DOI:
10.1039/C4RA14162H
(Paper)
RSC Adv., 2015,
5, 20390-20395
Surfactant-assisted electroless deposition of silver nanoparticles on Ge crystal for ultra-sensitive detection by surface-enhanced infrared absorption spectroscopy
Received
9th November 2014
, Accepted 13th February 2015
First published on 13th February 2015
Abstract
Silver nanoparticles (AgNPs) were electrolessly grown on Ge crystal to form substrate for ultra-sensitive detection by surface-enhanced infrared absorption (SEIRA) measurements. To effectively control the morphology of AgNPs, a surfactant-assisted electroless deposition method was proposed in this work. By addition of surfactant into the reaction solution, both reaction rate and behavior in agglomeration of AgNPs were significantly altered. To explore the role of surfactants, three types of surfactants were studied; cationic, anionic and Zwitterionic surfactants. Among them, cationic surfactant of cetyl trimethyl ammonium bromide (CTAB) showed best SEIRA effect as a labile interaction exists between the amine group in CTAB and the formed AgNPs. Also, the hydrophobic long tail of CTAB effectively protects the AgNPs from serious agglomeration. For Zwitterionic surfactants of amino acids, the polar groups on both ends caused severe percolation of AgNPs. With a strong interaction to AgNPs, anionic surfactant of sodium dodecyl benzene sulfonate (SDBS) regulated the AgNPs into a square shaped crystal, which resulted a distortion of infrared absorption band. Based on the obtained results, the substrates prepared with the optimal condition found in this work were at least 5 times higher than that of conventional electroless deposition method. The overall increase of the infrared absorption falls in a range between 300 to 400 times.
Introduction
Since the first report of the phenomenon of surface-enhanced infrared absorption (SEIRA) spectroscopy,1 it has been applied widely to different fields for ultra-trace chemical and biochemical analyses.2–5 The applying of SEIRA effect for analytical purposes have been addressed and successful results have been documented.6–10 However, the applications of this technique for practical usages rely on the methods to prepare sensitive SEIRA substrates, which should be simple and feasible for massive productions. Several types of methods have been developed for preparation of SEIRA substrates including vapor deposition (PVD),5,10,11 lithographic fabrication,12–15 electroless deposition,6,16,17 chemical reductions,18 and electrochemical deposition.19,20 The reported enhancement factors of the prepared SEIRA substrates by these methods are comparable and ranged from one to two orders of magnitude. Among them, electroless deposition method shows the most promising results with an extremely simple procedure in the preparation of the active substrates. Ge and Si discs have been commonly used in electroless deposition method in the past years because of their low IR absorption and capability to reduce noble metal ions spontaneously.6,16,17,21–24 Si disc is low in price but Si has spectral interferences raised from the formation of Si–O. Also, hazardous HF is generally required to remove silicon oxide during electroless deposition. On the other hand, Ge disc is favored to form substrate for SEIRA measurements and the observed enhancement factor is generally in the range of one to two orders of magnitude. The corresponding intensities of symmetric stretching of nitro group in para-nitrothiophenol (pNTP) are around 50 mAU. Although AgNPs could be formed electrolessly on Ge discs, the manipulation of the morphology of the AgNPs is very restricted and relies only on the chemical nature of the metals. Therefore, we propose a surfactant-assisted electroless deposition method to manipulate the formation of AgNPs for improving the sensitivity in SEIRA measurements.
As shown in Fig. 1a, the addition of surfactant into reaction solution of silver nitrate can effectively alter the behavior in formation of AgNPs during electroless deposition. The added surfactant works as a protecting agent to protect the formed AgNPs from further growth or agglomeration into unfavored sizes or shapes of the AgNPs. Also, surfactant interacts with silver ions to form complex, which can alter the reaction behavior during electroless deposition. To explore the roles of the surfactants, six surfactants are employed and can be classified into cationic, anionic and Zwitterionic surfactants as shown in Fig. 1b. Cetyl trimethyl ammonium bromide (CTAB) was used to represent the cationic surfactants. Sodium dodecyl benzene sulfonate (SDBS) is used to represent anionic surfactants. Four amino acids of leucine (Leu), phenylalanine (Phe), glutamate (Glu) and lysine (Lys) are used to represent Zwitterionic surfactants. Leu and Phe have a hydrophobic tail opposite to the amino acid group. Comparing to Leu, hydrophobic tail of Phe composed with a phenyl ring provides an extra chemical force in arranging the molecules into a more compact adsorption layer on the surface of AgNPs. Glu and Lys compose an addition polar functional group (carboxylate or amine group) opposited to the amino acid group.
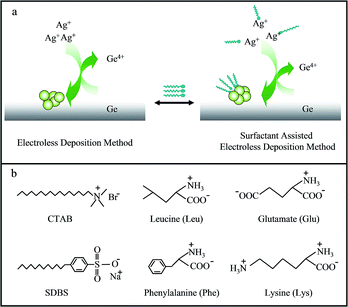 |
| Fig. 1 (a) Schematic diagram in preparation of AgNPs on Ge disc with and without addition of surfactants. (b) Chemical structures of used surfactants. | |
Experimental section
Chemicals
Silver nitrate was purchased from ProChem (Rockford, IL). para-Nitrothiophenol (pNTP) and lysine were purchased from Aldrich (Milwaukee, WI). Cetyl trimethylammonium bromide (CTAB), phenylalanine (Phe) and leucine (Leu) were purchased from Acros (Phillipsburg, NJ). Glutamate (Glu) was purchased from Alfa Aesar (Lones, UK). Sodium dodecyl benzene sulfonate (SDBS) was purchased from TCI Chemical Company (Tokyo, Japan). Spectroscopic-grade methanol was purchased from J.T. Baker (Phillipsburg, NJ). De-ionized water was used for the preparation of all aqueous solutions. Ge discs with a diameter of 1.2 cm were purchased from Lattice materials Co (Bozeman, MT). Ge discs were polished with 0.05 μm aluminum oxide (Buehler, Lake Bluff, IL). After cleaned with deionized water, Ge discs were soaked in methanol for 2 min and dried in air. Ge discs were further placed into 80 °C oven for 20 min. After cooling down to room temperature, Ge discs were placed into Petri dish and ready for electroless deposition. To re-use the Ge discs, these pretreatment procedures described above were followed.
Method in preparation of AgNPs on Ge substrates
AgNPs were formed on Ge discs by simply placing Ge discs into a Petri dish containing plating solution for a certain reaction time. Plating solutions were prepared by mixing 1 mM silver nitrate with different concentrations of surfactants. After a reaction time, the Ge discs were removed from the plating solution and placed into another Petri dish containing deionized water for 5 min to rinse off any residual chemicals. To probe the SEIRA effect, prepared substrates were soaked into 1 mM pNTP methanoic solution for 1 h to chemisorb pNTP on the surface of AgNPs.
IR measurement
Transmission spectra were collected using a Spectrum One FT-IR spectrometer (Perkin Elmer) with coadding of 4 scans at 4 cm−1 resolution. A home-made Ge holder was placed in the sample compartment at the focus of the IR beam. A bare Ge disc was always used as reference.
Results and discussion
Role of surfactant in preparation of AgNPs@Ge substrate
To examine the influences of surfactants, reaction solutions containing 1 mM silver nitrate and 0.5 mM of different surfactants were prepared. By soaking the Ge discs into these reaction solutions for certain times, the formed substrates were probed with pNTP to examine their performances in SEIRA measurements. Fig. 2 shows the observed spectra of substrates prepared with addition of 0.5 mM Leu, Phe, Glu, and Lys for a reaction time of 3.5, 10, 2.5 and 1 min, respectively. For SDBS and CTAB, the reaction times were 10 and 60 min, respectively. As can be seen in Fig. 2, the observed spectra show similar spectral features to the literature reports.12,16 Also, substrates prepared with CTAB gave the most pronounced SEIRA effect among the examined surfactants. When SDBS was used, serious distortion of absorption band was observed revealing that the formed AgNPs could be percolated easily with addition of this surfactant. Band distortion was also observable even the reaction time was shortened to 1 min. This behavior of SDBS is likely caused by the strong interaction between SDBS and AgNPs as absorption bands of SO3− stretching located around 1100 cm−1 were still observable. For other surfactants, no spectral features raised from the surfactant itself could be observed indicating that these surfactants interact weakly with AgNPs. On the other hand, functional groups of amine and carboxylate interact with AgNPs weaker than that of sulfonate group. For quantitative purpose, the NO2 symmetric stretching band at 1340 cm−1 was selected for further quantitative studies.
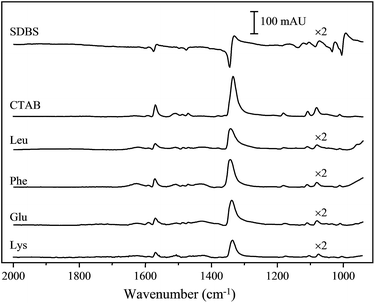 |
| Fig. 2 Spectra of pNTP on AgNPs@Ge substrates. The reaction solution composed 1 mM silver nitrate and 0.5 mM of SDBS, CTAB, Leu, Phe, Glu or Lys. The plotted spectra were selected with a reaction time of 3.5, 10, 2.5 and 1 min for Leu, Phe, Glu and Lys, respectively. The reaction times for SDBS and CTAB are 10 and 60 min, respectively. | |
By plotting the band intensity at 1340 cm−1 against the reaction time for different surfactants, the obtained reaction time profiles are plotted in Fig. 3 for 1 mM silver nitrate solution mixed with 0.5 mM Leu, Phe, Glu, or Lys. Because SDBS caused serious distortion of the absorption bands, the reaction time profile for SDBS is not plotted. The response time profile of 0.5 mM CTAB is plotted separately in Fig. 4 to have a clearer view. In Fig. 4, response time profiles of 0, 0.25, 0.75 and 1 mM CTAB are also plotted for later analyses.
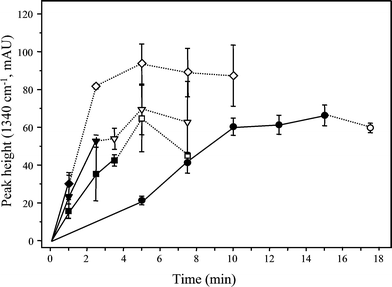 |
| Fig. 3 SEIRA signals of 500 ng cm−2 pNTP on AgNPs@Ge substrates prepared using: 1 mM AgNO3 with 0.5 mM of Leu (■), Phe (●), Glu (▼) or Lys (◆). Derivative band shape was observed and plotted with dash lines and blank symbols. Error bars represent the standard deviation from triplicate runs. | |
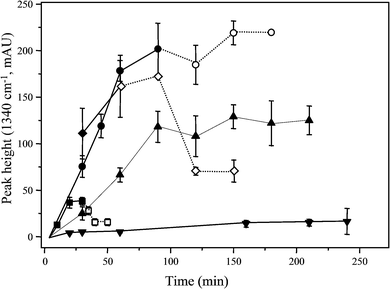 |
| Fig. 4 SEIRA signals of pNTP on AgNPs@Ge substrates prepared using 1 mM AgNO3 with 0 (■); 0.25 (◆), 0.5 (●), 0.75 (▲) and 1 mM CTAB (▼). Derivative band shape was observed and plotted with dash lines and blank symbols. Error bars represent the standard deviation from triplicate runs. | |
In general, derivative band shape can be observed when AgNPs start to merge together (a condition known as percolation). Once absorption band is distorted, large uncertainty is associated in the estimated band intensity. On the other hand, percolated AgNPs are not suitable for quantitative purpose and should be avoid. As can be seen in Fig. 3, the time to have a band distortion differs significantly between the examined surfactants. With addition of bi-functional surfactants of Glu and Lys, the time to have a band distortion was less than 5 min. This short reaction time verifies that silver ions and AgNPs can be effectively attracted by the polar functional groups in each end of these amino acids. Based on the reaction time to have band distortion, Lys provides better attraction ability as the percolation time for Lys was shorter than that of Glu. On the other hand, the attraction of silver ions is more effective with a negative charged carboxylate group than a positive charged amine group. For amino acids with hydrophobic tails, Phe showed a longer reaction times to form percolated AgNPs than any examined amino acid. The longest reaction time for Phe than other examined amino acids indicates that the phenyl ring in the tail end of Phe provide additional force in formation of blocking layer on AgNPs to slow down the reaction. However, the time to reach percolation of AgNPs was around 30 min for the reaction without addition of surfactant as plotted in Fig. 4. The addition of any of the examined surfactant can certainly shorten the reaction time even an amino acid with a hydrophobic tail. On the other hand, Zwitterionic surfactants are not suitable for controlling the morphologies of AgNPs in electroless deposition method.
With addition of 0.5 mM CTAB, the reaction time profile is plotted in Fig. 4. The reaction time to have percolation was extended to several hours and the highest SEIRA signal was observed around a reaction time of 90 min. This large increase of the reaction time to have percolation reveals that the silver ions are not easily accessible to the surface of the reduced AgNPs due to the formation of hydrophobic layer by the attaching of CTAB. However, the increase of the reaction time to have percolation could be also caused by the decrease of the concentration of free silver ion through formation of Ag+–CTAB complexes in the solution. Comparing with the maximal signals obtained by conventional electroless deposition (no addition of surfactant), an increase of SEIRA signal with a factor of 5 could be obtained when 0.5 mM CTAB was added. The corresponding overall enhancement factor for the substrates prepared in this work approaches 300 to 400.
To observe the morphologies of the formed AgNPs, substrates prepared by 1 mM silver nitrate alone (30 min) or with addition of 0.5 mM of SDBS (10 min), CTAB (90 min), Leu (5 min), Phe (5 min), Glu (5 min) and Lys (5 min) were scanned by SEM. The obtained images are shown in Fig. 5a to g, respectively. As can be seen in these images, without the addition of a surfactant, the formed AgNPs were slightly attached to each other with a particle size of 80 nm (see Fig. 5a). When SDBS was added, square shaped Ag crystals were observed, which could be caused by the restriction of the crystal growing direction from the strongly attached SDBS. When CTAB was added, AgNPs with 100 nm in diameter were formed and the particle density was higher than without addition of a surfactant as shown in Fig. 5c.
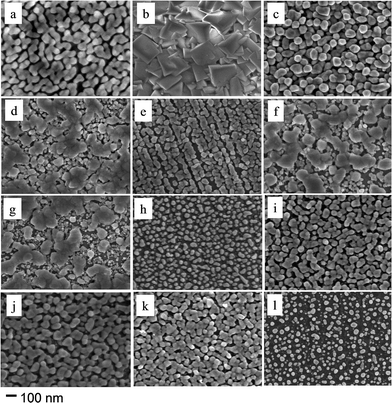 |
| Fig. 5 SEM images of substrates prepared by reacting in 1 mM silver nitrate alone for 30 min (a), with surfactants of 0.5 mM SDBS for 10 min (b), 0.5 mM CTAB for 90 min (c), 0.5 mM Leu for 5 min (d), 0.5 mM Phe for 5 min (e), 0.5 mM Glu for 5 min (f), 0.5 mM Lys for 5 min (g), 0.5 mM CTAB for 10 min (h), 0.5 mM CTAB for 45 min (i), 0.25 mM CTAB for 30 min (j), 0.75 mM CTAB for 60 min (k), and 1 mM CTAB for 210 min (l). | |
With addition of amino acids, the formed AgNPs were seriously percolated as can be seen in Fig. 5d to g for Leu, Phe, Glu, and Lys, respectively. These amino acids bear with functional groups of carboxylate and amine, which could both interact with AgNPs or silver ions to cause percolation of the AgNPs. Although Leu has a hydrophobic tail, the di-methyl group in the tail end may exhibit high hindering effect and can not form well packed hydrophobic layer to reduce percolation of AgNPs. Unlike Leu, Phe has a phenyl group to help the formation of more stable and well packed layer on the AgNPs for preventing percolation. The image in Fig. 5e verifies that the formed AgNPs were more isolated by Phe than by other amino acids. Again, these images prove that any of Zwitterionic surfactant is indeed not a good choice to regulate the AgNPs morphology for SEIRA measurements.
To further observe the processes in formation of AgNPs, the substrates prepared with addition of 0.5 mM CTAB for a reaction time of 10 and 45 min were also scanned and plotted in Fig. 5h and i, respectively. Along with the SEM image in Fig. 5c (90 min), the AgNPs were round in shape with any of the reaction time. Also, the particle size of AgNPs was gradually increased along with the reaction time. This reveal that adsorbed CTAB molecules can protect the AgNPs to form percolated AgNPs. Also, the protecting layer of CTAB is still labile enough to allow further growth of the AgNPs.
Effect of concentration of CTAB in preparation of AgNPs@Ge substrates
To study the effect of concentration of CTAB, the concentration of silver nitrate was kept at 1 mM and the concentrations of CTAB were varied to 0, 0.25, 0.5, 0.75 and 1 mM. The concentration of CTAB was limited to 1 mM because CTAB exhibits a critical micelle concentration (CMC) around 1 mM.25 The formed substrates were probed with pNTP and the obtained reaction time profiles are also shown in Fig. 4. SEM images of substrates prepared by 0.25 mM CTAB (30 min), 0.75 mM CTAB (90 min) and 1 mM CTAB (210 min) are shown in Fig. 5j to l, respectively. As can be seen in Fig. 4, the increase in the concentration of CTAB caused a decrease of reaction rate in growing AgNPs into a suitable size for SEIRA measurements. The maximal SEIRA intensities were also different as 0.5 mM and 0.75 mM CTAB having maximal band intensities of 200 mAU and 120 mAU. This difference reveals that concentration of CTAB not only affects the growing rate but also the morphologies of the particles. The increase in the concentration of CTAB resulted in a decrease of reaction rate implies either CTAB blocking the active reaction area or the CTAB interacting with silver ions to reduce the amount of free silver ions. When concentration of CTAB was changed to 0.25 mM, the reaction was fast and a suitable size of AgNPs could be formed within 30 min as can be seen in Fig. 5j. Compared the images in Fig. 5i and k, the formed particles were less regular as the concentration of CTAB increased from 0.5 mM to 0.75 mM. Small AgNPs were observed for the substrate prepared with 1 mM of CTAB even the reaction time was extended to 210 min (see Fig. 5l).
Effect of silver nitrate concentration
The addition of CTAB into the silver nitrate solution decreases the reaction rate significantly. This decrease of reaction rate can be caused by formation a thin hydrophobic layer on AgNPs to block the reaction or formation of Ag+–CTAB complex to reduce the amount of free silver ions in the solution. To study the contributions from these two behaviors, the concentration of silver nitrate was varied but the concentration of CTAB was kept 0.5 mM lower than the concentration of silver nitrate. In such case, the prepared solutions have the same free silver ion concentration of 0.5 mM if the formation of Ag+–CTAB complex is 1
:
1 reaction. By soaking Ge discs into these solutions with different reaction times, the prepared substrates were probed with pNTP and the obtained reaction time profiles are plotted in Fig. 6 for 1, 1.5, and 1.75 mM silver nitrate. If the concentration of free silver ion is the main factor in the growing of AgNPs, there will be no differences between these treatments as an excess of the silver nitrate concentration is kept at 0.5 mM. As plotted in Fig. 6, the maximal SEIRA intensities for these treatments are similar to each other but the time to reach the maximal signal was extended when higher concentration of silver nitrate was used. The similarity in maximal band intensity indicates that the final formed AgNPs are similar in morphology. However, the increase of the reaction time to obtain the maximal signal was inversely related to the concentration of CTAB indicating that the concentration of CTAB also plays a role as a blocking agent to slow down the growth of AgNPs.
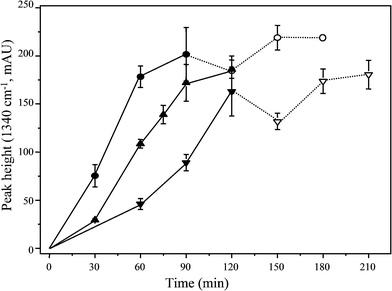 |
| Fig. 6 SEIRA signal of pNTP on AgNPs@Ge substrates prepared by soaking in different concentrations of AgNO3. The concentration of CTAB was kept 0.5 mM lower than that of silver nitrate. The concentrations of silver nitrate were varied to 1 (●), 1.5 (▲), and 1.75 mM (▼). Derivative band shape was observed and plotted with dash lines and blank symbols. Error bars represent the standard deviation from triplicate runs. | |
Effect of concentration of reaction solution in preparation of AgNPs@Ge
The amino group in the CTAB interacts with silver ions and AgNPs. To further understand the roles of silver ion and CTAB, reaction solutions containing silver nitrate and CTAB in same mole ratio of 2
:
1 (AgNO3
:
CTAB = 2
:
1) were prepared. By soaking Ge discs into these solutions for different reaction times, the prepared substrates were probed with pNTP and the obtained reaction time profiles are plotted in Fig. 7 for 0.5, 0.75, 1.0, and 1.5 mM silver nitrate. As can be seen in this graph, the maximal band intensities were similar to each other. Meanwhile, the reaction time to reach the maximal intensity was inversely proportional to the concentration of silver nitrate. Because the mole ratio was kept constant, the concentration of free silver ions was proportional to the concentration of silver nitrate. On the other hand, silver nitrate concentration only affects the reaction rate under the condition that mole ratio between silver nitrate and CTAB remains constant.
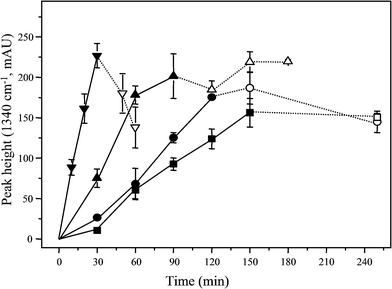 |
| Fig. 7 SEIRA signal of pNTP on AgNPs@Ge substrates prepared by soaking in solutions composed of AgNO3 and CTAB in a mole ratio of 2 : 1. The concentrations of AgNO3 were varied to 0.5 (■), 0.75 (●), 1 (▲) and 1.5 mM (▼). Derivative band shape was observed and plotted with dash lines and empty symbols. Error bars represent the standard deviation from triplicate runs. | |
Conclusions
Three types of surfactants were used and examined in this work to facilitate the production of suitable AgNPs for SEIRA measurements. Among them, Zwitterionic surfactant and surfactants with a strong interaction with AgNPs are not suitable to prepare AgNPs for SEIRA measurements. With the addition of suitable surfactant, such as CTAB, to the reaction solution of silver nitrate, the produced AgNPs meet the requirement to have intense SEIRA effect. The provided surface enhancement factor is at least 5 times higher than conventional electroless deposition method. This enlargement gives an overall enhancement factor of 400. The parameters to control the particle size of AgNPs were studied in detail and the influences of the concentration of silver nitrate, CTAB, and their relative amounts were examined. Results indicated that the concentration of free silver ion plays an important role in preparation of AgNPs on Ge disc. The addition of surfactant can clearly control the size of the AgNPs electrolessly grown on Ge disc and hence, significant improvement of the SEIRA substrate was approached in this work.
Acknowledgements
Financial assistance from Ministry of Science and Technology of Republic of China is gratefully acknowledged.
References
- A. Hartstein, J. R. Kirtley and J. C. Tsang, Phys. Rev. Lett., 1980, 45, 201 CrossRef CAS.
- M. Osawa, Surface-enhanced infrared absorption spectroscopy, in Handbook of Vibrational Spectroscopy, ed. J. M.Chalmers and P. R. Griffiths, John Wiley and Sons, New York, 2002, pp. 785–799 Search PubMed.
- M. Osawa, K. Ataka, K. Yoshii and Y. Nishikawa, Appl. Spectrosc., 1993, 47, 1497 CrossRef CAS.
- R. F. Aroca, D. J. Ross and C. Domingo, Appl. Spectrosc., 2004, 58, 324A CrossRef CAS PubMed.
- G. T. Merklin and P. R. Griffiths, J. Phys. Chem. B, 1997, 101, 5810 CrossRef CAS.
- K. Ataka and J. Heberle, Anal. Bioanal. Chem., 2007, 288, 29 Search PubMed.
- R.-L. J. Cheng and J. Yang, Analyst, 2011, 136, 2988 RSC.
- Y. Nishika and K. Fujiwara, Appl. Spectrosc., 1991, 45, 747 CrossRef.
- G. I. Dovbeshko, V. I. Chegel, N. Y. Gridina, O. P. Repnytska, Y. M. Shirshov, V. P. Tryndiak, I. N. Todor and G. I. Solyanik, Biopolymers, 2002, 67, 470 CrossRef CAS PubMed.
- G. P. C. Rao and J. Yang, Anal. Bioanal. Chem., 2011, 401, 2935 CrossRef CAS PubMed.
- D. A. Heaps and P. R. Griffiths, Vib. Spectrosc., 2006, 41, 221 CrossRef CAS PubMed.
- G. T. Merklin and P. R. Griffiths, Langmuir, 1997, 101, 5810 CAS.
- H. Wang, J. Kundu and N. J. Halas, Angew. Chem., Int. Ed., 2007, 46, 9040 CrossRef CAS PubMed.
- J. Kundu, F. Le, P. Nordlander and N. J. Halas, Chem. Phys. Lett., 2008, 452, 115 CrossRef CAS PubMed.
- R. Bukasov and J. S. S. Parry, Anal. Chem., 2009, 81, 4531 CrossRef CAS PubMed.
- J. Yang and P. R. Griffiths, Anal. Bioanal. Chem., 2007, 388, 109 CrossRef CAS PubMed.
- R.-L. J. Cheng and J. Yang, Appl. Spectrosc., 2010, 64, 219 CrossRef PubMed.
- J. Yang and S. C. Chen, Appl. Spectrosc., 2001, 55, 399 CrossRef CAS.
- H. Miyake and M. Osawa, Chem. Lett., 2004, 33, 278 CrossRef CAS.
- H. Miyake, E. Hosono, M. Osawa and T. Okada, Chem. Phys. Lett., 2006, 428, 451 CrossRef CAS PubMed.
- BhuvAna and G. U. Kulkarni, Bull. Mater. Sci., 2006, 29, 505 CrossRef CAS.
- S. G. dos Santo, L. F. O. Martins, P. C. T. D'Ajello, A. A. Pasa and C. M. Hasenack, Microelectron. Eng., 1997, 33, 59 CrossRef.
- C. B. Li, K. Fobelets and Z. A. K. Durrani, Appl. Mech. Mater., 2011, 110–116, 3284 CrossRef.
- N. Singh, A. K. Srivastava, K. N. Sood and A. Dhar, Mater. Tech., 2013, 28, 199 CrossRef CAS PubMed.
- U. M. Neugebauer, Methods Enzymol., 1990, 182, 239 Search PubMed.
|
This journal is © The Royal Society of Chemistry 2015 |