, Electrochim. Acta, 2004, 49, 3603 CrossRef CAS PubMed.
- F. Zhou, Y. Liang and W. Liu, Chem. Soc. Rev., 2009, 38, 2590 RSC.
- V. Chakrapani, F. Rusli, M. A. Filler and P. A. Kohl, J. Phys. Chem. C, 2011, 115, 22048 CAS.
- M. P. Scott, C. S. Brazel, M. G. Benton, J. W. Mays, J. D. Holbrey and R. D. Rogers, Chem. Commun., 2002, 1370 RSC.
- B. Tang, W. Bi, M. Tian and K. H. Row, J. Chromatogr. B: Anal. Technol. Biomed. Life Sci., 2012, 904, 1 CrossRef CAS PubMed.
- M. Volland, V. Seitz, M. Maase, M. Flores, R. Papp, K. Massonne, V. Stegmann, K. Halaritter, R. Noe, M. Bartsch, W. Siegel, M. Becker and O. Huttenloch, Chem. Eng. News, 2003, 81, 9 Search PubMed.
- A. Kumar, G. Gupta and S. Srivastava, Green Chem., 2011, 13, 2459 RSC.
- S. S. Chavan and M. S. Degani, Green Chem., 2012, 14, 296 RSC.
- V. V. Thorat, S. A. Dake, S. U. Deshmukh, E. Rasokkiyam, M. F. Uddin and R. P. Pawar, Lett. Org. Chem., 2013, 10, 178 CrossRef CAS.
- A. Dandia, R. Singh and D. Saini, J. Chem. Sci., 2013, 125, 1045 CrossRef CAS PubMed.
- S. H. Chen, Q. Zhao and X. W. Xu, J. Chem. Sci., 2008, 120, 481 CrossRef CAS PubMed.
- S. Kanakaraju, B. Prasanna and G. V. P. Chandramouli, J. Chem. Pharm. Res., 2012, 4, 2994 CAS.
- A. R. M. Zare, M. A. Zolfigol, O. Khaledian, V. Khakyzadeh, M. D. Farahani and H. G. Kruger, New J. Chem., 2014, 38, 2342 RSC.
- R. Sugimura, K. Qiao, D. Tomida and C. Yokoyama, Catal. Commun., 2007, 8, 770 CrossRef CAS PubMed.
- N. S. Suryawanshi, P. Jain, M. Singhal and I. Khan, J. Appl. Chem., 2012, 1, 18 CAS.
- Z. Zhou and X. Deng, J. Mol. Catal. A: Chem., 2013, 367, 99 CrossRef CAS PubMed.
- J. Weng, C. Wang, H. Li and Y. Wang, Green Chem., 2006, 8, 96 RSC.
- C. Wang, W. Zhao, H. Li and L. Guo, Green Chem., 2009, 11, 843 RSC.
- H. Jiang, C. Wang, H. Li and Y. Wang, Green Chem., 2006, 8, 1076 RSC.
- E. M. Beccalli, G. Broggini, A. Fasana and M. Rigamonti, J. Organomet. Chem., 2011, 696, 277 CrossRef CAS PubMed.
- K. Bahrami, M. M. Khodaei and A. M. Sheikh, J. Org. Chem., 2010, 75, 6208 CrossRef CAS PubMed.
- N. Fukuda and T. Ikemoto, J. Org. Chem., 2010, 75, 4629 CrossRef CAS PubMed.
- Y. Pandey, P. K. Sharma, N. Kumar and A. Singh, Int. J. PharmTech Res., 2011, 3, 980 CAS.
- V. Gududuru, E. Hurh, J. T. Dalton and D. D. Miller, Bioorg. Med. Chem. Lett., 2004, 14, 5289 CrossRef CAS PubMed.
- A. D. Taranalli, N. V. Thimmaiah, S. Srinivas and E. Saravanan, Asian J. Pharm. Clin. Res., 2009, 2, 79 CAS.
-
(a) J. Balzarini, B. Orzeszko, J. K. Maurin and A. Orzeszko, Eur. J. Med. Chem., 2007, 42, 993 CrossRef CAS PubMed;
(b) M. L. Barreca, J. Balzarini, A. Chimirri, E. D. Clercq, L. D. Luca, H. D. Holtje, M. Holtje, A. M. Monforte, P. Monforte, C. Pannecouque, A. Rao and M. Zappala, J. Med. Chem., 2002, 45, 5410 CrossRef CAS PubMed.
- V. R. Solomon, W. Haq, K. Srivastava, S. K. Puri and S. B. Katti, J. Med. Chem., 2007, 50, 394 CrossRef CAS PubMed.
- A. Agarwal, S. Lata, K. K. Saxena, V. K. Srivastava and A. Kumar, Eur. J. Med. Chem., 2006, 41, 1223 CrossRef CAS PubMed.
- A. Upadhyay, Eur. J. Med. Chem., 2010, 45, 3541 CrossRef CAS PubMed.
- O. Unsal-Tan, K. Ozadali, K. Piskin and A. Balkan, Eur. J. Med. Chem., 2012, 57, 59 CrossRef CAS PubMed.
- M. V. Diurno, O. Mazzoni, E. Piscopo, A. Calignano, F. Giordano and A. Bolognesell, J. Med. Chem., 1992, 35, 2910 CrossRef CAS.
- M. D. Ascenzio, B. Bizzarri, C. D. Monte, S. Carradori, A. Bolasco, D. Secci, D. Rivanera, N. Faulhaber, C. Bordon and L. Jones-Brando, Eur. J. Med. Chem., 2014, 86, 17 CrossRef PubMed.
- T. Kato, T. Ozaki, K. Tamura, Y. Suzuki, M. Akima and N. Ohi, J. Med. Chem., 1998, 41, 4309 CrossRef CAS PubMed.
- X. Zhang, X. Li, D. Li, G. Qua, J. Wang, P. M. Loiseau and X. Fan, Bioorg. Med. Chem. Lett., 2009, 19, 6280 CrossRef CAS PubMed.
- P. T. Holte, B. C. J. V. Esseveldt, L. Thijs and B. Zwanenburg, Eur. J. Org. Chem., 2001, 2001, 2965 CrossRef.
- V. Sriramurthy, G. A. Barcan and O. Kwon, J. Am. Chem. Soc., 2007, 129, 12928 CrossRef CAS PubMed.
- C. Palomo, M. Oiarbide, R. Lopez, P. B. Gonzalez, E. G. Bengoa, J. M. Saa and A. Linden, J. Am. Chem. Soc., 2006, 128, 15236 CrossRef CAS PubMed.
- A. F. Ward and J. P. Wolfe, Org. Lett., 2011, 13, 4728 CrossRef CAS PubMed.
- V. Tiwari and J. S. Meshram, Jordan J. Chem., 2011, 6, 371 CAS.
- R. V. Patel and S. W. Park, Med. Chem. Res., 2014, 23, 4099 CrossRef CAS.
- H. Varshney, A. Ahmad, N. N. Farshori, A. Ahmad, A. U. Khan and A. Rauf, Med. Chem. Res., 2013, 22, 3204 CrossRef CAS.
- T. Nishitani, H. Shiraishi, S. Sakaguchi and Y. Ishii, Tetrahedron Lett., 2000, 41, 3389 CrossRef CAS.
- N. F. Brégeot, A. Voituriez, P. Retailleau and A. Marinetti, Tetrahedron Lett., 2009, 50, 4700 CrossRef PubMed.
- S. J. Brickner, Curr. Pharm. Des., 1996, 2, 175 CAS.
- A. Buzas and F. Gagosz, Synlett, 2006, 2727 CAS.
- R. R. Machín, J. Adrio and J. C. Carretero, J. Org. Chem., 2006, 71, 5023 CrossRef PubMed.
- T. Munegumi, I. Azumaya, T. Kato, H. Masu and S. Saito, Org. Lett., 2006, 8, 379 CrossRef CAS PubMed.
- S. Riyaz, A. Naidu and P. K. Dubey, Synth. Commun., 2011, 41, 2756 CrossRef CAS.
- A. A. Aly, A. B. Brown, M. A. Aziz, G. E. A. A. A. Rahma, M. F. Radwan, M. Ramadan and A. M. G. Eldeen, J. Heterocycl. Chem., 2012, 49, 726 CrossRef CAS.
- E. M. Beccalli, G. Broggini, A. Fasana and M. Rigamonti, J. Organomet. Chem., 2011, 696, 277 CrossRef CAS PubMed.
- C. E. Song, M. Y. Yoon and D. S. Choi, Bull. Korean Chem. Soc., 2005, 26, 1321 CrossRef CAS.
- S. J. S. Suresh, Green Chem. Lett. Rev., 2011, 4, 289 CrossRef.
- S. J. S. Suresh, Green Chem. Lett. Rev., 2011, 4, 311 CrossRef.
- X. Zhang, X. Li, D. Li, G. Qua, J. Wang, P. M. Loiseau and X. Fan, Bioorg. Med. Chem. Lett., 2009, 19, 6280 CrossRef CAS PubMed.
- R. Jain, K. Sharma and D. Kumar, Helv. Chim. Acta, 2013, 96, 414 CrossRef CAS.
- Suresh and J. S. Sandhu, Org. Med. Chem. Lett., 2013, 3, 1 CrossRef PubMed.
- D. V. Jawale, U. R. Pratap, D. L. Lingampalle and R. A. Mane, Chin. J. Chem., 2011, 29, 942 CrossRef CAS.
- K. F. Shelke, S. B. Sapkal, B. R. Madje, B. B. Shingate and M. S. Shingare, Bull. Catal. Soc. India, 2009, 8, 30 Search PubMed.
- J. Zhang, Y. Zhang and Z. Zhou, Green Chem. Lett. Rev., 2014, 7, 90 CrossRef.
- M. Kalantari, Heterocycl. Lett., 2013, 3, 325 CAS.
- M. Parveen, A. M. Malla, A. Ali, M. Alam and M. F. Mustafa, Chem. Res. Chin. Univ., 2014, 30, 55 CrossRef CAS.
- M. Parveen, A. M. Malla, M. Alam, M. Ahmad and S. Rafiq, New J. Chem., 2014, 38, 1655 RSC.
- J. W. Baker and J. B. Holdsworth, J. Chem. Soc., 1947, 713 RSC.
- J. W. Baker and J. Gaunt, J. Chem. Soc., 1949, 9 RSC.
- H. Zhang, Q. Shen and L. Lu, Tetrahedron Lett., 2011, 52, 349 CrossRef CAS PubMed.
- G. Sommen, A. Comel and G. Kirsch, Tetrahedron Lett., 2002, 43, 257 CrossRef CAS.
-
(a) A. Dorsey, P. R. McClure, A. R. McDonald and M. Singh, Toxicological Profile for Toluene (Draft), Agency for Toxic Substances and Disease Registry, U.S. Public Health Service, U.S. Department of Health and Human Services, Atlanta, GA, 1992 Search PubMed;
(b) Integrated Risk Information System on Toluene, U.S. Environmental Protection Agency, Environmental Criteria and Assessment Office, Office of Health and Environmental Assessment, Office of Research and Development, Cincinnati, OH, 1993 Search PubMed.
- G. Shkapenko, G. T. Gmitter and E. E. Gruber, Ind. Eng. Chem., 1960, 52, 605 CrossRef CAS.
- C. Wang, L. Guo, H. Li, Y. Wang, J. Weng and L. Wu, Green Chem., 2006, 8, 603 RSC.
- N. M. Rateb, Phosphorus, Sulfur Silicon Relat. Elem., 2005, 180, 2361 CrossRef CAS.
- K. Lonsdale, International tables for X-Ray crystallography, Kynoch Press, Birmingham, England, 1952, vol. I Search PubMed.
- SAINT, Version 6.02, Bruker AXS, Madison, WI, 1999 Search PubMed.
- G. M. Sheldrick, SADABS: Empirical absorption correction program, Gottingen, University of Gottingen, 1997 Search PubMed.
- XPREP, version 5.1, Siemens industrial automation inc, Madison, WI, 1995 Search PubMed.
- G. M. Sheldrick, SHELXTL Reference Manual, version 5.1, Bruker AXS, Madison, WI, 1997 Search PubMed.
- G. M. Sheldrick, SHELXL-97: Program for crystal structure refinement, Gottingen, University of Gottingen, 1997 Search PubMed.
Footnote |
† Electronic supplementary information (ESI) available. CCDC 917624. For ESI and crystallographic data in CIF or other electronic format see DOI: 10.1039/c4ra15590d |
|
This journal is © The Royal Society of Chemistry 2015 |