DOI:
10.1039/C4RA12979B
(Paper)
RSC Adv., 2015,
5, 13107-13111
High-efficient inhibition of recognition in allorejection via a pMyD88/liposomes complex†
Received
23rd October 2014
, Accepted 6th January 2015
First published on 6th January 2015
Abstract
Data are emerging that the recognition of foreign antigens by Toll/like receptors (TLRs) was predominant in skin graft rejection. More interestingly, most of the TLRs recruit myeloid differentiation factor 88 (MyD88) as an adaptor during signaling transduction. Design of an efficient shRNA/vector complex to suppress the expression of MyD88 protein seems to be a potential approach in preventing allorejection of skin. In this study, we prepared a complex composed of a MyD88-shRNA plasmid (pMyD88) and a cationic polymeric vector. The results showed that the pMyD88 and vectors with an optimal mass ratio (1
:
2) was selected by agarose gel electrophoresis (AGE) experiments; moreover, the cationic liposome vectors increased the transfection efficiency of naked pMyD88, and the gene transcription of MyD88 mRNA and expression of MyD88 protein was significantly suppressed by the pMyD88/liposomes complex. Notably, the lipopolysaccharide (LPS) stimulation test showed that the recognition of foreign antigen in DCs, which are treated with the pMyD88/liposomes complex, was significantly inhibited. This attempt promises to solve skin allorejection problems in the future.
1. Introduction
The transplantation of skin is the most effective therapeutic option for large-area burn patients. However, rejection always comes to the graft after the operation. Unlike heart, pancreas and kidney allografts, current immunosuppressors have little effect in skin transplantation.1–3 Though artificial skins are being research upon, they are not clinically effective. Therefore, new strategies have a strong demand to prevent allorejection of skin.
Once the allogeneic skin patch is placed on the injury, toll-like receptors (TLRs) first respond, and then induce DCs to migrate from the skin graft to the lymphoid tissue of the recipient.4 TLRs are a family of transmembrane proteins that play an important role in innate immunity and provide a link between innate and adaptive responses to an allograft.5–8 There at least 11 types of known TLRs molecules. More interestingly, most of them (TLR1, TLR2, TLR4, TLR5, TLR6, TLR7, TLR9, TLR11) require myeloid differentiation factor 88 (MyD88) as an adaptor during signal transduction1,9–12 (as shown in Fig. 1). MyD88 protein contains a molecular structure with a Toll/IL-1R (TIR) domain and a death domain.13 The former is identified to be involved in the activation of TLRs, while the latter propagates a signal by capturing downstream signaling intermediates. Data are emerging that TLR signaling through the MyD88 adaptor cause downstream effects, which include pro-inflammatory cytokines/typeI interferon (IFNs) release.2,14 Many reports have demonstrated that the skin grafts in mice with targeted deletion of the MyD88 adaptor protein received allograft acceptance without rejection.1,15 In addition, MyD88 was also required for the induction of T cell proliferation and the production of the acute phase proteins.16 Therefore, MyD88 is an ideal target to prevent the signal transduction of TLRs for recognizing and inhibiting immune response.
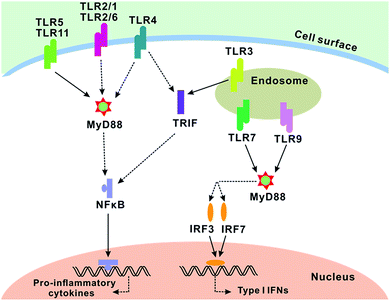 |
| Fig. 1 TLR signaling in conventional dendritic cells. TLR2 (in association with TLR1 or TLR6), TLR4, TLR5 and TLR11 are localized on the cell surface for ligand recognition. TLR3, TLR7 and TLR9 are localized in the endosome. All TLRs, except TLR3, recruit MyD88. TLR4 and TLR6 recruit other additional adaptors. Dendritic cells stimulated with TLR1, TLR2, TLR5, TLR6, TLR11 ligands initiate the MyD88-dependent pathway. MyD88 activated NFκB and IRFs via complicated interactions. NFκB initiated the transcription of pro-inflammatory cytokines, whereas IRFs initiate the transcription of typeI interferons. | |
RNA interference (RNAi) is widely used to introduce an exogenous specific siRNA to the cells for the degradation of targeted mRNA,17 which leads to relative gene silencing. The shRNA is an enhanced approach of RNAi for inhibiting the expression of unwanted protein.18 Herein, we anticipated to block the signal transduction of TLRs, to obtain the results, by employing shRNA to suppress the expression of MyD88. However, shRNA has a difficulty in reaching the targeted cells because they lack serum stability and rapid clearance. Thus, a reliable vector holds the significance of protecting shRNA from nuclease, serum protein and RES capture. Conventional viral vectors can trigger insertional mutagenesis and immunological problems, despite of the fact they have high delivery efficiency.19,20 Nonviral delivery systems provide some advantages over viral vectors, including safety of the materials, simplicity of preparation, ability to load plasmids of any size and multifunctional modifiability. In our previous study,21,22 we successfully synthesized a cationic polymer liposomes-based vector with three advantages: (1) the vectors with high transfection efficiency are easily prepared; (2) the cationic polymer can stably condense genes via electrostatic interactions; (3) nano-shells formed by octadecyl-quaternized lysine modified Chitosan (OQLCS) with positive charges have a good dispersibility.
In this study, we proposed a new approach on inhibiting immune recognition by delivering a shRNA of MyD88 with a cationic polymeric liposomes vector. We expected that this method can efficiently inhibit the expression of MyD88, resulting in failed immune recognition. This attempt promised to have potential in solving immune-related diseases.
2. Materials and methods
2.1 Construction of shRNA of MyD88 plasmid
The MyD88 complementary shRNA was inserted into the Bam HI and Hind III restriction sites of the I pGenesil-1 expression vector containing EGFP sequences (Wuhan Genesil Bio-technology, Wuhan, China). The shRNA was cloning specific sequences: GCC TCT CGC TGT TCT TGAA. Primer 1 (5′-AGG ACA AAC GCC GGA ACT TTT-3′) and primer 2 (5′-GCC GAT AGT CTG TCT GTT CTA GT-3′) were set as primers of the target genes and gene of β-actin (primer 1: 5′-GTC GTA CCA CTG GCA TTG TG-3′, primer 2: 5′-CTC TCA GCT GTG GTGGTG AA-3′) was set as the reference gene. The formed MyD88-shRNA plasmid was named as pMyD88. In addition, the control plasmid pHK was also constructed by cloning a non-specific shRNA sequence into the same restriction sites.
2.2 Preparation of the cationic polymer liposomes system
We have successfully synthesized an excellent cationic polymer liposomes (OQLCS) vector for genes in our previous study.21,22 Amphiphilic molecules OQLCS (180 μg) and cholesterol (60 μg) dissolved in 3 mL chloroform was assembled to form a nanostructure in 6 mL distilled water under an ultrasonic bath. After the removal of chloroform using a vacuum rotary evaporator, the residue was dispersed in distilled water. Subsequently, 0.5 mg SPDP in 1 mL water was added to the lipid solution. After stirring the mixture for 2 h, the solution was purified by dialysis with a 10
000 MW cutoff membrane for 4 h. Then, 1 mL of TAT solution (0.01 M) was added, and the mixture was incubated overnight at 4 °C. Finally we obtained the liposomes vectors on purification using dialysis.
2.3 Optimization of pMyD88/liposomes complex
The formed pMyD88 was incubated with the liposomes at different mass ratio (1
:
0, 1
:
0.5, 1
:
1, 1
:
2, 1
:
2.5, 1
:
3, and 1
:
3.5). Plasmids were loaded onto the surface of liposomes via electrostatic interaction. The systems with different mass ratio were detected and screened by agarose gel electrophoresis (AGE) experiment. The optimum mass ratio selected was used in the subsequence experiments.
2.4 Cell culture and cell transfection
Rats' myeloid dendritic cells (DCs, from Cell bank, Chinese academy of medical science) were cultured in complete medium (Dulbecco's minimal essential medium with 10% fetal calf serum, 1% penicillin and streptomycin) at 37 °C in a humidified atmosphere of 5% CO2. After treating with 0.25% Trysin-EDTA, the cells were resuspended in a complete medium at a concentration of 1 × 105 cells per mL were seeded on the plates. 2 μg naked pMyD88, 1 μL lipofectamine 2000 and 2 μg pMyD88/liposomes complex were added to 50 μL opti-MEM, and the mixtures were incubated for 30 min. Then the mixtures were transferred into the seeded cells. 50 μL opti-MEM was used as the control. Subsequently, 500 μL of complete medium were added into all the wells. After incubating for 48 h, the transfection efficiency was detected using fluorescence microscopy and flow cytometry (FAC-Scalibur, Becton Dickinson, Franklin Lakes, NJ, USA).
2.5 Examination of gene silence effect in transfected DCs
The transfected cells were collected in Eppendorf tubes. After adding 1 mL Trizol and 0.2 mL CHCL3 to the tube, the mixture was left standing for 3 min. Next, the mixture was centrifuged and the supernatant was washed three times with ethyl alcohol. Then 1 μL mRNA extract was used for reverse transcription to obtain cDNA. 1.0 μL cDNA, 9.0 μL SYBR MasterMix, 1.0 μL primer 1 (10 μM), and 1.0 μL primer 2 (10 μM) was added to 2.0 μL double distilled water, then 20 μL of the mixture was used for amplification with Real Time PCR (Bio-Rad, Hercules, CA). The β-actin was set as a reference gene for PCR analysis.
At the same time, the MyD88 protein was determined by western blot analysis. The rest of the collected cells were treated with lysis buffer (RIPA 1.0 mL and PMSF 10 μL) to obtain proteins. The proteins were separated using the SDS-PAGE method. Separated proteins were transferred to a nitrocellulose membrane, which was incubated with blocking buffer for 1 h. The membrane was washed with PBST three times before being incubated with rabbit-anti-rat MyD88 antibody (1
:
200) at 4 °C overnight. After washing the membrane with PBST three times, goat-anti-rabbit HRP-tagged antibody (1
:
1000) was added and incubated for 1 h. Then, the membrane was washed and treated with chemiluminescence reagents. Finally, the membrane was used for detection, and the images were analyzed with Image-Pro Plus 6.0 software. Rabbit-anti-rat GAPDH antibody was used to test the reference protein.
2.6 Investigation on immunogenicity of transfected DCs
1.6 μg LPS was dissolved in 80 μL of opti-MEM to obtain LPS solution. Transfected cells were collected for resuspension in a complete media at 1 × 105 cells per mL. 180 μL resuspended solution was incubated with 20 μL LPS solutions for 3 days. 20 μL of opti-MEM solution was used as the control.
After being cultured for 3 days, IFN-Γ and IL-2 concentrations in the supernatant from the cultured cells were examined using ELISA kit. Subsequently, the blank wells, standard wells and testing wells were incubated for 2 h, and the supernatant was discarded and corresponding antibody was added into each well. The wells were washed with PBST 5 times after incubating for 1 h. Then 90 μL TMB was added into the well followed by incubation for 30 min. Finally, the optical density was read by Safire 2 at a wavelength of 450 nm. Standard curve and sample density was calculated by the applied software of Safire 2. At the same time, the cells were collected for the examination of MyD88 protein with western blot.
The expression of MHC II protein was tested by flow cytometry. Phycoerythrin-conjugated mouse-anti-rat MHC II antibody was used to incubate cells for 30 min in the dark. Then the DCs were analyzed by flow cytometry.
3. Results and discussions
3.1 Optimization of pMyD88/liposomes complex
The MyD88 complementary shRNA was inserted in to the plasmid containing enhanced green fluorescent protein (EGFP) sequences (as shown in Fig. 2). The resulting recombinant MyD88-shNRA pGenesil-1 was named as plasmid shRNA-MyD88 (pMyD88). EGFP can be observed using fluorescence microscopy, which was used as a marker of exogenously administered MyD88-shRNA. The same plasmid with a non-specific shRNA (pHK) was used as the control.
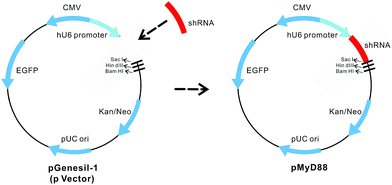 |
| Fig. 2 Schematic illustration of the pMyD88 design. The shRNA-pMyD88 was inserted into the plasmid. | |
The liposome vectors with sufficient positive charges condensed genes to form a pMyD88/liposomes complex as shown in Fig. 3(1). Charge density influenced the electrostatic interaction between pMyD88 and cationic polymeric liposomes. Therefore, in order to obtain stable complexes, AGE was used to screen the optimal mass ratio between the two components in their assembled attachment. As shown in Fig. 3(2), when the mass ratio equals to 1
:
2 or is higher than 1
:
2, no separated plasmid was observed. Thus, the mass ratio of 1
:
2 was selected as the optical ratio and was used in all subsequent experiments.
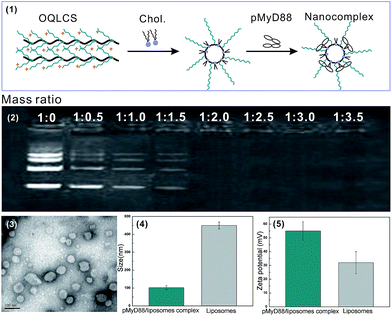 |
| Fig. 3 Preparation and characterization of the optimal pMyD88/liposomes complex. (1) The formation of the pMyD88/liposomes complex. (2) The complex with different weight ratio of pMyD88 to liposomes was optimized by AGE. (3) TEM image of the optimal complex. (4) Average size of the liposomes and optimal complex. (5) Zeta potential of the liposomes and the optimal complex. | |
The morphology of the optimal complex was characterized by TEM. From the Fig. 3(3), we can see a uniform distribution and a diameter less than 100 nm, most of which were about 70 nm. Results from DLS and surface zeta potential analysis showed the average size and zeta potential of the liposomes and the complexes. In Fig. 3(4), the average size of pMyD88/liposomes complexes was about 95 nm, whereas the liposomes had a much larger size of 400 nm. There was sufficient positive charge on the surface of the liposomes since surface zeta potential was 54 mV as shown in Fig. 3(5). The zeta potential decreased with the combination of negatively charged pMyD88. The addition of shRNA condensed the liposomes via electrostatic interaction, which resulted in complex with much smaller size in the DLS analysis. Moreover, the decreased zeta potential confirmed the formation of the pMyD88/liposomes complexes.
3.2 Evaluation of transfection efficiency of the complex in DCs
After characterizing the optical complex formation, we investigated the pMyD88/liposomes complex transfection ability in rats' DCs, with naked pMyD88 and lipofectamine as the controls. Firstly, the uptake of exogenously administered pMyD88 was observed under a fluorescence microscope with the help of enhanced green fluorescent protein (EGFP). The strong green fluorescence came from the transfected cells, which indicates that the plasmid was successfully delivered to the cells. It is clear from Fig. 4 that the liposomes vector enhanced the transfection efficiency of the naked pMyD88. Flow cytometry was used for the quantitative analysis of transfection efficiency. On comparing the values of the four groups, the transfection efficiency of the pMyD88/liposomes complex was the strongest (28.19 ± 5.38), which indicates that the liposomes system significantly enhanced the transfection efficiency of naked pMyD88 (1.25 ± 0.86).
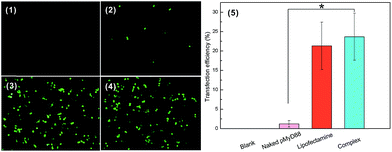 |
| Fig. 4 Transfection efficiency of the complex. (1)–(4) are fluorescence images: (1) blank media, (2) media with pMyD88, (3) media with lipofectamine, (4) media with the pMyD88/liposomes complex, and (5) flow cytometry analysis of intracellular delivery efficiency. The data are the mean ± SE (n = 6). * P < 0.01 as compared with the cells treated with naked pMyD88 (ANOVA, analysis of variance). | |
3.3 Gene silence effect of pMyD88/liposomes complex
In order to assess the gene silencing effect of pMyD88/liposomes complex, we investigated MyD88 gene transcription level and protein expression level. We confirmed that the vectors indeed enhanced transfection efficiency of naked pMyD88, and in the subsequent experiments, non-specific pHK was used as the control. The real time PCR results showed that there was a significant decrease of MyD88 mRNA in the cells treated with pMyD88/liposomes complex. As depicted in Fig. 5(1), the pMyD88/liposomes complex group was at a much lower value of 0.11 ± 0.04, whereas the controls were about 1. The results indicated a significant inhibition of MyD88 gene transcription in the cells treated with the pMyD88/liposomes complex. Moreover, western blots revealed the evident suppressed expression of MyD88 protein in the pMyD88/liposomes complex group (0.11 ± 0.05), but not in the other groups as shown in Fig. 5(2). These results suggested that the pMyD88/liposomes complex worked well in DCs to obtain gene MyD88 silence.
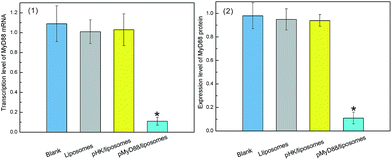 |
| Fig. 5 Gene expression of MyD88. (1) Transcription level of MyD88 mRNA. (2) Expression level of MyD88 protein. The data are the mean ± SE (n = 6). * Significantly lower than other groups (P < 0.01, ANOVA). | |
3.4 Investigation on immunogenicity of the gene silenced DCs
We expected that the DCs transfected by pMyD88/liposomes complex would fail in recognizing exogenous antigens. Lipopolysaccharide (LPS), a major cell wall constituent of Gram-negative bacteria, was used as external stimuli. The LPS stimulation test was carried out to mimic the initial contact of DCs with a foreign antigen, in which process TLRs recruit MyD88 to produce cytokines and induce immune response.
TLRs signaling recruited MyD88 as an adaptor and induced more MyD88 protein to be synthesized. Therefore, we first investigated the MyD88 gene expression of transfected DCs incubated with LPS. The real time PCR and western blot analysis showed that there was a significant suppressed expression of mRNA (Fig. 6(1)) in the cells treated with the pMyD88/liposomes complex as well as the MyD88 protein (Fig. 6(2)), whereas there was no difference in the other groups. Downstream effects of TLRs signaling confirmed the release of cytokines (IFN-Γ and IL-2) and the up-regulation of MHC II antigen on the cells surface. Cytokines and MHC II were associated with a subsequent immune response, such as T cells activation and proliferation. Therefore, we investigated the secretion of cytokines (IFN-Γ and IL-2) in culture media of transfected DCs. As shown in Fig. 6(3) and (4), ELISA results revealed that the cytokines secretion in pMyD88/liposomes complex group was remarkably suppressed. No difference occurred in the control groups. Finally, we tested the MHC II protein expressed on the cell surface. In Fig. 7, flow cytometry results showed a similar expression level of MHC II protein in pMyD88/liposomes complex group to that of the negative control. These results indicated that immune recognition via TLRs signaling was successfully blocked in DCs transfected by the pMyD88/liposomes complex.
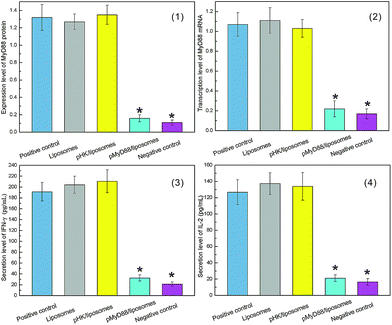 |
| Fig. 6 Expression of MyD88 protein and secretion level of cytokines in deficiency-MyD88 DCs treated with LPS. (1) Transcription level of MyD88 mRNA. (2) Expression level of MyD88 protein. (3) Secretion of IFN-γ. (4) Secretion of IL-2. The data are the mean ± SE (n = 6). * P < 0.01 as compared with cells in positive control, liposomes and pHK/liposomes groups (ANOVA). | |
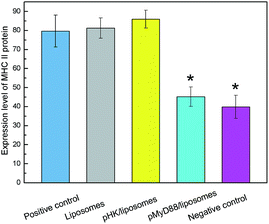 |
| Fig. 7 Expression of MHC II protein in deficiency-MyD88 DCs treated with LPS. The data are the mean ± SE (n = 6). * P < 0.01 as compared with cells in positive control, liposomes and pHK/liposomes groups (ANOVA). | |
4. Conclusions
In this study, we demonstrated that the pMyD88/liposomes complex obtained MyD88 gene silencing with high efficiency. Moreover, immune recognition via TLRs signaling was significantly inhibited in DCs transfected by the pMyD88/liposomes complex. AGE experiment was utilized to obtain the optimal mass ratio of 1
:
2 between plasmids and liposomes for the complex. The exciting transfection results revealed that the liposomes system significantly enhanced the transfection efficiency of naked pMyD88. Gene and protein detection proved that the plasmid/liposomes complex produced deficiency-MyD88 DCs with high efficiency. Furthermore, the LPS stimulation experiment confirmed that the deficiency of MyD88 protein blocked the signal transduction of immune recognition, resulting in the low immunogenicity of transfected DCs. In conclusion, our results indicated that the pMyD88/liposomes complex could be developed as an effective approach for inhibiting immune recognition. Moreover, the pMyD88/liposomes complex seems to have potential in solving skin graft rejection where TLRs signaling was predominant.
Acknowledgements
The authors gratefully acknowledge National Natural Science Foundation of China (81102246, 51373117, 51303126), Tianjin Natural Science Foundation (13JCQNJC11900), Tianjin Municipal Education Commission Science Foundation (20090126), Key Project of Tianjin Natural Science Foundation (13JCZDJC33200), and Doctoral Base Foundation of Educational Ministry of China (20120032110027).
Notes and references
- L. Chen, T. Wang, P. Zhou, L. Ma, D. Yin and J. Shen, et al., Am. J. Transplant., 2006, 6, 2282 CrossRef CAS PubMed
. - D. R. Goldstein, B. M. Tesar, S. Akira and F. G. Lakkis, J. Clin. Invest., 2003, 111, 1571 CrossRef CAS
. - D. McKay, A. Shigeoka, M. Rubinstein, C. Surh and J. Sprent, Eur. J. Immunol., 2006, 36, 1994 CrossRef CAS PubMed
. - D. Ducloux, M. Deschamps, M. Yannaraki, C. Ferrand, J. Bamoulid, P. Saas, A. Kazory, J. M. Chalopin and P. Tiberghien, Relevance of Toll-like receptor-4 polymorphisms in renal transplantation, Kidney Int., 2005, 67, 2454–2461 CrossRef CAS PubMed
. - S. S. Diebold, Immunol. Cell Biol., 2008, 86, 389 CrossRef CAS PubMed
. - J. S. Leventhal and B. Schroppel, Kidney Int., 2012, 81, 826 CrossRef CAS PubMed
. - S. Pandey and D. K. Agrawal, Immunol. Cell Biol., 2006, 84, 333 CrossRef CAS PubMed
. - H. Wu, G. A. Noordmans, M. R. O'Brien, J. Ma, C. Y. Zhao and G. Y. Zhang, et al., J. Am. Soc. Nephrol., 2012, 23, 1701 CrossRef CAS PubMed
. - U. Hasan, C. Chaffois, C. Gaillard, V. Saulnier, E. Merck and S. Tancredi, et al., J. Immunol., 2005, 174, 2942 CrossRef CAS
. - H. Kumar, T. Kawai and S. Akira, Biochem. Biophys. Res. Commun., 2009, 388, 621 CrossRef CAS PubMed
. - S. M. Palmer, W. Klimecki, L. Yu, N. L. Reinsmoen, L. D. Snyder and T. M. Ganous, et al., Am. J. Transplant., 2007, 7, 693 CrossRef CAS PubMed
. - K. Takeda, J. Endotoxin Res., 2005, 11, 51 CrossRef CAS PubMed
. - D. R. Goldstein, Transplant Immunol., 2006, 17, 11 CrossRef CAS PubMed
. - A. A. Shigeoka, T. D. Holscher, A. J. King, F. W. Hall, W. B. Kiosses and P. S. Tobias, et al., J. Immunol., 2007, 178, 6252 CrossRef CAS
. - W. E. Walker, I. W. Nasr, G. Camirand, B. M. Tesar, C. J. Booth and D. R. Goldstein, J. Immunol., 2006, 177, 5307 CrossRef CAS
. - E. Ingulli, Pediatr. Nephrol., 2010, 25, 61 CrossRef PubMed
. - K. Nakamura, A. S. Abu Lila, M. Matsunaga, Y. Doi, T. Ishida and H. Kiwada, Mol. Ther., 2011, 19, 2040 CrossRef CAS PubMed
. - S. Gonzalez, D. Castanotto, H. Li, S. Olivares, M. C. Jensen and S. J. Forman, et al., Mol. Ther., 2005, 11, 811 CrossRef CAS PubMed
. - E. J. Kim, H. J. Cho, D. Park, J. Y. Kim, Y. B. Kim and T. G. Park, et al., Mol. Ther., 2011, 19, 355–361 CrossRef CAS PubMed
. - Y. Zhang, A. Satterlee and L. Huang, Mol. Ther., 2012, 20, 1298 CrossRef CAS PubMed
. - H. Wang, P. Zhao, X. Liang, X. Gong, T. Song and R. Niu, et al., Biomaterials, 2010, 31, 4129 CrossRef CAS PubMed
. - H. Wang, P. Zhao, X. Liang, T. Song, X. Gong and R. Niu, et al., Biotechnol. Bioeng., 2010, 106, 952 CrossRef CAS PubMed
.
Footnotes |
† Electronic supplementary information (ESI) available. See DOI: 10.1039/c4ra12979b |
‡ Both authors contributed equally to this work. |
|
This journal is © The Royal Society of Chemistry 2015 |