DOI:
10.1039/C5NJ01664A
(Paper)
New J. Chem., 2015,
39, 8337-8341
A BODIPY/pyridine conjugate for reversible fluorescence detection of gold(III) ions†
Received
(in Nottingham, UK)
27th June 2015
, Accepted 10th August 2015
First published on 13th August 2015
Abstract
We designed a “turn-on” type fluorescent probe based on a BODIPY–pyridine conjugate which exhibits high selectivity towards Au(III) ions and, also responds to changes in the pH within the acidic pH range. The probe offers features such as a rapid response time, a low detection limit, and high sensitivity and selectivity. The detection of Au(III) is recognized by a distinct change in the emission intensity which relies on a reversible “ligand to ion” binding mechanism. We also document the utility of the probe for the quantification of gold ion residues in synthetic end products prepared via gold catalysis.
Introduction
During the last decade, the catalytic properties of gold have attracted a surge of attention in the field of synthetic chemistry. Ionic species of gold, in contrary to elemental gold, have the exceptional capability to activate unsaturated bonds (e.g. alkyne) towards the addition of nucleophiles.1–5 Besides their peerless catalytic activities, gold ions present interesting biological properties. For a long time, certain drugs based on various gold ion complexes have been used in the treatment of diseases including rheumatic arthritis, cancer, asthma, and HIV.6–8 In contrast to their beneficial roles in disease treatment, the intake of gold ions can be toxic to living organisms because of the possibility of the interaction with biomolecules such as enzymes and DNA. Numerous scientific studies have established the detrimental effects of gold species on vital human organs. For instance, the intake of AuCl3 causes damage to the kidneys, liver, and the peripheral nervous system.9–11
The potential health hazards associated with gold species pushes the demand for developing trustable and efficient methods to detect trace levels of gold species existing in synthetic chemicals prepared via gold catalysis.
Trace metal analysis relying on fluorescence techniques are very popular nowadays.12–16 In contrast to the traditional instrumental techniques, fluorescence-based techniques have many preferable advantages such as low cost, simplicity, high sensitivity and reproducibility. Several gold ion selective molecular sensors utilizing various fluorophore units – including rhodamine,17–21 BODIPY,22–25 fluorescein,26,27 naphthalimide,28,29 and coumarin30 dyes – have been developed over the last few years.31,32 The great majority of the sensors present in the literature are based on irreversible chemical events, which take advantage of the alkynophilic behaviour of gold species. In general, a fluorophore core integrated with a gold ion specific reactive unit (i.e. alkyne), will transform the action of the gold ions into a fluorescence signal output either through a change in the fluorescence wavelength or a change in the fluorescence intensity.33 Notably, one chronic issue in reaction-based gold ion sensing is the potential of other alkynophilic metal species to interfere with the detection of the gold ions. Thus, new sensing strategies utilizing alternative recognition events need to be developed in order to improve the general shortcomings of reaction based sensing strategies.
In this regard, molecular sensors relying on reversible ion–dipole interactions appear to be good alternatives to reaction-based molecular sensors. Interestingly, however, molecular sensors which operate reversibly towards gold ions are extremely scarce, most predominantly due to the challenges of design and synthesis.34
Herein, we present the design, synthesis and spectral properties of BOD-Pyr, a novel turn-on type fluorescent chemosensor that allows both Au3+ and H3O+ ions to be detected on the basis of reversible ion–dipole interactions. Knowing the capability of pyridine to act as a ligand for ionic gold species, we envisioned that a pyridine motif, when integrated with a fluorophore core, could act as a specific recognition motif for gold ions. Several BODIPY–pyridine conjugates have been reported in the literature which respond to changes in pH or to certain metal species such as Cu2+ and Hg2+.35–37
With all this in mind, we designed a molecular structure, which comprises a BODIPY dye as the fluorophore unit and a pyridylethenyl motif as the recognition site. The BODIPY core was the fluorophore of choice owing to its exceptional photophysical properties such as high photo stability, high fluorescence quantum yield, robustness towards light and chemicals, and long emission/absorption wavelengths.38–41
Results and discussion
BOD-Pyr was prepared by the synthetic route outlined in Scheme 1. First, a Vilsmeier Haack's formylation reaction of BODIPY-1 gave BODIPY-2,42 which in the final step was treated with an appropriate Wittig reagent to give exclusively the E-isomer of the title compound in a reasonable yield of ca. 56% (Scheme 1). The chemical structure of BOD-Pyr was clearly confirmed by performing NMR spectroscopy and HRMS analysis.
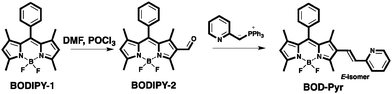 |
| Scheme 1 Synthetic route for BOD-Pyr. | |
Au3+ sensing properties of BOD-Pyr
The photophysical behaviour of BOD-Pyr in response to a series of metal species was carried out by both UV-vis absorption and fluorescence spectroscopy. We commenced our investigation by first determining the optimum conditions for the recognition of Au3+ ions. A variety of solvent combinations involving EtOH–H2O, DMF–H2O, and CH3CN–H2O (Fig. S1, ESI†) were screened. Furthermore, the ratio of water in the semi-aqueous environment and the effect of the pH on the sensing process were carefully investigated. Eventually, the optimum conditions for the sensing process were established as a 0.1 M phosphate buffer/EtOH (pH = 7.0, v/v, 1
:
1) with a 10 μM dye concentration (Fig. S2 and S3, ESI†).
The spectral changes of BOD-Pyr in the absence and presence of Au3+ ions are displayed in Fig. 1. As shown, free BOD-Pyr exhibits a faint fluorescence emission centered at 591 nm. (ΦF = 0.044). However, the addition of Au3+ (6 equiv.) to BOD-Pyr results in an observable enhancement of the emission intensity together with a slight blue shift in the emission wavelength (λem = 577 nm) in a very short time (30 s) (Fig. S4, ESI†). This spectral behaviour of BOD-Pyr is suggested to be due to the complexation of AuCl3 with the lone pair of electrons of the pyridylethenyl unit, which terminates the overall PET-quenching process.
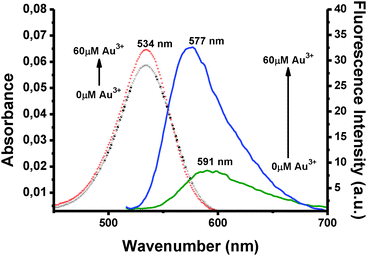 |
| Fig. 1 Absorbance spectra of BOD-Pyr (10 μM) in the absence (black dot-line) and presence (red dot-line) of 6 equiv. (60 μM) of Au3+ and fluorescence spectra of BOD-Pyr (10 μM) in the absence (green line) and presence (blue line) of 6 equiv. (60 μM) of Au3+ in a 0.1 M phosphate buffer/EtOH (pH = 7.0, v/v, 1 : 1). | |
Importantly, neither the absorption nor the fluorescence emission wavelength of BOD-Pyr was affected by changing the polarity of the sensing medium which indicates that BOD-Pyr has indeed no solvatochromic behaviour.
The selectivity profile of BOD-Pyr was surveyed by screening the spectral response towards metal species including Zn2+, Cd2+, Ba2+, Cu2+, Li+, K+, Ni2+, Cr2+, Mg2+, Fe3+, Pb2+, Hg2+, Co2+, Ag+ and Au+. Delightfully, no significant changes were measured in the presence of the other metal species. Only the addition of Au3+ and, to a lesser extent the addition of Au+ resulted in an increase of fluorescence at 577 nm, which obviously implies the high selectivity of BOD-Pyr to gold ions (Fig. S8, ESI†). Fortunately, Hg2+ and Pd2+, being the most competitive metal ions in the detection of gold species did not result in any spectral change.
In order to assess the interference of other metal ions we explored the fluorescence response in the presence of other metal ions. As shown in Fig. S9 (ESI†), the response of BOD-Pyr towards Au3+ was not affected in the presence of other competitive metal species. These results establish that BOD-Pyr can properly detect gold ions in mixtures of other related species.
We further investigated the binding ability of BOD-Pyr to Au3+ by performing a fluorescence titration experiment (Fig. 2 and Fig. S6, ESI†). The emission intensity reached its maximum when 6 equiv. of Au3+ was added and the stoichiometry of the sensing event was established by following the Benesi–Hildebrand method and Job's plot analysis. In addition, there was a good linear relationship between the fluorescence intensity and the concentration of the Au3+ ions (0.2 to 0.7 equivalents of Au3+) (Fig. S10, ESI†). From this data the detection limit of BOD-Pyr was evaluated as 4.0 μM. A straight line was obtained from the plot of ln[(F − F0)/(Fmax − F)] against ln[Au3+] and the related binding constant was determined to be 4.9 × 104 M−2. Moreover, the Job's plot analysis supported a 2
:
1 stoichiometry for the complexation. Despite great efforts, we failed to grow a single crystal of the binding complex suitable for XRD analysis in order to confirm the binding stoichiometry.
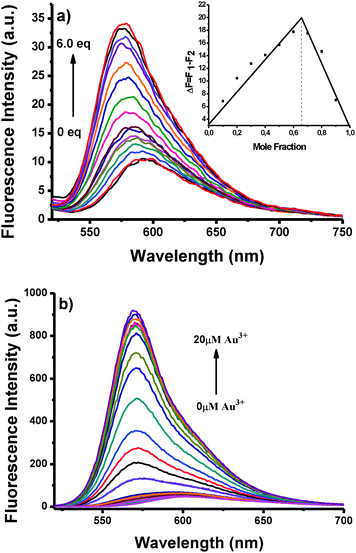 |
| Fig. 2 Fluorescence spectra of BOD-Pyr (10 μM) in the presence of increasing concentrations of Au3+ (a) (0–60 μM, 0–6 equiv.) in a 0.1 M phosphate buffer/EtOH (pH = 7.0, v/v, 1 : 1) (λexc = 500 nm at 25 °C) and (b) (0–20 μM, 0–2 equiv.) in DCE. Inset: Job’s plot analysis between BOD-Pyr and Au3+. The total concentration of BOD-Pyr and Au3+ was kept constant at 20 μM. | |
The specific binding of Au3+ to the pyridine ligand was clearly followed by the aid of 1H-NMR spectroscopy. Upon the binding of AuCl3 with BOD-Pyr, the pyridine ring protons (Hb, Hc and Hd) of the binding complex were dramatically shifted to a higher frequency (downfield shift), consistent with the coordination of nitrogen to AuCl3 (Fig. 3b and Fig. S15, ESI†).
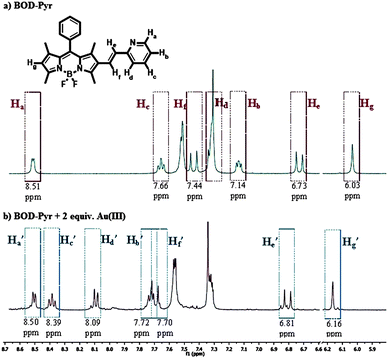 |
| Fig. 3 (a) Partial 1H NMR spectra of BOD-Pyr and (b) BOD-Pyr + AuCl3 (2 equiv.). | |
Further experiments revealed that the binding ability of BOD-Pyr to Au3+ could be dramatically improved in non-aqueous sensing media. For example in DCE the binding constant was evaluated to be 1.8 × 105 M−2, which was visibly greater than that measured in aqueous media (Fig. S14, ESI†).
In non-aqueous sensing media, where the effects of solvation are minimized, the complexation of Au3+ to the BODIPY–pyridine conjugate gave a 20-fold fluorescence enhancement, which is visibly greater than the fluorescence increase observed in aqueous media. Similar to the measurements carried out in aqueous conditions, the fluorescence titration profile of BOD-Pyr with Au3+ in a non-aqueous medium showed a linear relationship for the concentration range of 0.05–1.0 μM (Fig. S16, ESI†). Notably, the detection limit of BOD-Pyr was significantly improved to nanomolar levels (63 nm, S/N > 3). However, it is worth mentioning here that, despite the improved sensitivity, the selectivity towards Au3+ was dramatically decreased in non-aqueous media.
The sensing mechanism of the probe was designed to be reversible. To get insight into whether the sensing mechanism is reversible or not, an excess amount of CN− ions (NaCN, 6.0 equiv.) were introduced into a solution containing 10 μM of dye and 6.0 equivalents of Au3+ (0.1 M phosphate buffer/EtOH (pH = 7.0, v/v, 1
:
1)). As shown in Fig. S12 (ESI†), the fluorescence intensity of the probe solution was immediately reduced by the addition of CN− which proves the reversibility of the recognition process (Scheme 2).
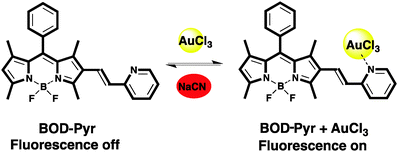 |
| Scheme 2 Reversible interaction of BOD-Pyr with Au3+. | |
Response of BOD-Pyr toward pH changes
In addition to being exceptionally selective towards Au3+ ions, BOD-Pyr is also sensitive to changes in pH within the acidic pH range. Free BOD-Pyr, which exhibits a faint fluorescence emission at 591 nm in the pH range of 7–12, gave an immediate strong emission band at 564 nm upon protonation of the pyridylethenyl moiety (Fig. 4b). As the pH of the solution decreased from 7.0 to 2.0, concomitantly the emission intensity increased by 18-fold. The quantum yield of BOD-Pyr at pH = 2.0, was determined to be 0.39 using rhodamine 6G (ΦF = 0.95 in ethanol) as a standard dye.
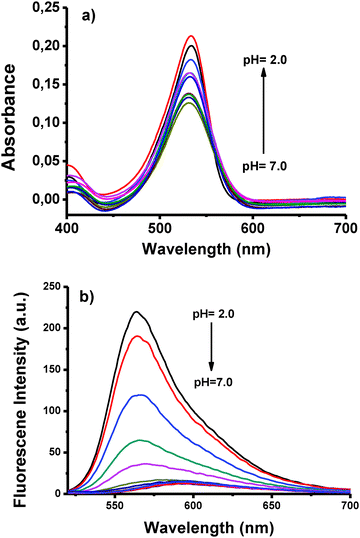 |
| Fig. 4 (a) Absorbance and (b) fluorescence spectra of BOD-Pyr (10 μM) in a 0.1 M phosphate buffer/EtOH (v/v, 1 : 1) at various pH values (2.0–7.0) (λexc = 500 nm, 25 °C). | |
As for the gold sensing process, BOD-Pyr operates in a reversible manner towards the detection of hydronium ions (Scheme 3). The reversible interaction between BOD-Pyr and H3O+ was confirmed by the addition of OH− ions to the acidified probe solution (BOD-Pyr + H3O+) which resulted in a sharp decrease in the emission intensity.
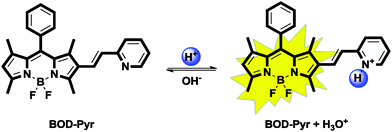 |
| Scheme 3 Reversible protonation of BOD-Pyr. | |
The regeneration of the fluorescence was possible by introducing H3O+ ions into the solution. Obviously, the “off–on” switching ability of the system proves the reversibility of the process (Fig. 5). In addition, the acidity constant pKa of the probe was established using the Henderson–Hasselbalch equation (Fig. S20, ESI†). A linear fit was obtained within the range of pH from 2.0 to 6.0 and the pKa value was calculated to be 3.06 ± (0.14). All of these results indicate that BOD-Pyr can be utilized on demand as a “turn-on” fluorescent probe for monitoring acidity changes within the pH range of 7.0–2.0.
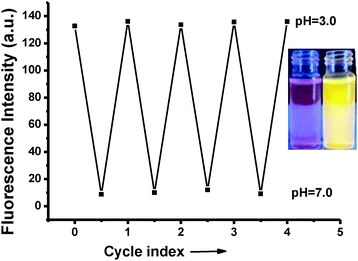 |
| Fig. 5 pH reversibility study of BOD-Pyr (10 μM) between the pH values of 7.0 and 3.0 in water/EtOH (v/v, 1 : 1) (λexc = 500 nm, λem = 564 nm, 25 °C). Inset: fluorescence photograph of BOD-Pyr at pH = 7.0 (left) and 3.0 (right) under illumination with 365 nm light. | |
Quantitative detection of residual Au3+
In order to assess whether BOD-Pyr could be applied for the monitoring of residual Au3+ ions in a synthetic end-product we performed a known chemical transformation utilizing AuCl3 as the active catalyst.43 To this end, a propargylic amide derivative in dichloromethane was rapidly transformed into its Oxazole derivative under the catalysis of AuCl3 (10 mol%) (Scheme 4).
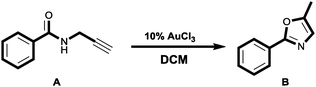 |
| Scheme 4 AuCl3 catalysed cyclization reaction of propargyl amide. | |
The crude product of the chemical reaction was subjected to chromatographic purification using silica as the stationary phase and dichloromethane as an eluent. A defined amount of the purified sample (2 mg) was added to a solution of BOD-Pyr (10 μM in a 0.1 M phosphate buffer/EtOH (pH = 7.0, v/v, 1
:
1)). Immediately, a distinct change in colour and fluorescence was observed in the probe solution indicating the presence of gold species in the solution. This observation is consistent with previous literature reports, where others have proven that a synthetic end product may still contain residues of the metal catalyst even after chromatographic purification.
By the aid of fluorescence measurements the gold content in the sample solution was measured to be 1.8 × 10−8 mol mg−1 based on a standard calibration curve (Fig. S16, ESI†). This result was also consistent with that obtained by inductively coupled plasma mass spectrometry (ICP-MS) analysis (1.27 × 10−8 mol mg−1). With this experiment we unambiguously confirmed the viability of BOD-Pyr for quantitative gold analysis.
Conclusions
To close, we have constructed a dual response fluorescent chemosensor for the rapid detection of gold species and, also for monitoring changes in acidity (pH = 2–7). This novel chemosensor (BOD-Pyr) comprises a pyridylethenyl unit as the recognition site and a BODIPY core as the fluorophore unit. The sensing mechanism of BOD-Pyr is based on the selective binding of gold ions to the pyridyl nitrogen atom which is recognized by a distinct change in the emission intensity. Importantly, BOD-Pyr represents a rare example of a fluorescent probe that operates “reversibly” towards gold species. As a practical application we have successfully documented the utility of BOD-Pyr for the quantification of gold ion residues in synthetic chemicals that were prepared via gold catalysis.
Experimental section
General methods
All reagents were purchased from commercial suppliers (Aldrich and Merck) and they were used without further purification. 1H NMR and 13C NMR were measured on a Varian VNMRJ 400 Nuclear Magnetic Resonance Spectrometer. A Bruker MALDI-TOF mass spectrometer was used for mass spectrometry analysis. The UV absorption spectra were obtained on a Shimadzu UV-2550 spectrophotometer. The fluorescence measurements were performed by using a Varian Cary Eclipse fluorescence spectrophotometer. Samples were placed in 10.0 mm path length quartz cuvettes (2.0 mL volume). Upon excitation at 500 nm, the emission spectra were integrated over the range of 520 nm to 750 nm. The slit width was 5 nm for both excitation and emission. The melting points were determined by using an Electrothermal Melting Point Apparatus 9200. The pH was recorded by a HI-8014 instrument (HANNA). All measurements were conducted at least in triplicate.
Synthesis of BOD-Pyr
To a solution of BODIPY-2 (100 mg, 0.285 mmol) in dioxane (10 mL) was added triphenyl(2-pyridylmethyl)phosphonium chloride hydrochloride (389 mg, 0.896 mmol). Then, 250 μL of triethyl amine was added drop by drop and the resultant solution was stirred at room temperature overnight. After the reaction was complete, the solution was concentrated under vacuum and was extracted three times with dichloromethane. The organic layer was dried over sodium sulfate and was concentrated under reduced pressure. The resultant residue was purified by silica gel column chromatography (hexane/ethyl acetate (8/1)) to afford BOD-Pyr as a green solid (68.1 mg, 56% yield). Mp: 267–269 °C. 1H NMR (400 MHz, CDCl3) δ: 8.56 (d, J = 4.0 Hz, 1H), 7.62 (dt, J = 8.0, 1.6 Hz 1H), 7.52–7.50 (m, 3H), 7.46 (s, 1H), 7.32–7.29 (m, 2H), 7.28 (s, 1H), 7.12–7.09 (m, 1H), 6.72 (d, J = 16.0 Hz, 1H), 6.01 (s, 1H), 2.76 (s, 3H), 2.58 (s, 3H), 1.51 (s, 3H) and 1.38 (s, 3H). 13C NMR (100 MHz, CDCl3) δ: 156.3, 155.9, 154.9, 149.6, 143.8, 141.8, 139.3, 136.5, 135.0, 132.0, 131.0, 129.5, 129.2, 129.1, 128.1, 127.8, 123.9, 121.8, 121.8, 14.7, 14.5, 14.1 and 12.9. Calcd for C26H24BF2N3: 427.203 [M]+, found: 428.244 [M + H]+.
Acknowledgements
We thank the İzmir Institute of Technology (İZTECH) and TUBİTAK for financial support.
Notes and references
- I. Braun, A. M. Asiri and A. S. K. Hashmi, ACS Catal., 2013, 3, 1902 CrossRef CAS.
- Z. Li, C. Brouwer and C. He, Chem. Rev., 2008, 108, 3239 CrossRef CAS PubMed.
- A. Arcadi, Chem. Rev., 2008, 108, 3266 CrossRef CAS PubMed.
- A. S. K. Hashmi and M. Rudolph, Chem. Soc. Rev., 2008, 37, 1766 RSC.
- N. Krause and C. Winter, Chem. Rev., 2011, 111, 1994 CrossRef CAS PubMed.
- C. F. Shaw III, Chem. Rev., 1999, 99, 2589 CrossRef PubMed.
- I. Ott, Coord. Chem. Rev., 2009, 253, 1670 CrossRef CAS PubMed.
- M. Navarro, Coord. Chem. Rev., 2009, 253, 1619 CrossRef CAS PubMed.
- C. M. Goodman, C. D. McCusker, T. Yilmaz and V. M. Rotello, Bioconjugate Chem., 2014, 15, 897 CrossRef PubMed.
- A. Habib and M. Tabata, J. Inorg. Biochem., 2004, 98, 1696 CrossRef CAS PubMed.
- W. D. Block and E. L. Knapp, J. Pharmacol. Exp. Ther., 1945, 83, 275 CAS.
- X. Li, X. Gao, W. Shi and H. Ma, Chem. Rev., 2014, 114, 590 CrossRef CAS PubMed.
- K. P. Carter, A. M. Young and A. E. Palmer, Chem. Rev., 2014, 114, 4564 CrossRef CAS PubMed.
- X. Chen, T. Pradhan, F. Wang, J. S. Kim and J. Yoon, Chem. Rev., 2012, 112, 1910 CrossRef CAS PubMed.
- X. Su and I. Aprahamian, Chem. Soc. Rev., 2014, 43, 1963 RSC.
- Z. Guo, S. Park, J. Yoon and I. Shin, Chem. Soc. Rev., 2014, 43, 16 RSC.
- M. J. Jou, X. Chen, K. M. K. Swamy, H. N. Kim, H.-J. Kim, S. G. Lee and J. Yoon, Chem. Commun., 2009, 7218 CAS.
- O. A. Egorova, H. Seo, A. Chatterjee and K. H. Ahn, Org. Lett., 2010, 12, 401 CrossRef CAS PubMed.
- Y. K. Yang, S. Lee and J. Tae, Org. Lett., 2009, 11, 5610 CrossRef CAS PubMed.
- L. Yuan, W. Lin, Y. Yang and J. Song, Chem. Commun., 2011, 47, 4703 RSC.
- M. Emrullahoğlu, E. Karakuş and M. Üçüncü, Analyst, 2013, 138, 3638 RSC.
- J.-B. Wang, Q.-Q. Wu, Y.-Z. Min, Y.-Z. Liu and Q.-H. Song, Chem. Commun., 2012, 48, 744 RSC.
- E. Karakuş, M. Üçüncü and M. Emrullahoğlu, Chem. Commun., 2014, 50, 1119 RSC.
- M. Üçüncü and M. Emrullahoğlu, Chem. Commun., 2014, 50, 5884 RSC.
- M. Üçüncü, E. Karakuş and M. Emrullahoğlu, Chem. – Eur. J., 2015 DOI:10.1002/chem.201502411.
- H. Seo, M. E. Jun, O. A. Egorova, K. H. Lee, K. T. Kim and K. H. Ahn, Org. Lett., 2012, 14, 5062 CrossRef CAS PubMed.
- N. Y. Patil, V. S. Shinde, M. S. Thakare, P. H. Kumar, P. R. Bangal, A. K. Barui and C. R. Patra, Chem. Commun., 2012, 48, 11229 RSC.
- M. Dong, Y.-W. Wang and Y. Peng, Org.
Lett., 2010, 12, 5310 CrossRef CAS PubMed.
- J. Y. Choi, G.-H. Kim, Z. Guo, H. Y. Lee, K. M. K. Swamy, J. Pai, S. Shin, I. Shin and J. Yoon, Biosens. Bioelectron., 2013, 49, 438 CrossRef PubMed.
- J. H. Do, H. N. Kim, J. Yoon, J. S. Kim and H.-J. Kim, Org. Lett., 2010, 12, 932 CrossRef CAS PubMed.
- Z. Öztaş, M. Pamuk and F. Algi, Tetrahedron, 2013, 69, 2048 CrossRef PubMed.
- Y. Yang, C. Yin, F. Huo and J. Chao, RSC Adv., 2013, 3, 9637 RSC.
- S. Singha, D. Kim, H. Seo, S. W. Cho and K. H. Ahn, Chem. Soc. Rev., 2015, 44, 4367 RSC.
- J. Wang, W. Lin, L. Yuan, J. Song and W. Gao, Chem. Commun., 2011, 47, 12506 RSC.
- E. Deniz, G. C. Isbasar, Ö. A. Bozdemir, L. T. Yıldırım, A. Siemiarczuk and E. U. Akkaya, Org. Lett., 2008, 10, 3401 CrossRef CAS PubMed.
- S. Wagner, K. Brödner, B. A. Coombs and U. H. F. Bunz, Eur. J. Org. Chem., 2012, 2237 CrossRef CAS PubMed.
- L. Zhou, C.-C. Zhu, Y.-S. Xue, W.-J. He, H.-B. Du, X.-Z. You and Y.-Z. Li, Inorg. Chem. Commun., 2013, 35, 355 CrossRef CAS PubMed.
- N. Boens, V. Leen and W. Dehaen, Chem. Soc. Rev., 2012, 41, 1130 RSC.
- R. Ziessel, G. Ulrich and A. Harriman, New J. Chem., 2007, 31, 496 RSC.
- A. Loudet and K. Burgess, Chem. Rev., 2007, 107, 4891 CrossRef CAS PubMed.
- G. Ulrich, R. Ziessel and A. Harriman, Angew. Chem., Int. Ed., 2008, 47, 1184 CrossRef CAS PubMed.
- M. Isik, T. Ozdemir, I. S. Turan, S. Kolemen and E. U. Akkaya, Org. Lett., 2013, 15, 216 CrossRef CAS PubMed.
- A. S. K. Hashmi, J. P. Weyrauch, W. Frey and J. W. Bats, Org. Lett., 2004, 6, 4391 CrossRef CAS PubMed.
Footnote |
† Electronic supplementary information (ESI) available: Absorbance and fluorescence data and all experimental procedures. See DOI: 10.1039/c5nj01664a |
|
This journal is © The Royal Society of Chemistry and the Centre National de la Recherche Scientifique 2015 |
Click here to see how this site uses Cookies. View our privacy policy here.