DOI:
10.1039/C4RA07523D
(Paper)
RSC Adv., 2015,
5, 19041-19047
Sugar-functionalized water-soluble pillar[5]arene and its host–guest interaction with fullerene†
Received
24th July 2014
, Accepted 4th February 2015
First published on 5th February 2015
Abstract
Pillar[5]arenes have attracted much attention as a new member of macrocycles due to their unique symmetrical pillar architecture. Pillar[5]arenes are mostly soluble in organic solvents and slightly soluble in water, thus their application in aqueous media has been limited as a result. It is therefore necessary to design water-soluble pillar[5]arene derivatives. Herein, by the introduction of neutral sugar moieties at both upper and lower rims via click reaction, a neutral water-soluble pillar[5]arene (P5AG) was prepared and characterized well. Furthermore, the host–guest interaction between P5AG and fullerene was investigated in both organic medium and aqueous solution. The host–guest complexation was confirmed by fluorescence, nuclear magnetic resonance, ultraviolet-visible, and Raman spectroscopy.
Introduction
Since they were first reported as a new class of macrocyclic host in 2008,1 the pillarenes have attracted considerable attention owing to their unique structure and intriguing host–guest binding behavior. Compared with traditional macrocyclic hosts, pillarenes have a highly rigid and symmetrical structure. The pillar-like conformation facilitates selective binding toward various guest molecules. They are also easier to be functionalized in several ways.
Despite a great amount of work already dedicated to pillarenes, these pillar-shaped molecules continue to fascinate researchers in many different fields such as molecular recognition,2 sensing and detection,3 delivery systems,4 liquid crystals,5 and dispersion of carbon materials.6 Among all these situations, complexation behaviors of pillarenes towards different guest molecules have been extensively studied. With the electron-rich cavity, pillarenes are especially good at binding the electron-deficient guest molecules and some neutral molecules, such as viologen derivatives,7,8 (bis)-imidazolium cations,9 n-octyltrimethyl ammonium hexafluorophosphate,10 alkanediamines,11 and neutral bis(imidazole) derivatives.12 It is worth noting that the studies on host–guest properties of pillar[5]arenes are always carried out in organic media owing to their poor solubility in aqueous media. Therefore, it is necessary to design water-soluble pillar[5]arene derivatives. A water-soluble pillar[5]arene with ten negatively charged carboxylate groups functionalized at both rims was reported,7 and its binding behavior towards cationic viologen salts was studied in water. Subsequently, a pillar[5]arene decaamine was prepared and its encapsulation of linear diacids in neutral, alkaline, and acidic conditions were further investigated.13 Cationic pillar[5]arenes containing trimethylammonium groups were reported to strongly bind sodium 1-octanesulfonate in aqueous media, mainly driven by hydrophobic and electrostatic interactions.14
Fullerene, a condensed ring aromatic compound with extended π-conjugated systems, has cage-like structure and is 0.7 nm in diameter. Several studies have shown that fullerene has unique biological properties both in vitro and in vivo.15 Its applications have been gradually extended to various research fields, such as pharmaceutical science and biological field.15 However, the low solubility of fullerene in aqueous media significantly hampers its biological application. To overcome the natural repulsion of fullerenes and water, strategies including preparation of water-soluble supramolecular complexes with macrocyclic hosts such as cyclodextrin,16 calixarene derivatives,17 or cyclotriveratrylene (CTV) derivatives18 have been developed. These macrocycles can form complex with C60 in aqueous media. Water-soluble glycoconjugated CTV hosts prepared by introducing neutral sugar moieties into the CTV platform can bind C60 with good binding constants.18b,c The supramolecular chemistry of pillarene and fullerene is still rarely studied. Herein, by the introduction of neutral sugar moieties at both upper and lower rims via click reaction, a neutral water-soluble pillar[5]arene (P5AG) was prepared, and the host–guest interaction between P5AG and C60 was investigated in both aqueous solution and co-organic solvent.
Experimental section
Materials and characterization
All chemical reagents are commercially available and used as received unless otherwise stated. Pillar[5]arene derivative 1 (Scheme 1) and alkyne-functionalized sugar derivative 2 (Scheme 1) were prepared according to reported methods.7,13 Ultrapure water (18.2 MΩ cm) was purified with a Millipore purification system (Milli-Q water, Merck Millipore, Germany).
 |
| Scheme 1 Synthesis route to P5AG. | |
Proton nuclear magnetic resonance (1H NMR) and carbon nuclear magnetic resonance (13C NMR) spectra were acquired with a Bruker DMX400 spectrometer (Bruker, Germany). Mass spectra were measured using a Microflex LRF MALDI-TOF mass spectrometer (Bruker Daltonics, USA). Ultraviolet-visible (UV-vis) spectra were recorded with a Lambda 950 UV-vis-NIR spectrophotometer (Perkin-Elmer Instruments Co. Ltd., USA) and quartz cells with 1 cm path length. The fluorescence spectra were measured in a conventional cell with 1 cm path length using an LS55 spectrophotometer (Perkin-Elmer Instruments Co. Ltd., USA) at room temperature. Raman spectra were recorded with a Renishaw inVia Raman spectrometer (Renishaw, UK) by dropping the sample solutions onto a slide glass (after air drying) or putting C60 powder directly on a slide glass.
Synthesis of P5AG-OAc
The synthesis of P5AG-OAc is outlined in Scheme 1. To attach sugar groups via the click reaction, an intermediate azide substituted pillar[5]arene 1 was prepared from bromoethoxy group substituted pillar[5]arene through azidation. Then compound 1 (0.0455 g, 0.035 mmol) and glucose derivative 2 (0.1477 g, 0.367 mmol) were dissolved in DMF (1 mL) under nitrogen atmosphere. Ascorbic acid (6.93 mg, 0.036 mmol) and CuSO4 5H2O (4.37 mg, 0.018 mmol) were added to the mixture as catalyst. The reaction mixture was stirred overnight at 60 °C and then poured into pure water. The resulted precipitate was collected by filtration after washing with water for three times. After recrystallization in acetone and ether co-solvent, the pale yellow solid 3 was obtained (0.145 g, yield: 78%). 1H NMR (CDCl3) δ (ppm) 7.81 (s, 10H), 6.57 (d, J = 6.8 Hz, 10H), 5.24–5.18 (m, 10H), 5.12–5.03 (m, 20H), 4.72–4.63 (m, 30H), 4.26–3.90 (m, 50H), 3.72 (s, 10H), 3.27 (s, 10H), 3.12–3.38 (m, 10H), 2.04–1.97 (m, 120H). 13C NMR (CDCl3) 171.4, 170.8, 170.2, 150.3, 145.5, 129.5, 123.7, 116.8, 83.7, 83.6, 76.6, 74.6, 70.7, 69.1, 68.2, 66.6, 62.7, 59.1, 51.1, 31.7; MALDI-TOF-MS calcd. for C225H280N30O100S10, 5324.95 (m/z), found 5324.96. Melting point: 132.2–134.8 °C.
Synthesis of P5AG
A solution of sodium methoxide (1.0 M) in methanol was added to a solution of P5AG-OAc (60 mg, 0.011 mmol) in methanol–dichloromethane (2
:
1, 12 mL) drop-wise until the pH value reached to 11. The reaction mixture was stirred at room temperature for 6 h. After neutralization, the resulted suspension was filtered and washed with dichloromethane and petroleum ether for three times to give 4 (0.037 g, yield: 93%). 1H NMR (DMSO-d6) δ (ppm) 8.18 (s, 10H), 6.71 (s, 10H), 5.08–4.69 (m, 40H), 4.58–4.38 (m, 20H), 4.36–4.08 (m, 20H), 4.02 (d, J = 14.0 Hz, 10H), 3.91 (d, J = 13.6 Hz, 10H), 3.70 (d, J = 11.2 Hz, 20H), 3.17 (s, 20H), 3.14 (s, 20H), 3.09 (s, 20H). 13C NMR (DMSO-d6) δ (ppm) 154.7, 150.7, 134.4, 129.3, 90.3, 87.0, 84.1, 79.1, 76.1, 73.3, 67.3, 62.0, 60.9, 55.8, 54.6, 29.1. MALDI-TOF-MS calcd. for C145H200N30O60S10, 3643.95 (m/z), found 3643.97. Melting point: 148.5–150.9 °C.
Spectrofluorometric titration of P5AG−C60 complexation in toluene–DMSO
A solution of P5AG was prepared in toluene–DMSO (1
:
1, v/v). Aliquots of C60 in the same solvent were added to the solution. The final concentration of P5AG was 5.0 × 10−6 M, and the final concentration of C60 ranged from 0 to 25.0 × 10−6 M. After each addition, the sample was allowed to equilibrate for hours prior to recording a spectrum. Additions of C60 were continued until no significant change in the fluorescence signal was observed. The excitation wavelength was 280 nm and the emission scan ranged from 290 to 520 nm.
Preparation of P5AG−C60 complexes in aqueous solution
The aqueous solutions of sugar-bearing P5AG−C60 complexes were prepared by stirring an aqueous solution of P5AG (5.0 × 10−5 M) containing C60 solid (>99%) for days. After centrifugation, clear aqueous solutions containing sugar-bearing P5AG−C60 complexes were obtained for further studies.
Computational details
A molecular dynamics (MD) simulation of a 1
:
1 complex in a 60 × 60 × 60 Å3 periodic TIP3P water box was run by the NAMD code19 using CHARMM force field parameters.20–23 The details are included in the ESI.† Simulations were run for 50 nanoseconds (ns) with a two femtosecond timestep in the NPT ensemble at 298 K and 1.013 bar. The van der Waals interactions were cut off at 12 Å with a switching function starting at 10 Å.
Results and discussion
A sugar-bearing pillar[5]arene derivative was designed by introducing ten glucose moieties into the pillarene structure. Cu(I)-catalyzed azide “click” ligation between azido-substituted pillarene and alkyl-functionalized sugar derivative is chosen to introduce ten sugar groups into pillar[5]arene structure, on account of its highly efficient ligation and mild reaction condition. Formation of the triazole ring was confirmed by the chemical shift nearly at 7.81 ppm in the 1H NMR spectrum and the peaks nearly at 123.7 and 145.5 ppm in the 13C NMR spectrum of the corresponding desired intermediate P5AG-OAc. After removing all the acetyl groups with MeONa/MeOH, the desired water-soluble P5AG was obtained quantitatively and further confirmed by 1H NMR, 13C NMR, and mass spectrum. The disappearance of the peak of Ac (δ = 2.04 ppm) after final efficient global deprotection suggests the successful preparation of P5AG compared with 1H NMR spectrum of the protected precursor. The protected sugar-bearing pillar[5]arene is readily soluble in common organic solvents such as methylene chloride, chloroform, and tetrahydrofuran, but insoluble in methanol, ethanol, and water. The solubility of the resulting P5AG is different from its precursor, exhibiting a good solubility in DMF, DMSO, toluene–DMSO (1
:
1, v/v), and water.
The photophysical properties of the complexes generated from interaction of P5AG with C60 were investigated. P5AG possesses a distinct photoluminescence, an emission maximum peak at 323 nm in toluene–DMSO (1
:
1, v/v) solution appears when excited at 280 nm. The fluorescence in the region from 310 to 340 nm is probably due to the formation of ground-state dimer as a result of the interactions between the benzene rings upon excitation24 or excimeric emission caused by the interaction between the phenyl groups.25 Introducing the bulky sugar substituents by the click reaction not only inhibits the rotation of the units, but also extends arms of ten benzene rings thus making it possible to exhibit better host–guest behavior. The fluorescence may be ascribed to the enhanced planar conformation of benzene rings of pillar[5]arene derived from spatial effect of bulky branch chains.
The complexing properties of sugar-substituted P5AG with extended arms towards C60 were further verified by photoluminescence and Raman spectroscopy.26 The fluorescence spectroscopy is widely used as a tool to investigate the complexion between macrocycles and small guest molecules for its sensitivity, convenience, and cost-effectiveness. Both sugar-bearing P5AG and C60 are readily soluble in toluene–DMSO co-solvent (1
:
1, v/v) at low concentrations. Host–guest interaction between sugar-bearing P5AG and C60 was studied by spectrofluorometric titrations27,28 in the co-solvent at 25 °C. The emission spectrum from C60 solution is broad and between 650 and 725 nm in toluene.29 Furthermore, the fluorescence emission of highly purified samples of C60 at room temperature has been found to be very weak.30 The fluorescence emission of P5AG dissolved in toluene–DMSO (1
:
1, v/v) ranged from 310 to 340 nm, and no peak for C60 can be observed. Upon successive addition of the fullerene (0–25 μM) to the host solutions, a continuous fluorescence quenching was observed in the fluorescence spectra of sugar-bearing P5AG. The fluorescence intensity of P5AG gradually decreased and was significantly quenched when concentration of C60 was up to 10 μM (Fig. 1). Furthermore, fluorescence intensity of P5AG was finally quenched by 88% when concentration of C60 was up to 25.0 μM. The quenching might be attributed to the change in the extended cavity of the host with sugar moieties modified on ten arms of pillar[5]arene. Ground-state C60 possesses remarkable electron acceptor properties and is capable of accommodating as many as six electrons in solution.31 When sugar-bearing pillar[5]arene interacts with C60, there may exist photo-induced intermolecular electron transfer between sugar-bearing pillar[5]arene host and C60 thus leading to fluorescence quenching of sugar-bearing host. The extended spatial structure of P5AG with ten sugar moieties can accommodate C60 to some extent, so the photo-induced intermolecular electron transferring tends to take place. On the basis of linear fitting of the plot of [C60]−1 vs. ΔI and the hypothesis that the complex can be formed by host and C60 with 1
:
1 ratio at a low concentration, the calculated association constants Ka of host P5AG with C60 in toluene–DMSO solution was 1.75 × 105 M−1 at 298 K (R2 = 0.9991).
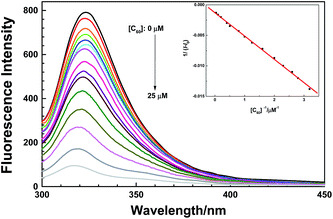 |
| Fig. 1 Fluorescence spectra of P5AG (5.0 × 10−6 M) in the absence and presence of various concentrations of C60 in the toluene–DMSO solution at room temperature (λex = 280 nm). The concentrations of C60 (×10−6 M) are 0, 0.313, 0.357, 0.384, 0.435, 0.500, 0.625, 0.800, 1.000, 1.250, 2.000, 2.500, 5.000, 10.000 and 25.000, respectively. The inset is the plot of ΔI vs. [C60]−1. | |
In order to further characterize and confirm the host–guest interaction between P5AG and C60 in toluene-d8–DMSO-d6 (1
:
1, v/v) co-solvent, 1H NMR spectroscopy (Fig. 2) was employed. The solubility of C60 in toluene is very limited at room temperature, reported by Ruoff.32 In order to maintain a proper proportion of host and guest and get well-dissolved mixture to characterize, we prepared the host solution with a concentration of 4.0 μM. Two peaks that appear at 7.01 and 7.11 ppm should be assigned to toluene-d8. As shown in Fig. 2, in the presence of about 1 equiv. of C60, the signals of P5AG's triazole proton (Ha) and part of sugar protons (Hb) exhibited a very slight upfield shift (Δδ < −0.1 ppm) compared to the free P5AG. As 2 equiv. of C60 were added, the corresponding protons of P5AG showed broadening effects and a bit larger upfield shift (Δδ = −0.1 ppm) compared to the free P5AG. Although only slight chemical shift changes were observed in the complexation systems probably owing to poor solubility of C60 in the solvent, the NMR experiments can give us some proof about interaction between P5AG host and C60.
 |
| Fig. 2 Partial 1H NMR spectra (400 MHz, toluene-d8–DMSO-d6 (1 : 1, v/v), 25 °C) of (a) P5AG, (b) P5AG + 1 equiv. C60, (c) P5AG + 2 equiv. C60 [P5AG] = 4.0 μM. | |
The host–guest interaction of sugar-bearing pillar[5]arene host and C60 was also investigated in aqueous solution. Formation of the complex facilitates the dispersion of fullerene in aqueous media. The water-soluble supramolecular complex between pillar[5]arene-based host and fullerenes was prepared simply by stirring an aqueous solution of P5AG (5.0 × 10−5 M) containing C60 solid (>99%) for days. Fig. 3 shows the UV-vis absorption spectra of C60, P5AG, and P5AG−C60 complex, respectively, in aqueous solution. C60 is insoluble in water as we known, therefore no absorption intensity was observed. However, after interacting with pillar[5]arene host for days, a new absorption peak at around 342 nm which assigned to characteristic signal peak of C60 in UV-vis region was observed, and its intensity gradually increased. The interaction of sugar-bearing P5AG hosts with C60 can also be visually monitored by a color change of the corresponding solutions. As shown in the inset of Fig. 3, the aqueous solution of P5AG is almost colorless, upon addition of C60, the color of aqueous P5AG−C60 solution gradually changes into yellow after stirring for days. The color changes of the solution suggest that P5AG can interact with C60 in aqueous solution and form a water-soluble complex. Although there is also possibility that the C60 aggregates might contribute to the peak of 342 nm,33 we did not observe any absorption of C60 in the control experiment in pure water.
 |
| Fig. 3 Absorption spectra of aqueous P5AG (a) and P5AG−C60 solutions (b–d) concentration of P5AG is 5.0 × 10−5 M), and C60 in water (e). Inset shows the photographs of aqueous P5AG and P5AG−C60 solutions corresponding to the traces ((a) P5AG; (b) P5AG+C60 70 h; (c) P5AG+C60 2 weeks; (d) P5AG+C60 3 weeks). | |
Equilateral pentagonal P5AG cavity with sugar–triazole cluster structure can not only provide an internal cavity capable of encapsulating the hydrophobic C60 guest considering the size-fit concept, but also prevent the aggregation in an aqueous solution owing to the fullerene–fullerene interaction. Multiple hydroxyl groups of sugar moieties make the supramolecular complexes water-soluble. The hydrophobic triazole groups and ten-membered ring skeleton of sugars can increase the hydrophobic and van der Waals interactions between the inner surface of sugar-bearing P5AG hosts and C60. These major driving forces are important in forming the supramolecular complexes. Further study with Raman spectra can also prove the existence of C60 in aqueous solution. The featured peak at 1470 cm−1 was assigned to the totally symmetric pentagonal pinch mode of C60 in P5AG−C60 sample, while no corresponding peak was found in P5AG (Fig. 4).34
 |
| Fig. 4 Raman spectra of P5AG−C60, P5AG and C60. | |
Molecular dynamics simulations of complex in water
Molecular dynamics simulations of a 1
:
1 complex were conducted to investigate possible binding conformations. During the first 35 nanoseconds (ns), C60 stays close to its initial position in front of the cavity opening of the pillarene. It moves somewhat back and forth but stays encapsulated by the sidechains, as shown in Fig. 5a. The sidechains are relatively mobile and alternate between interacting with the C60 molecule and being immersed in the water, but all of the time, three or four sidechains are in direct contact with the C60 and form a basket that keeps C60 in place in front of the cavity opening. Both the triazole and glucose moieties interact with C60, but it never moves so close to the pillarene cavity that is in direct contact with the ester oxygen and the two methylene groups that connect the central pillarene structure to the triazole ring. Shortly after 35 ns of simulation, C60 moves from this binding conformation to a new binding conformation on the side of the central pillarene structure, where it is in direct contact with one of the phenyl groups (Fig. 5b). This change in binding conformation takes less than 5 ns. Gradually, several sidechains from both rims of the pillarene start interacting with the C60, thereby forming a basket that restricts it to this binding conformation for the remaining simulation time (up to 50 ns). Two additional 25 ns simulations with different initial velocities support the observed binding conformations.
 |
| Fig. 5 Snapshot from MD simulation. (a) C60 moves around in front of the cavity opening of the pillarene. Three or four ones of the sidechains form a basket that keeps C60 in place. (b) C60 sticks to the side of the central pillarene structure and is in direct contact with the phenyl groups as well as the various parts of the sidechains. The atoms of the central pillarene structure are shown in black, oxygens are red, nitrogen is blue, carbons are cyan, and sulfur is yellow. Hydrogens are not shown. | |
The identification of these two distinct binding conformations opens up for the following consequences. First, when a single C60 binds P5AG, there is plenty of room for another, or maybe several other C60 molecules, to bind P5AG and therefore form higher-order complexes. The formation of higher-order complexes is of course concentration dependent, being favored by high concentrations of C60. Second, the C60 molecules are not surrounded by the host molecules and more than half of the C60 surface is still exposed to water. It does not seem unreasonable that these surfaces engage in hydrophobic interactions with other C60 or P5AG molecules, thereby forming extended structures in the form of clusters.
Conclusions
A sugar-functionalized water-soluble pillar[5]arene derivative (P5AG) modified with ten glucose moieties via click reaction has been successfully synthesized in a high yield. P5AG exhibits a distinct photoluminescence, thus can be used to conveniently detect their interaction with C60 through spectrofluorometric titration. P5AG can bind C60 to form supramolecular complexes in toluene–DMSO co-solvent. As a result of the extended arms on both rims of pillar[5]arene, the intrinsic cavity is enlarged. C60 can interact with host molecules owing to van der Waals force and hydrophobic interaction. The supramolecular complexation between P5AG and C60 was investigated in both organic solvent and aqueous solution, and also confirmed by fluorescence, 1H NMR, UV-vis, and Raman spectra. Molecular dynamics simulations of complexes reveal two distinct binding conformations in which C60 is located either in front of the cavity opening or at the side of the central pillarene structure. In both conformations the arms of the pillarene interact with C60 to form binding pockets. The association constant Ka of host P5AG with C60 was 1.75 × 105 M−1 in organic solvent, calculated on the hypothesis that supramolecular complex was formed with 1
:
1 molar ratio at 298 K. The host–guest interaction between sugar-bearing P5AG and C60 in aqueous solution will boost the potential biological application of C60.
Acknowledgements
The financial support of the National Science Foundation of China (Grant no. 21374024 and 61261130092), the Ministry of Science and Technology of China (Grant no. 2014CB932200 and 2011CB932500) is acknowledged. The authors also acknowledge the financial support from the Danish-Chinese Center for Molecular Nanoelectronics funded by the Danish National Research Foundation and the Danish Agency for Science, Technology and Innovation.
Notes and references
- T. Ogoshi, S. Kanai, S. Fujinami, T. A. Yamagishi and Y. Nakamoto, Para-bridged symmetrical pillar[5]arenes: their lewis acid catalyzed synthesis and host–guest property, J. Am. Chem. Soc., 2008, 130(15), 5022–5023 CrossRef CAS PubMed.
-
(a) C. Han, F. Ma, Z. Zhang, B. Xia, Y. Yu and F. Huang, DIBPillar[n]arenes (n = 5, 6): syntheses, X-ray crystal structures, and complexation with n-octyltriethyl ammonium hexafluorophosphate, Org. Lett., 2010, 12(19), 4360–4363 CrossRef CAS PubMed;
(b) G. Yu, C. Han, Z. Zhang, J. Chen, X. Yan, B. Zheng, S. Liu and F. Huang, Pillar[6]arene-based photoresponsive host–guest complexation, J. Am. Chem. Soc., 2012, 134(20), 8711–8717 CrossRef CAS PubMed;
(c) M. Xue, Y. Yang, X. Chi, Z. Zhang and F. Huang, Pillararenes, a new class of macrocycles for supramolecular chemistry, Acc. Chem. Res., 2012, 45(8), 1294–1308 CrossRef CAS PubMed;
(d) X. Shu, S. Chen, J. Li, Z. Chen, L. Weng, X. Jia and C. Li, Highly effective binding of neutral dinitriles by simple pillar[5]arenes, Chem. Commun., 2012, 48(24), 2967–2969 RSC;
(e) W. Chen, Y. Zhang, J. Li, X. Lou, Y. Yu, X. Jia and C. Li, Synthesis of a cationic water-soluble pillar[6]arene and its effective complexation towards naphthalenesulfonate guests, Chem. Commun., 2013, 49(72), 7956–7958 RSC.
-
(a) N. L. Strutt, R. S. Forgan, J. M. Spruell, Y. Y Botros and J. F. Stoddart, Monofunctionalized pillar[5]arene as a host for alkanediamines, J. Am. Chem. Soc., 2011, 133(15), 5668–5671 CrossRef CAS PubMed;
(b) T. Adiri, D. Marciano and Y. Cohen, Potential 129Xe-NMR biosensors based on secondary and tertiary complexes of a water-soluble pillar[5]arene derivative, Chem. Commun., 2013, 49(63), 7082–7084 RSC;
(c) S. Sun, X.-Y. Hu, D. Chen, J. Shi, Y. Dong, C. Lin, Y. Pan and L. Wang, Pillar[5]arene-based side-chain polypseudorotaxanes as an anion-responsive fluorescent sensor, Polym. Chem., 2013, 4(7), 2224–2229 RSC;
(d) S. Sun, J. Shi, Y. Dong, C. Lin, X.-Y. Hu and L. Wang, A pillar[5]arene-based side-chain pseudorotaxanes and polypseudorotaxanes as novel fluorescent sensors for the selective detection of halogen ions, Chin. Chem. Lett., 2013, 24(9), 987–992 CrossRef CAS PubMed;
(e) Y. Yao, X. Chi, Y. Zhou and F. Huang, A bola-type supra-amphiphile constructed from a water-soluble pillar[5]arene and a rod-coil molecule for dual fluorescent sensing, Chem. Sci., 2014, 5(7), 2778–2782 RSC;
(f) P. Wang, X. Yan and F. Huang, Host–guest complexation induced emission: a pillar[6]arene-based complex with intense fluorescence in dilute solution, Chem. Commun., 2014, 50(39), 5017–5019 RSC;
(g) R. R. Kothur, J. Hall, B. A. Patel, C. L. Leong, M. G. Boutellec and P. J. Cragg, A low pH sensor from an esterified pillar[5]arene, Chem. Commun., 2014, 50(7), 852–854 RSC.
-
(a) Q. Duan, Y. Cao, Y. Li, X. Hu, T. Xiao, C. Lin, Y. Pan and L. Wang, pH-responsive supramolecular vesicles based on water-soluble pillar[6]arene and ferrocene derivative for drug delivery, J. Am. Chem. Soc., 2013, 135(28), 10542–10549 CrossRef CAS PubMed;
(b) Y.-L. Sun, Y.-W. Yang, D.-X. Chen, G. Wang, Y. Zhou, C.-Y. Wang and J. F. Stoddart, Mechanized silica nanoparticles
based on pillar[5]arenes for on-command cargo release, Small, 2013, 9(19), 3224–3229 CAS.
-
(a) I. Nierengarten, S. Guerra, M. Holler, J. F. Nierengarten and R. Deschenaux, Building liquid crystals from the 5-fold symmetrical pillar[5]arene core, Chem. Commun., 2012, 48(65), 8072–8074 RSC;
(b) I. Nierengarten, S. Guerra, M. Holler, L. Karmazin-Brelot, J. Barberá, R. Deschenaux and J. F. Nierengarten, Macrocyclic effects in the meso morphic properties of liquid-crystalline pillar[5]- and pillar[6]arenes, Eur. J. Org. Chem., 2013,(18), 3675–3684 CrossRef CAS.
-
(a) G. Yu, M. Xue, Z. Zhang, J. Li, C. Han and F. Huang, A water-soluble pillar[6]arene: synthesis, host–guest chemistry, and its application in dispersion of multiwalled carbon nanotubes in water, J. Am. Chem. Soc., 2012, 134(32), 13248–13251 CrossRef CAS PubMed;
(b) J. Yang, G. Yu, D. Xia and F. Huang, A pillar[6]arene-based UV-responsive supra-amphiphile: synthesis, self-assembly, and application in dispersion of multiwalled carbon nanotubes in water, Chem. Commun., 2014, 50(30), 3993–3995 RSC.
- T. Ogoshi, M. Hashizume, T. A. Yamagishi and Y. Nakamoto, Synthesis, conformational and host–guest properties of water-Soluble pillar[5]arene, Chem. Commun., 2010, 46(21), 3708–3710 RSC.
- T. Ogoshi, D. Yamafuji, T. Aoki and T. A. Yamagishi, Photoreversible transformation between seconds and hours time-scale: threading of pillar[5]arene onto the azobenzene-end of a viologen derivative, J. Org. Chem., 2011, 76(22), 9497–9503 CrossRef CAS PubMed.
- C. Li, L. Zhao, J. Li, X. Ding, S. Chen, Q. Zhang, Y. Yu and X. Jia, Self-assembly of [2]pseudorotaxanes based on pillar[5]arene and bis(imidazolium) cations, Chem. Commun., 2010, 46(47), 9016–9018 RSC.
- Z. Zhang, Y. Luo, B. Xia, C. Han, Y. Yu, X. Chen and F. Huang, Four constitutional isomers of BMpillar[5]arene: synthesis, crystal structures and complexation with N-octyltrimethyl ammonium hexafluorophosphate, Chem. Commun., 2011, 47(8), 2417–2419 RSC.
- N. L. Strutt, R. S. Forgan, J. M. Spruell, Y. Y. Botros and J. F. Stoddart, Monofunctionalized pillar[5]arene as a host for alkanediamines, J. Am. Chem. Soc., 2011, 133(15), 5668–5671 CrossRef CAS PubMed.
- C. Li, S. Chen, J. Li, K. Han, M. Xu, B. Hu, Y. Yu and X. Jia, Novel neutral guest recognition and interpenetrated complex formation from pillar[5]arenes, Chem. Commun., 2011, 47(40), 11294–11296 RSC.
- X.-B. Hu, L. Chen, W. Si, Y. Yu and J.-L. Hou, Pillar[5]arene decaamine: synthesis, encapsulation of very long linear diacids and formation of ion pair-stopped [2]rotaxanes, Chem. Commun., 2011, 47(16), 4694–4696 RSC.
- Y. Ma, X. Ji, F. Xiang, X. Chi, C. Han, J. He, Z. Abliz, W. Chen and F. Huang, Cationic water-soluble pillar[5]arene: synthesis and host–guest complexation with sodium 1-octanesulfonate, Chem. Commun., 2011, 47(45), 12340–12342 RSC.
- M. Prato and T. D. Ros, Medicinal chemistry with fullerenes and fullerene derivatives, Chem. Commun., 1999,(8), 663–669 Search PubMed.
-
(a) C. N. Murthy and K. E. Geckeler, The water-soluble β-cyclodextrin–[60]fullerene complex, Chem. Commun., 2001,(13), 1194–1195 RSC;
(b) S. Takekuma, H. Takekuma, T. Matsumoto and Z. Yoshida, A highly efficient generation of cyclodextrin-bicapped C60 in aqueous solution, Tetrahedron Lett., 2000, 41(25), 4909–4912 CrossRef CAS.
- A. Ikeda, T. Hatano, M. Kawaguchi, H. Suenaga and S. Shinkai, Water-soluble [60]fullerene–cationic homooxacalix[3]arene complex which is applicable to the photocleavage of DNA, Chem. Commun., 1999,(15), 1403–1404 RSC.
-
(a) Y. Rio and J. F. Nierengarten, Water soluble supramolecular cyclotriveratrylene–[60] fullerene complexes with potential for biological applications, Tetrahedron Lett., 2002, 43(24), 4321–4324 CrossRef CAS;
(b) F. Yang, Q. Chen, Q.-Y. Cheng, C.-G. Yan and B.-H. Han, Sugar-functionalized water-soluble cyclotriveratrylene derivatives: preparation and their interaction with fullerene, J. Org. Chem., 2012, 77(2), 971–976 CrossRef CAS PubMed;
(c) L.-J. Feng, H. Li, Q. Chen and B.-H. Han, Cationic cyclotriveratrylene-based glycoconjugate and its interaction with fullerene, RSC Adv., 2013, 3(19), 6985–6990 RSC.
- J. C. Phillips, R. Braun, W. Wang, J. Gumbart, E. Tajkhorshid, E. Villa, C. Chipot, R. D. Skeel, L. Kalé and K. Schulten, Scalable molecular dynamics with NAMD, J. Comput. Chem., 2005, 26(16), 1781–1802 CrossRef CAS PubMed.
- K. Vanommeslaeghe, E. Hatcher, C. Acharya, S. Kundu, S. Zhong, J. Shim, E. Darian, O. Guvench, P. Lopes, I. Vorobyov and A. D. Mackerell, CHARMM general force field: A force field for drug-like molecules compatible with the CHARMM all-atom additive biological force fields, J. Comput. Chem., 2010, 31(4), 671–690 CAS.
- K. Vanommeslaeghe and A. D. MacKerell, Automation of the CHARMM general force field (CGenFF) I: bond perception and atom typing, J. Chem. Inf. Model., 2012, 52(12), 3144–3154 CrossRef CAS PubMed.
- K. Vanommeslaeghe, E. P. Raman and A. D. MacKerell, Automation of the CHARMM general force field (CGenFF) II: assignment of bonded parameters and partial atomic charges, J. Chem. Inf. Model., 2012, 52(12), 3155–3168 CrossRef CAS PubMed.
- O. Guvench, S. N. Greene, G. Kamath, J. W. Brady, R. M. Venable, R. W. Pastor and A. D. Mackerell, Additive empirical force field for hexopyranose monosaccharides, J. Comput. Chem., 2008, 29(15), 2543–2564 CrossRef CAS PubMed.
- H. Itagaki, Y. Inagaki and N. Kobayashi, Microenvironments in poly(ethylene terephthalate) film revealed by means of fluorescence measurements, Polymer, 1996, 37(16), 3553–3558 CrossRef CAS.
- C. W. Frank, D. J. Henker and J. W. Thomas, Photophysical studies of amorphous orientation in poly(ethylene terephthalate) films, Polymer, 1988, 29(3), 437–447 CrossRef.
- J. L. Atwood, J. W. Steed, P. C. Junk, C. L. Raston, M. J. Barnes and R. S. Burkhalter, Ball and socket nanostructures: new supramolecular chemistry based on cyclotriveratrylene, J. Am. Chem. Soc., 1994, 116(22), 10346–10347 CrossRef.
- Y. Liu, B.-H. Han, S.-X. Sun, T. Wada and Y. Inoue, Molecular recognition study on supramolecular systems. 20. Molecular recognition and enantioselectivity of aliphatic alcohols by tryptophan-modified β-cyclodextrin, J. Org. Chem., 1999, 64(5), 1487–1493 CrossRef CAS PubMed.
- Y. Liu, B.-H. Han and Y.-T. Chen, Molecular recognition and complexation thermodynamics of dye guest molecules by modified cyclodextrins and calixarenesuffonates, J. Phys. Chem. B, 2002, 106(18), 4678–4687 CrossRef CAS.
- D. Kim and M. Lee, Observation of fluorescence emission from solutions of C60 and C70 and measurement of their excited-state lifetimes, J. Am. Chem. Soc., 1992, 114(11), 4429–4430 CrossRef CAS.
- D. J. van den Heuvel, G. J. B. van den Berg, E. J. J. Groenen and J. Schmidt, Lowest excited singlet state of C60: a vibronic analysis of the fluorescence, J. Phys. Chem., 1995, 99(30), 11644–11649 CrossRef CAS.
- D. M. Guldi, M. Maggini, G. Scorrano and M. Prato, Intramolecular electron transfer in fullerene/ferrocene based donor-bridge-acceptor dyads, J. Am. Chem. Soc., 1997, 119(5), 974–980 CrossRef CAS.
- R. S. Ruoff, R. Malhotra, D. L. Huestis, D. S. Tse and D. C. Lorents, Anomalous solubility behavior of fullerene C60, Nature, 1993, 362(6416), 140–141 CrossRef CAS.
- A. D. Bokare and A. Patnaik, C60 aggregate structure and geometry in nonpolar o-xylene, J. Phys. Chem. B, 2005, 109(1), 87–92 CrossRef CAS PubMed.
- M. Moskovits, K. L. Akers, C. Douketis and T. L. Haslett, Raman spectroscopy of C60 solid films: a tale of two spectra, J. Phys. Chem., 1994, 98(42), 10824–10831 CrossRef.
Footnote |
† Electronic supplementary information (ESI) available: Computational details; 1H NMR, 13C NMR and MS spectra of the key compounds. See DOI: 10.1039/c4ra07523d |
|
This journal is © The Royal Society of Chemistry 2015 |
Click here to see how this site uses Cookies. View our privacy policy here.