DOI:
10.1039/C5QI00134J
(Research Article)
Inorg. Chem. Front., 2015,
2, 927-930
A bis(1H-pyridin-2-one)salen Eu(III) complex for vapoluminescence sensing†
Received
28th July 2015
, Accepted 28th August 2015
First published on 31st August 2015
Abstract
A bis(1H-pyridin-2-one)salen Eu(III) complex shows strong sensitized luminescence with a quantum yield of 28% and vapoluminescence sensing of acid–base vapour on a fabricated device.
Luminescent lanthanide complexes have received considerable attention because of their highly desirable applications such as lighting, displays, sensing, and biomedical imaging.1 Due to the low absorption coefficients of trivalent lanthanide ions, organic ligands with chromophoric groups are required to populate the lanthanide emitting states through energy transfer, which is known as sensitization. In the past few decades, a large variety of ligands (e.g., phosphine oxides,2 β-diketonates,3 dipicolinates,4 salen derivatives,5 cyclen derivatives,6 and cryptands7) have been developed to construct emissive lanthanide complexes especially Eu(III) complexes (featuring saturated red emission), some of which showed chemical sensing.6b,c,7c Although these excellent sensing performances in aqueous solution are of great significance, we believe that the exploitation of lanthanide complexes for gas sensing would be of increased necessity, because most lanthanide complexes possess excellent solubility in methanol and ethanol, which facilitates the fabrication of thin film devices.
Vapoluminescence is a luminescence vapochromic phenomenon that is very promising for the detection of harmful chemical gases.8 Recently, there has been increasing interest in acid–base gas-responsive luminescent materials because of their application not only as sensors but also as display devices.9 However, structurally characterized materials remain rare. Through design of a bis(1H-pyridin-2-one)salen ligand, 3,3′-[ethane-1,2-diylbis(azanylylidene)bis(methanylylidene)]bis(1H-pyridin-2-one) (H2L, Fig. 1a), here we report a mononuclear Eu(III) complex and its luminescence switching triggered by acid–base vapour. Previously, the O donors of the N2O2 salen ligands were invariably the phenol hydroxyl oxygen atom.5,10 This is the first time the ketone carbonyl oxygen atom as a salen O donor has been employed, which may provide lanthanide complexes with novel optical properties.11
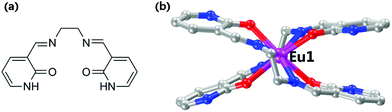 |
| Fig. 1 (a) The H2L ligand. (b) The mononuclear structure of 1. | |
The solvothermal reaction of H2L with EuCl3·6H2O in a butanol solution at 75 °C results in the formation of colourless polyhedral crystals of [Eu(H2L)2·(H2O)2Cl3] (1). Single-crystal X-ray analysis of complex 1 at 80 K reveals that it crystallizes in the monoclinic space group Cc. The asymmetric unit contains only one crystallographically independent Eu(III) ion. The Eu(III) ion is eight-coordinate and adopts D2d 8,18,12-dodecahedron geometry (Fig. S4†).12 The sites in the axial direction are covered by two sets of chelating NN donor units in a criss-cross fashion [Eu–N in the range of 2.524–2.625 Å], while the sites at the equator are occupied by four ketone carbonyl oxygen atoms [Eu–O in the range of 2.296–2.342 Å]. Thanks to the kink in the ethylenediimine spacer, two H2L ligands can clasp each other around one Eu(III) ion, thus forming a mononuclear structure (Fig. 1b). It should be noted that the ligands (H2L) retain the neutral keto form in the complex. The valence requirements of the mononuclear complex are satisfied by the presence of three uncoordinated chloride ions. Each complex molecule is associated with the others through the hydrogen bonding of all N–H donors to the chlorine and water medium (Fig. S5†). Three different kinds of hydrogen bonding interactions of N⋯O, N⋯Cl, and O⋯Cl fall in the range of 2.65–2.68 Å, 3.05–3.10 Å, and 2.74–3.10 Å respectively.
Fig. 2 presents the reflectance spectra of the H2L ligand and complex 1. The absorption band of the complex shows a little red shift compared to that of the ligand, but both contain three peaks in regard to n → σ*, π → π*, and n → π* transitions. The π → π* and n → π* absorption peaks of complex 1 are located at 332 and 381 nm, respectively. The appearance of the n → σ* transition at 247 nm reveals the unique electronic property of the bis(1H-pyridin-2-one)salen ligand. The excitation spectrum of complex 1 exhibits one broad band peaking at 367 nm (Fig. 3a), corresponding to the mixed π → π* and n → π* transitions of the H2L ligand. This suggests the particularly efficient sensitization of the Eu(III) ion by the bis(1H-pyridin-2-one)salen ligand.
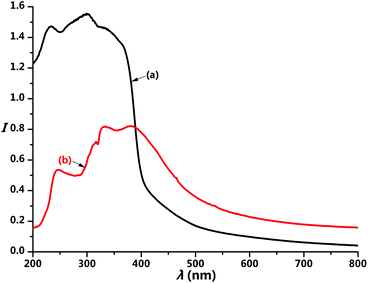 |
| Fig. 2 Reflectance spectra of (a) the H2L ligand and (b) complex 1. | |
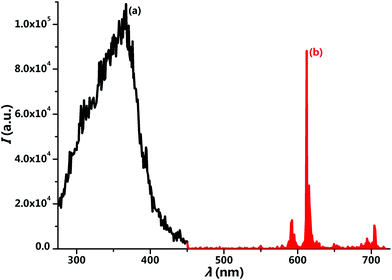 |
| Fig. 3 Solid-state excitation (a) and emission (b) spectra of complex 1. | |
After excitation at 367 nm, complex 1 exhibits the characteristic emission of the Eu(III) ion (Fig. 3b). The sharp emission lines are attributed to the f–f transitions of 5D0 → 7FJ (J = 0–4), where the 5D0 → 7F2 transition at 613 nm dominates the spectrum. The luminescence decay curve of complex 1 fits a single-exponential function with a lifetime of 813 ± 1 μs upon monitoring the 5D0 → 7F2 emission line, in agreement with the single Eu(III) site. The quantum yield was measured to be 28% by an absolute integrating-sphere method.
The bis(1H-pyridin-2-one)salen ligand has poor solubility in ordinary organic solvents, but it becomes a good solute in methanol and ethanol after complexation of the Eu(III) ion. The deposition from a methanol solution (10 μM) of complex 1 onto a glass substrate by the spin-coating method leads to a transparent and homogeneous thin film. Upon excitation at 365 nm, interestingly, the photoluminescence of the film could be switched between green and red by exposure to acid–base vapour (Fig. 4). When the red-emissive film was exposed to hydrogen chloride (HCl) vapour, it changed into green emission immediately. After subsequent exposure to triethylamine (Et3N) vapour, the red emission was recovered in ten seconds. As can be seen in Fig. 5 and S6,† the red emission peak at 613 nm corresponds to the sensitized Eu(III) emission, and the green emission band around 467 nm is from the ligand. The acid–base vapour-induced luminescence switching is ascribed to the dissociation of the complex structure by protonating the imine nitrogen and ketone carbonyl oxygen atoms in an acidic gas environment and the recomplexation of the Eu(III) ion by neutralizing the proton in a basic gas environment.
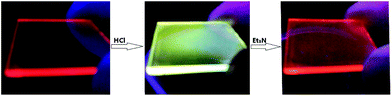 |
| Fig. 4 Photographs of film during the exposure to HCl–Et3N vapour. | |
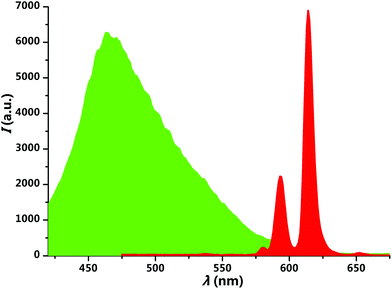 |
| Fig. 5 Spectral changes before and after exposure to HCl–Et3N vapour. Original Eu(III) complex (red area), after exposure to HCl vapor (green area). | |
To examine the reversibility and response time, we treated the film alternately with HCl gas and Et3N vapour. After eight HCl–Et3N cycles, the film can still return to the strong red emission (Fig. 6), but with growing response times no more than half a minute. These results demonstrate that complex 1 is an excellent substrate for acid–base gas sensing. The slower response may be due to the increased disorder of molecular arrangement and the gradual accumulation of triethylamine hydrochloride in film during the cycles.
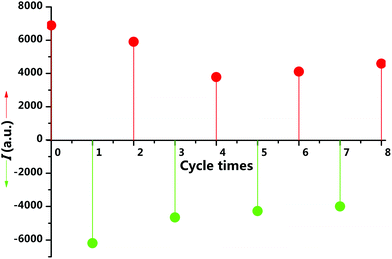 |
| Fig. 6 Response of luminescence intensities at 466 nm (green circles) and 613 nm (red circles) of the film during HCl–Et3N vapour exposure cycles. | |
Conclusions
In summary, we designed the first bis(1H-pyridin-2-one)salen ligand, and succeeded in obtaining a mononuclear Eu(III) complex. The photophysical studies show the particularly efficient sensitization of the Eu(III) ion by the bis(1H-pyridin-2-one)salen ligand. The thin film device fabricated by the Eu(III) complex possesses excellent vapoluminescence sensing performance towards acid–base vapour.
Experimental
Synthesis of complex 1
H2L (27 mg, 0.1 mmol), EuCl3·6H2O (20 mg, 0.055 mmol), and n-BuOH solvent (2 mL) were placed in a 20 mL vial. After three minutes of ultrasound treatment, the sealed sample was heated at 75 °C for 1 day, and then cooled to room temperature. The resulting colourless crystals were filtered off and dried in air. Yield (based on H2L): 84%. IR (KBr, cm−1): 3336 (w), 3082 (w), 2910 (w), 2853 (w), 2782 (w), 2738 (w), 1653 (vs), 1595 (vs), 1548 (s), 1465 (s), 1423 (s), 1344 (s), 1226 (s), 1115 (w), 1060 (m), 1029 (w), 927 (m), 898 (w), 774 (s), 650 (m), 599 (s), 526 (m), 450 (w). Elemental analysis (%): calcd for C28H30Cl3EuN8O6 (832.92): C 40.34, H 3.83, N 13.41; found: C 40.20, H 3.75, N 13.64.
Crystallographic analysis
Single-crystal X-ray diffraction data of complex 1 were collected on an Agilent Technologies Gemini A System (Cu Kα, λ = 1.54178 Å) at 80 K. The data were processed using CrysAlis. The structure was solved by direct methods and refined by full-matrix least-squares cycles on F2. Crystal data for complex 1: C28H30Cl3EuN8O6, M = 832.92, monoclinic, space group Cc, a = 15.4368(13), b = 18.1268(7), c = 13.4833(8) Å, α = 90°, β = 119.572(8)°, γ = 90°, V = 3281.4(4) Å3, Z = 4, Dc = 1.686 g cm−3. The final refinement converges to final R = 0.0601 and wR = 0.1437 with 6399 parameters from 10
667 independent reflections (I > 2σ(I)). The goodness of fit on F2 was 1.022. CCDC reference number 1414545.
Acknowledgements
The authors acknowledge financial support from the National Natural Science Foundation of China (NSFC) (grant number 21431002) and the Specialized Research Fund for the Doctoral Program of Higher Education (grant number 20110211130002).
Notes and references
-
(a) J.-C. G. Bünzli and C. Piguet, Chem. Soc. Rev., 2005, 34, 1048 RSC;
(b) S. V. Eliseeva and J.-C. G. Bünzli, Chem. Soc. Rev., 2010, 39, 189 RSC.
-
(a) K. Miyata, T. Nakagawa, R. Kawakami, Y. Kita, K. Sugimoto, T. Nakashima, T. Harada, T. Kawai and Y. Hasegawa, Chem. – Eur. J., 2011, 17, 521 CrossRef CAS PubMed;
(b) Y. Kuramochi, T. Nakagawa, T. Yokoo, J. Yuasa, T. Kawaia and Y. Hasegawa, Dalton Trans., 2012, 41, 6634 RSC;
(c) K. Miyata1, Y. Konno, T. Nakanishi1, A. Kobayashi, M. Kato, K. Fushimi and Y. Hasegawa, Angew. Chem., Int. Ed., 2013, 52, 6413 CrossRef PubMed.
-
(a) A. P. Bassett, S. W. Magennis, P. B. Glover, D. J. Lewis, N. Spencer, S. Parsons, R. M. Williams, L. D. Cola and Z. Pikramenou, J. Am. Chem. Soc., 2004, 126, 9413 CrossRef CAS PubMed;
(b) L. F. Smith, B. A. Blight, H.-J. Park and S. Wang, Inorg. Chem., 2014, 53, 8036 CrossRef CAS PubMed;
(c) H. Xin, F.-Y. Li, M. Shi, Z.-Q. Bian and C.-H. Huang, J. Am. Chem. Soc., 2003, 125, 7166 CrossRef CAS PubMed;
(d) M. Shi, F.-Y. Li, T. Yi, D.-Q. Zhang, H.-M. Hu and C.-H. Huang, Inorg. Chem., 2005, 44, 8929 CrossRef CAS PubMed.
-
(a) A.-S. Chauvin, F. Gumy, D. Imbert and J.-C. G. Bünzli, Spectrosc. Lett., 2007, 40, 193 CrossRef CAS PubMed;
(b) M. R. George, C. A. Golden, M. C. Grossel and R. J. Curry, Inorg. Chem., 2006, 45, 1739 CrossRef CAS PubMed;
(c) H.-J. Park, S.-B. Ko, I. W. Wyman and S. Wang, Inorg. Chem., 2014, 53, 9751 CrossRef CAS PubMed.
- X.-P. Yang, R. A. Jones and S.-M. Huang, Coord. Chem. Rev., 2014, 273, 63 CrossRef PubMed.
-
(a) M. Tropiano, N. L. Kilah, M. Morten, H. Rahman, J. J. Davis, P. D. Beer and S. Faulkner, J. Am. Chem. Soc., 2011, 133, 11847 CrossRef CAS PubMed;
(b) J. D. Moore, R. L. Lord, G. A. Cisneros and M. J. Allen, J. Am. Chem. Soc., 2012, 134, 17372 CrossRef CAS PubMed;
(c) M. Tropiano and S. Faulkner, Chem. Commun., 2014, 50, 4696 RSC.
-
(a) B. Alpha, J.-M. Lehn and G. Mathis, Angew. Chem., Int. Ed. Engl., 1987, 26, 266 CrossRef PubMed;
(b) B. Alpha, V. Balzani1, J.-M. Lehn, S. Perathoner and N. Sabbatini, Angew. Chem., Int. Ed. Engl., 1987, 26, 1266 CrossRef PubMed;
(c) C. Bazzicalupi, A. Bencini, A. Bianchi, C. Giorgi, V. Fusi, A. Masotti, B. Valtancoli, A. Roque and F. Pina, Chem. Commun., 2000, 561 RSC.
-
(a) O. S. Wenger, Chem. Rev., 2013, 113, 3686 CrossRef CAS PubMed;
(b) X. Zhang, B. Li, Z.-H. Chen and Z.-N. Chen, J. Mater. Chem., 2012, 22, 11427 RSC.
-
(a) Y.-S. Zhao, J.-S. Wu and J.-X. Huang, J. Am. Chem. Soc., 2009, 131, 3158 CrossRef CAS PubMed;
(b) C.-D. Dou, L. Han, S.-S. Zhao, H.-Y. Zhang and Y. Wang, J. Phys. Chem. Lett., 2011, 2, 666 CrossRef CAS;
(c) F. Nastasi, F. Puntoriero, N. Palmeri, S. Cavallaro, S. Campagna and S. Lanza, Chem. Commun., 2007, 4740 RSC;
(d) K. Li, Y. Chen, W. Lu, N.-Y. Zhu and C.-M. Che, Chem. – Eur. J., 2011, 17, 4109 CrossRef CAS PubMed;
(e) T. Sato and M. Higuchi, Chem. Commun., 2012, 48, 4947 RSC;
(f) J. Xu, L. Jia, N.-Z. Jin, Y.-F. Ma, X. Liu, W.-Y. Wu, W.-S. Liu, Y. Tang and F. Zhou, Chem. – Eur. J., 2013, 19, 4556 CrossRef CAS PubMed.
- C. J. Whiteoak, G. Salassa and A. W. Kleij, Chem. Soc. Rev., 2012, 41, 622 RSC.
-
(a) B.-B. Du, Y.-X. Zhu, M. Pan, M.-Q. Yue, Y.-J. Hou, K. Wu, L.-Y. Zhang, L. Chen, S.-Y. Yin, Y.-N. Fan and C.-Y. Su, Chem. Commun., 2015, 51, 12533 RSC;
(b) Q.-Y. Yang, K. Wu, J.-J. Jiang, C.-W. Hsu, M. Pan, J.-M. Lehna and C.-Y. Su, Chem. Commun., 2014, 50, 7702 RSC;
(c) Y.-B. Shu, X.-L. Tang and W.-S. Liu, Inorg. Chem. Front., 2014, 1, 226 RSC.
- R. B. King, J. Am. Chem. Soc., 1969, 91, 7211 CrossRef CAS.
Footnote |
† Electronic supplementary information (ESI) available: Materials and instruments, IR spectrum, powder XRD pattern, luminescence decay profile, emission spectrum of the protonated free ligand, and additional diagrams. CCDC 1414545. For ESI and crystallographic data in CIF or other electronic format see DOI: 10.1039/c5qi00134j |
|
This journal is © the Partner Organisations 2015 |