DOI:
10.1039/C4MT00263F
(Paper)
Metallomics, 2015,
7, 165-173
The combination of arsenic and cryptotanshinone induces apoptosis through induction of endoplasmic reticulum stress-reactive oxygen species in breast cancer cells
Received
4th October 2014
, Accepted 7th November 2014
First published on 7th November 2014
Abstract
Arsenic trioxide has been successfully used for the treatment of patients with acute promyelocytic leukemia (APL) worldwide. Recently, it has also been further developed to treat solid tumors in clinical trials. However, the therapeutic effects on malignant tumors appeared to be unsatisfactory, as these cells exhibited resistance towards arsenic. In this study, we explored new therapeutic strategies for treatment of human breast cancer MCF-7 cells based on arsenic metabolites. The MCF-7 cells were exposed to three arsenic species, namely, inorganic arsenite (iAsIII) and its intermediate metabolites monomethylarsonous acid (MMAIII) and dimethylarsinous acid (DMAIII) either alone or in combination with cryptotanshinone (CPT) to establish their anticancer effects against MCF-7 cells. Surprisingly, MCF-7 cells were shown to be resistant to both iAsIII and CPT when used alone; however, they were shown to be relatively sensitive to treatment when exposed to MMAIII and DMAIII alone. Conversely, the combination of MMAIII with CPT showed significantly enhanced anticancer effects on MCF-7 cells at low doses, but no appreciable effect was observed upon exposure to the other two arsenic species with CPT. In addition, remarkable redistribution of pro-apoptosis related proteins Bax and Bak was observed in the mitochondria, together with activation of poly(ADP-ribose) polymerase (PARP) and caspase-9 after exposure to the combination of MMAIII with CPT. Furthermore, we clearly found that induction of apoptosis in MCF-7 cells was predominantly triggered by endoplasmic reticulum (ER) stress after exposure to the combination of MMAIII with CPT.
Introduction
Arsenic trioxide (As2O3) is widely used for the treatment of patients with acute promyelocytic leukemia (APL) and has shown remarkable clinical success.1 Therefore, it has also been used in the treatment of other forms of tumors and diseases.2,3 However, many reports have indicated that As2O3 as a single-agent has not been as effective as anticipated against non-promyelocytic leukemia and other malignant tumors in clinical trials.4,5
Generally, arsenic trioxide is commonly hydrolyzed to arsenite (i.e., As2O3 → iAsIII) and then metabolized in the liver to trivalent monomethylarsonous acid (MMAIII) and dimethylarsinous acid (DMAIII) species by arsenic methyltransferase (AS3MT) in the body and is finally excreted into urine as low toxic pentavalent methylated forms.6–8 Moreover, these trivalent intermediate metabolites have been shown to be more cytotoxic towards different cell lines as compared to their precursor, iAsIII.9,10 However, so far there is no information regarding the anticancer effects of arsenic intermediate metabolites (i.e., MMAIII and DMAIII) in clinical trials. Thus, arsenic intermediate metabolites may have more stronger anticancer effects on malignant cancers than that of inorganic iAsIII.
To date, many scientists have tried to develop the single-agent As2O3 for treatment of solid tumors in clinical trails, but it has not shown any significant response against solid cancer tumors including hepatocellular carcinoma (HCC) or multiple myeloma (MM).11 Although arsenic trioxide has shown potent antiproliferative and proapoptotic effects in pancreatic cancer cells, despite this promising in vitro data, As2O3 has not demonstrated any satisfactory outcomes in pancreatic cancer patients.4 Moreover, Hayashi et al.12 found that As2O3 around 2–5 μM could induce apoptosis in drug-resistant multiple myeloma cell lines or primary patient cells, but the exposure concentrations are indeed very high. Such reports suggest that the efficacy of As2O3 as a single-agent is not significantly acceptable for the treatment of non-APL cancer. As an alternative, efforts may be made to reduce the dose of arsenic trioxide or may be combined with other standard regimen in reversing the chemoresistance.
Recently, a great increase in the public concern and awareness about breast cancer has been observed. The human breast adenocarcinoma cell line MCF-7, an estrogen receptor (ER) positive breast cancer cell line, has been used as a cellular model to study As2O3 treatment. However, clinically achievable concentrations of As2O3 at 2 μM (i.e., 4 μM iAsIII) have shown no effectiveness in breast cancer in clinical trials.12,13 Different from the singe-agent treatment, Baumgartnerwe et al. reported that the combination of As2O3 (1 μM) with docosahexaenoic acid (DHA) could remarkably enhance the apoptotic effect in drug-resistant leukemia cells as compared to As2O3 treatment alone. Moreover, they observed that the combination treatment was also effective against other cancer cells that were derived from various hematologic malignancies,14 indicating the combination of As2O3 with other agents as an alternative anticancer treatment strategy. Our previous work found that the combination of iAsIII with cryptotanshinone (CPT), isolated from the root of Salvia miltiorrhiza, at sub-toxic concentrations significantly increased the anticancer effects of arsenic on human multiple myeloma (MM) cell lines as compared with the arsenic treatment alone, implying that the combination of arsenic with other natural chemical compounds could improve the treatment for drug resistant malignant cancers.15 CPT has been identified to have anti-inflammatory, anti-cancer, antioxidative and anti-angiogenic activities.16 Especially, recent studies have indicated that CPT exerts potential anticancer activity through targeting STAT3 signaling, inhibiting the signaling pathway of the mammalian target of rapamycin (mTOR) and mTOR-mediated cyclin D1 expression and Rb phosphorylation.
Based on arsenic metabolism in the body which results in the formation of active arsenic intermediate metabolites, we have tried to develop a novel therapeutic strategy for human breast cancers. Here we have examined the anti-cancer effect of arsenite and its intermediate metabolites (i.e., MMAIII and DMAIII) alone and in combination with CPT in the human breast cancer cell line MCF-7. We found that the MCF-7 cells were much more resistant to iAsIII as compared to the two methylated arsenic species, however, the combination of 1 μM MMAIII with 15 μM CPT at sub-toxic concentrations was found to significantly reduce the cell survival. In addition, we found that the combination of MMAIII with CPT induced apoptosis in MCF-7 cells predominantly through activation of ER stress, but not by the STAT3 pathway. Hence, our findings suggest that the combination of arsenic intermediate metabolites with CPT has more potent anticancer activity as compared to iAsIII, thereby this approach might be used for the treatment of human breast cancer in clinical trials in near future.
Materials and methods
Reagents
All reagents were of analytical grade. Milli-Q water (Millipore) was used throughout the experiment. Trizma® HCl and Trizma® base were purchased from Sigma (St. Louis, MO, USA). L-Cysteine, sodium arsenite (iAsIII), sodium arsenate (iAsV), and dimethylarsinic acid [(CH3)2AsO(OH)] (DMAV) were purchased from Wako Pure Chemical Industries, Ltd (Osaka, Japan). Monomethylarsonic acid (MMAV) was obtained from Tri Chemicals (Yamanashi, Japan).
Cell culture
Human breast cancer MCF-7 cells were purchased from Chinese Academy of Sciences in Shanghai. The cells were cultured in the logarithmic growth phase using RPMI-1640 medium supplemented with 10% fetal bovine serum (FBS) (Invitrogen, Grand Island, USA), 100 U mL−1 penicillin, and 100 μg mL−1 streptomycin, at 37 °C in a 5% CO2 atmosphere. After twenty-four hours of seeding, the cultures were washed twice with PBS, fresh medium was added, and then the cells were treated with indicated doses of iAsIII, MMAIII, DMAIII alone or in combination with CPT for 24 h. In the current study, iAsIII will represent As2O3.
Preparation of monomethylarsonous acid (MMAIII) and dimethylarsinous acid (DMAIII)
MMAIII and DMAIII were prepared by reducing MMAV and DMAV, respectively, with 5 molar equivalents of L-cysteine in distilled water at 90 °C for 1 h.10 The trivalent forms were confirmed by comparison of the respective retention times on a GS 220 gel filtration column by HPLC-ICP MS with those prepared from their iodide forms in distilled water under a nitrogen atmosphere. The purity of MMAIII (98%, with 2% of MMAV) and DMAIII (95%, with 5% of DMAV) was confirmed by HPLC-ICP MS and then were used for further experimental purpose.
MTT assay for cell viability
Human breast cancer MCF-7 cells were seeded at a density of 2 × 104 cells per 100 μL per well in 96-well microtiter plates (Promega Corporation). Twenty-four hours post-seeding, the cultures were washed twice with PBS and then exposed to various concentrations of iAsIII, MMAIII and DMAIII or CPT for 24 h. Then, 20 μL of an MTT solution was added to each well (at the final concentration of 0.5 mg mL−1), and the plates were incubated for an additional 3 h at 37 °C. Afterward, cell cultures were washed with PBS, and 150 μL of DMSO was added to each well. Cell viability was measured as absorbance at 570 nm using a microplate reader and the results were expressed as percentage of the control level.
Western blot analysis
MCF-7 cells were washed twice with cold D-Hanks solution, followed by the whole cells using RIPA lysis buffer containing 50 mM Tris-HCl, pH 7.5, 150 mM NaCl, 1% NP-40, 0.5% sodium deoxycholate, 0.1% SDS, 0.2 mM PMSF, and a complete mini protease inhibitor tablet. Lysates were incubated on ice for 30 min and centrifuged for 30 min at 13
000 × g at 4 °C to obtain the supernatant. Protein concentrations were determined by Bio-Rad microprotein assay using bovine serum albumin as the standard. Twenty-five micrograms of each protein sample were resolved by 10 or 12% SDS-PAGE and electro-blotted on nitrocellulose membranes (Bio-Rad, Mississauga, ON). The membranes were blocked for 1 h at room temperature using 5% skimmed milk plus 0.1% Tween-20 (PBST) and incubated overnight at 4 °C with different primary antibodies, followed by incubation with HRP-linked secondary antibodies for 1 h at room temperature then after washing the proteins were visualized by enhanced chemiluminescence (ECL).
Assessment of cellular apoptosis
Cellular apoptosis was measured by Annexin V-FITC and propidium iodide (PI) staining. MCF-7 cells were treated with 1 μM iAsIII, MMAIII and DMAIII alone or in combination with 15 μM CPT for 24 h. Later the cells were washed with PBS and re-suspended (1 × 106 mL−1). The cells were then stained with 5 μL of Annexin V-FITC and were incubated for 15 min in dark at 37 °C. Afterward, PI (20 μg mL−1) was added and the samples were immediately analyzed on a flow cytometer (Beckman Coulter).
Measurement of intracellular ROS by flow cytometry
The oxidation-sensitive fluorescent probe (DCFH-DA) was used to detect the intracellular ROS level, as described in our previous work.10 Briefly, MCF-7 cells were treated with 1 μM MMAIII alone or in combination with 15 μM CPT for 12 h. After incubation, cells were washed with PBS and then incubated with 10 μM L−1 DCFH-DA at 37 °C for 20 min. Fluorescence was detected using a flow cytometer (Beckman Coulter).
Immunofluorescence microscopy
MCF-7 cells (1 × 105) were cultured on Chamber slides and then exposed to 1 μM MMAIII alone or with CPT for 12 h. After washing with PBS twice, the slides were fixed by paraformaldehyde (PFA) for 30 min. The cell nuclei were stained with 4′,6-diamidino-2-phenylindole (DAPI, blue) for 5 min at 4 °C. After washing with PBS, phycoerythrin-conjugated streptavidin was added for 30 min before washing, mounted in Aquamount, and examined under a Zeiss (Göttingen, Germany) 510 confocal microscope. Confocal scanning parameters were set up so that the cells in the well without the compounds had no fluorescence signal.
Statistical analysis
Each viability value represents the mean ± S.D. of four determinations, and IC50 values were calculated from the log–log plot between the percentages of viable cells. Subsequently, each experiment was performed at least three times. Statistical analysis of data was carried out using a one-way ANOVA followed by the Holm–Sidak pairwise multiple comparison test (Sigmaplot, Systat Software Inc), and a probability value of less than 0.05 (*P < 0.05) was accepted as a significant difference.
Results
Effect of three arsenic species with or without CPT on cell viability of MCF-7 cells
Arsenic trioxide is commonly metabolized in the body to mono- and di-methylated arsenic metabolites such as monomethylarsonous acid (MMAIII) and dimethylarsinous acid (DMAIII), whereas, iAsIII represents As2O3 in the present study.
MCF-7 cell survival was determined by MTT assay after exposure to iAsIII, MMAIII, DMAIII and CPT alone or in combination at indicated concentrations for 24 h, as shown in Fig. 1A and B. Human breast cancer MCF-7 cells exhibited resistance to both inorganic iAsIII and CPT alone, whereas they were found to be relatively more sensitive towards MMAIII, and less sensitive towards DMAIII. However, when the three arsenic species were administered, no appreciable toxic effect was found in MCF-7 cells following exposure to low dose, 1 μM (Fig. 1).
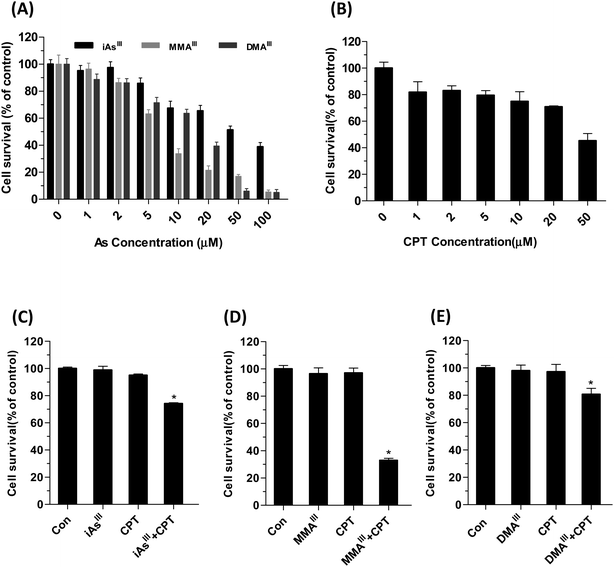 |
| Fig. 1 Effect of three arsenic species with or without CPT on the viability of MCF-7 cells. Human breast cancer MCF-7 cells were exposed to indicated concentrations of iAsIII, MMAIII, DMAIII (A), and cryptotanshinone (B) for 24 h. Additionally, cells were also exposed to the combination of 1 μM iAsIII (C), MMAIII (D), DMAIII and (E) with 15 μM CPT for 24 h. Cell viability was determined by MTT assay as described in Materials and methods. Data are expressed as mean values ± S.D. Asterisks (*) indicate a significant difference between the CPT and arsenic species treated groups at P < 0.05. | |
Based on these results, we further compared the cytotoxic effect of three arsenic species at 1 μM concentration with 15 μM CPT on MCF-7 cells (Fig. 1C–E). Interestingly, after exposure to MMAIII with CPT, the cell viability was significantly reduced to approximately 40% of control (Fig. 1D), however, about 75–80% of cell survival was observed when MCF-7 cells were exposed to the combination of iAsIII or DMAIII at 1 μM concentration with 15 μM CPT (Fig. 1C and E), suggesting that the combination of MMAIII with CPT has a much stronger synergistic effect than the other two species on the MCF-7 cells. In addition, the induction of apoptosis after exposure to 1 μM iAsIII, MMAIII or DMAIII with 15 μM CPT was also determined (Fig. 2A). As anticipated, the combination of MMAIII with CPT showed a significant induction of cellular apoptosis (Fig. 2A), and remarkable activation of poly(ADP-ribose) polymerase (PARP) and the apoptosis related proteins were observed (Fig. 2B). However, no appreciable effect was observed after exposure to other two species including iAsIII and DMAIII with CPT under the same conditions, which was found to be consistent with the results of MCF-7 cell viability (Fig. 2B and C).
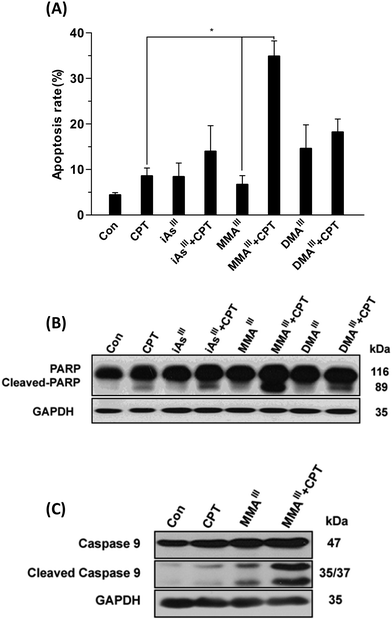 |
| Fig. 2 Determination of apoptosis in MCF-7 cells after exposure to the combination of arsenicals with CPT. MCF-7 cells were exposed to 1 μM concentration of iAsIII, MMAIII and DMAIII alone or with 15 μM CPT for 24 h. Induction apoptosis was determined by flow cytometry (A). Poly(ADP-ribose) polymerase (PARP) (B) and caspase-9 (C) were determined by western blot. Proteins (25 μg) extracted from whole cells were separated by electrophoresis on a 12% SDS–polyacrylamide gel as described in “Materials and methods.” Actin was used as a loading control. Asterisks (*) indicate a significant difference between the CPT and MMAIII treated groups at P < 0.05. | |
Changes in proapoptotic proteins Bax, Bak and cyt c in the cytoplasm and mitochondria of MCF-7 cells following exposure to three arsenic species with or without CPT
In order to understand the mechanism of synergistic effects of the three arsenic species with CPT changes in proapoptotic proteins including Bax, Bak or cyt c in the cytoplasm and mitochondria of MCF-7 cells were further determined in terms of induction of MCF-7 cell death as shown in Fig. 3. In fact, inorganic iAsIII with CPT showed a slight increase of the Bax, and reduction of cyt c in mitochondria (Fig. 3A and a), while MMAIII with CPT remarkably induced the translocation of Bax from the cytoplasm to mitochondria, along with an increase in Bak and reduction of cyt c in mitochondria (Fig. 3B and b). However, no related significant changes were observed in MCF-7 cells after exposure to DMAIII with CPT (Fig. 3C and c). These observations were also found to be consistent with the results of cell viability obtained after exposing MCF-7 cells to the combination treatment (Fig. 1D), suggesting that MMAIII with CPT could enhance the induction of apoptosis and has much stronger effects on MCF-7 cells than the other two arsenic species (i.e., iAsIII and DMAIII).
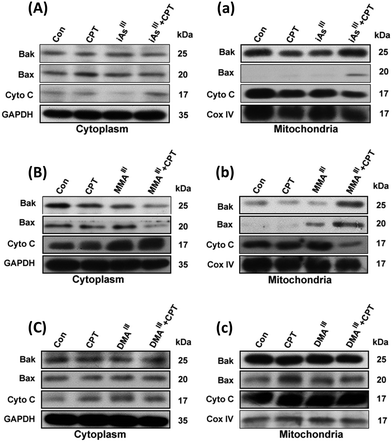 |
| Fig. 3 Changes in proapoptotic proteins in the cytoplasm and mitochondria following exposure to the combination of arsenic with CPT. MCF-7 cells were exposed to 1 μM concentration of iAsIII (A and a), MMAIII (B and b) and DMAIII (C and c) along or with 15 μM CPT for 24 h. The proapoptotic proteins Bak and Bax or cyt c in the cytoplasm (A–C) and mitochondria (a–c) were determined by immunoblotting. | |
Determination of ER stress in MCF-7 cells after exposure to the combination of MMAIII with CPT
Phosphorylation of signal transducer and activator of transcription 3 (STAT3) can be specifically inhibited by CPT.16 Thus, we are interested in determining the effects of the arsenic species on the inhibition of STAT3 activity. Interestingly, phosphorylation of STAT3 (p-STAT3) was not observed after exposure to either of the three arsenic species alone, however, a complete inhibition of STAT3 activity was observed after exposure to the sub-toxic concentration of CPT, as shown in Fig. 4A. In addition, we also found that CPT was able to inhibit p-STAT3 in MCF-7 cells even at low concentrations, 2–10 μM (data not shown), implying that the inhibition of p-STAT3 is not involved in the induction of apoptosis after exposure to the combination of arsenic with CPT. According to the above results, we found that MMAIII with CPT has much potent effects on induction of apoptosis as compared to the other two arsenic species. Thus, we predominantly focused on the effect of MMAIII with CPT on MCF-7 cells in subsequent experiments.
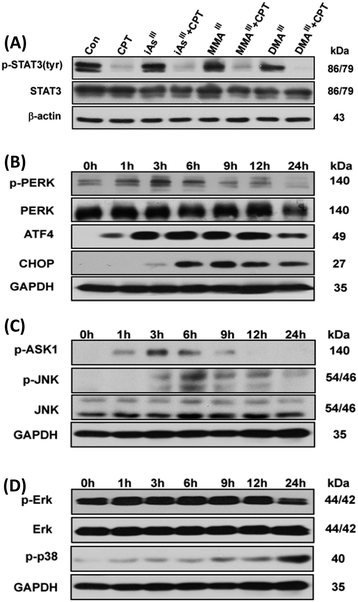 |
| Fig. 4 Combination of MMAIII and CPT induces ER-stress in human breast cancer MCF-7 cells. MCF-7 cells were exposed to1 μM concentration of each arsenic species alone or with 15 μM CPT for 24 h and the changes in phosphor-STAT3 (Try) protein expression (A) were determined. Cells were exposed to 1 μM MMAIII with 15 μM CPT for the indicated time points to determine the changes in p-PERK, ATF4 and CHOP (B) or p-ASK1 and p-JNK (C) as well as p-ERK and p-p38 (D) protein expressions by immunoblotting. | |
It has been reported that ER stress and MAPK pathways play an essential role in the regulation of cellular response such as cellular apoptosis, survival, proliferation and differentiation.17,18 In this study, we opt to find out that whether the induction of apoptosis observed in MCF-7 cells after exposure to the combination of MMAIII with CPT was through ER stress or not, thereby ER-stress related proteins p-PERK, ATF4 and CHOP or p-ASK1 and p-JNK were determined at different time points (0, 1, 3, 6, 9, 12 and 24 h) after exposure to the indicated combination. Surprisingly, a significant induction of p-PERK was observed as early as 1 h after exposure to the combination treatment (i.e. MMAIII with CPT), followed by the activation of the downstream proteins, ATF4 and CHOP, with the increase in the exposure time (Fig. 4B). On the other hand, induction of p-ASK1 and p-JNK was also observed (Fig. 4C), suggesting that the induction of apoptosis by MMAIII with CPT mainly occurred through ER-stress. Conversely, in this study, we were also interested in evaluating the involvement of the MAPK pathway, however, we found that MMAIII with CPT induced the p-ERK and p-38 activation at a very late time, indicating that the MAPK signaling pathway might not be the main pathway involved in the induction of apoptosis in MCF-7 cells after exposure to the combination of MMAIII with CPT.
Effect of p-JNK or caspase inhibitors on induction of apoptosis in MCF-7 cells after exposure to the combination of MMAIII with CPT
Although, MMAIII or CPT alone could not significantly induce ER-stress in MCF-7 cells, a significant induction of ER-stress was observed in MCF-7 cells after exposure to the combination treatment (Fig. 5A). In order to further verify the involvement of ER-stress as a main pathway for the induction of apoptosis in the current study, we used two specific inhibitors to evaluate our results. Interestingly, when the cells were pretreated with a p-JNK inhibitor (SP600125), the induction of apoptosis after combination treatment was significantly attenuated (Fig. 5B), suggesting that MMAIII with CPT induced apoptosis by predominantly triggering ER-stress in MCF-7 cells. Moreover, similar results were observed after the pretreatment of a caspase inhibitor (Z-VAD-FMK), as this inhibitor also prevented the induction of apoptosis by the combination treatment, indicating that the MMAIII with CPT induced apoptosis in MCF-7 cells was caspase dependent (Fig. 5C).
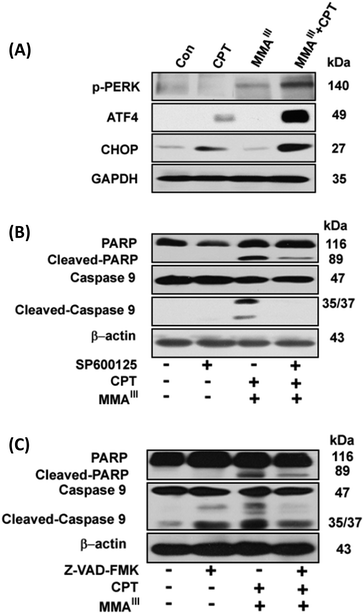 |
| Fig. 5 Effect of p-JNK or caspase inhibitors on induction of apoptosis in MCF-7 cells after exposure to the combination of MMAIII with CPT. MCF-7 cells were exposed to 1 μM MMAIII and 15 μM CPT alone or combination of the two compounds for 24 h to determine the induction of ER-stress (A). The effects of pretreatment of the p-JNK inhibitor (SP600125) (B) or the caspase inhibitor (Z-VAD-FMK) (C) on induction of apoptosis in MCF-7 cells by combination of 1 μM MMAIII and 15 μM CPT. Cleaved caspase-3, -9 and poly(ADP-ribose) polymerase (PARP) were determined by immunoblotting using specific antibodies. Beta-actin was used as a loading control. | |
Localization of reactive oxygen species (ROS) in MCF-7 cells after exposure to the combination of MMAIII with CPT
We were further interested in determining the ER-stress-induced generation of reactive oxygen species (ROS). Thus, the generation of ROS was determined in MCF-7 cells after exposure to MMAIII, CPT alone or MMAIII with CPT in the presence or absence of the antioxidant N-acetylcysteine (NAC) as shown in Fig. 6A. Interestingly, ROS generation was not significantly increased in cells exposed either to MMAIII (1 μM) or CPT (15 μM) alone (data not shown), however, strikingly increased generation of ROS was observed after the exposure to the combination treatment at 12 h. Moreover, this ROS generation was completely inhibited in the cells pretreated with NAC, implying that ROS is also involved in the induction of apoptosis in MCF-7 cells in the current study. In addition, we found that ROS was generated in both ER and mitochondria after exposure to the combination treatment, and no significant ROS were generated in both organelles by exposure to either CPT or MMAIII alone (Fig. 6B). Additionally, the induction of apoptosis was attenuated by pretreatment with NAC following the combination treatment, indicating that the generation of ROS was involved in the apoptosis (Fig. 6C).
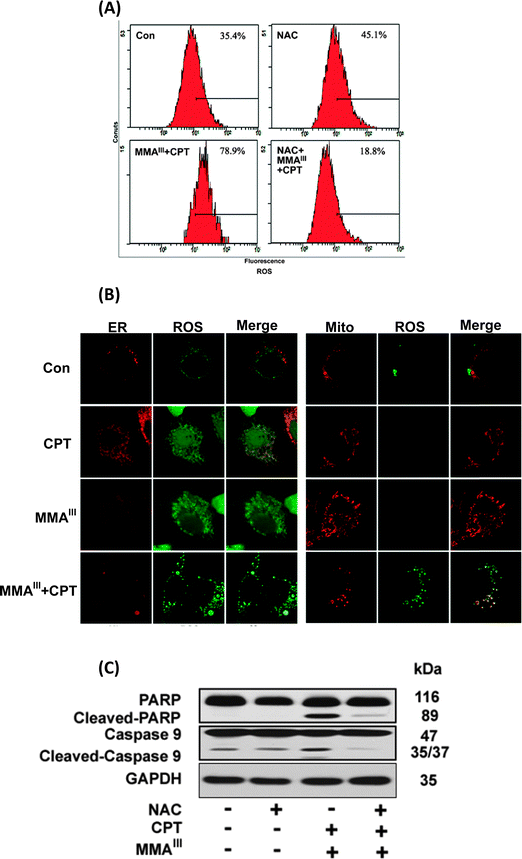 |
| Fig. 6 Determination of generation of ROS and localization in MCF-7 cells following exposure to the combination of MMAIII with CPT. MCF-7 cells were exposed to 1 μM concentration of MMAIII and 15 μM CPT alone or a combination of MMAIII and CPT for 6 h to determine the generation of ROS by flow cytometry (A). MCF-7 cells were seeded on a 6-well culture plates with cover glasses, and then cultured for 24 h. Cells were pre-treated with 2 μM CM-H2DCFDA (green) and ER-tracker Red (red) or Mito-tracker (red) for 30 min. After washing with PBS, cells were exposed to the mentioned concentration as indicated above for 3 h, (B). Following exposure, cells were fixed with 10% formalin, and then ROS generation was determined using a confocal laser scanning microscope. (C) Changes in apoptosis related proteins caspase-9 and PARP in MCF-7 cells after exposure to the combination of MMAIII with CPT in the presence of N-acetylcysteine (NAC) for 24 h. Cleaved caspase-3, -9 and poly(ADP-ribose) polymerase (PARP) were determined by immunoblotting using specific antibodies. | |
Discussion
Arsenic trioxide, a therapeutic agent used for thousands of years in Chinese, Mongolian and Tibetan traditional medicine, has proven to be an effective drug for the treatment of acute promyelocytic leukemia (APL).1 This successful approach has also encouraged many scientists to use this compound for the treatment of other malignant tumors.2–6 However, as a single-agent, As2O3 has shown limited efficacy against non-APL and solid tumors may be because these cells are highly resistant to As2O3.
An early study has reported that high dose of As2O3 may increase the overall survival rate in multiple myeloma (MM) patients, but most of the patients may not tolerate such high-dose chemotherapy.19 Moreover, high dose of As2O3 can also cause a number of adverse events like cytopenia, deep vein thrombosis and infectious complications.20 Thereby, new therapeutic approaches are needed to be developed for the use of arsenicals in clinical treatment against various arsenic-resistant tumors.21–24
On the other hand, it is well known that inorganic arsenic can be metabolized into mono- and dimethylated arsenic species (e.g., MMAIII and DMAIII) by arsenic methyltransferase (AS3MT) in the human body.6,7 Moreover, these two intermediate metabolites have shown to be more toxic than that of their precursor; iAsIII in different cells.8–10 Thus, as an attempt to develop new therapeutic approach that can help increase the anticancer activity and reduce the toxicity of arsenicals, we have established and compared the synergistic effects of three arsenic species in combination with the natural chemical compounds (CPT) using human cancer breast cancer MCF-7 cells. The MTT assay revealed that MCF-7 cells exhibit great resistance for inorganic iAsIII and relative sensitivity towards the two methylated metabolites alone, in particular, for MMAIII (Fig. 1A and B). However, cell viability was significantly reduced with the subtoxic concentration of the MMAIII and CPT combination (Fig. 1D), suggesting that CPT may probably increase the efficacy of MMAIII at low doses against MCF-7 cells.
Activation of mitochondrial-mediated apoptotic pathways is one of the most well-characterized mechanisms of action of iAsIII. In previous work, we have clearly found that the combination of iAsIII and CPT could increase the induction of apoptosis through the mitochondria-mediated apoptotic pathway in human multiple myeloma U266 cells.15 However, we could not find appreciable synergic effects of iAsIII with CPT in MCF-7 cells as compared to our previous work. Conversely, the combination of MMAIII and CPT has shown to have a strong synergistic anticancer effect on MCF-7 cells (Fig. 2). The pro-apoptotic protein Bax was markedly recruited in the mitochondria followed by its reduction in the cytoplasm (Fig. 3), which facilitated the release of cyt c from the mitochondria (Fig. 3). As, it is well known that release of cytochrome c into the cytoplasm can lead to the formation of apoptosome (a complex of dATP, cytochrome c, Apaf-1 and procaspase-9) which results in the activation of caspase-9 that may finally induce cellular apoptosis.25–27
Caspase-3 has been shown to play a key role in apoptotic events such as DNA fragmentation and membrane blabbing, moreover, the caspase-3 null mice have shown to die perinatally displaying hypercellularity.28 Although it has been reported that MCF-7 cells also lack the caspase-3 proteins, the induction of apoptosis in MCF-7 cells was significantly inhibited by pretreatment of Z-VAD-FMK (i.e., inhibitor of apoptosis) (Fig. 5B). Correspondingly, activation of PARP and caspase9 was also clearly found in MCF-7 cells after the combination treatment, implying the apoptotic effect of MMAIII with CPT to be caspase-mediated (Fig. 2). We further found that the induction of apoptosis occurred predominantly through ER-stress instead of the MAPK/ERK pathway (Fig. 5), as ER-stress related proteins p-PERK, ATF4 and CHOP or p-ASK and p-JNK were induced significantly as early as 1–3 h of treatment (Fig. 4). Additionally, combination treatment induced apoptosis was prevented by pretreatment with EIF2 or p-JNK inhibitors (Fig. 5), suggesting that the apoptosis was ER-stress mediated.
Notably, although CPT is known to be capable of inhibiting p-STAT3,16 it seems that the combination of arsenic with CPT did not induce apoptosis through this pathway in MCF-7 cells as observed in the current study. Our previous study has demonstrated mitochondria as a target organelle for methylation induced toxicity, especially, we found that MMAIII is capable of inducing ROS generation in the mitochondria through inhibition of the complexes II and IV of electron transport chain (ETC).29 However, their combination treatment was found to be much toxic to ER in the present study. Future studies need to probe the mechanism by which a combination of MMAIII and CPT induced ROS in ER. Additionally, we also found that oxidative stress was also involved as a toxic event at late time (Fig. 6A), and the localization of ROS was found to be in endoplasmic reticulum (ER) as well as in mitochondria (Fig. 6B). Inhibition of ROS could attenuate the induction of apoptosis mediated by the combination of MMAIII with CPT (Fig. 6C), suggesting that the ER-stress resulted in generation of ROS in ER which also ultimately affected the mitochondria.
Taken together, our data demonstrate that human breast cancer is indeed resistant to the single-agent As3O3 treatment, but we found that the combination of CPT with arsenic intermediate metabolites (particularly MMAIII) could strongly increase the anticancer activity even at low doses. We provided new evidence that the arsenic intermediate metabolite, MMAIII, at low concentrations is able to increase the induction of apoptosis in combination with CPT, suggesting that this combination may be a good therapeutic approach for the treatment of human breast cancer.
Conflicts of interest
The authors report no conflicts of interest.
Abbreviations
APL | Acute promyelocytic leukemia |
As2O3 | Arsenic trioxide |
iAsIII | Arsenite |
CPT | Cryptotanshinone |
Acknowledgements
The authors wish to acknowledge the National Natural Science Foundation of China (No. 81274138 and 81473289), a Key Scientific and Technological Project of Zhejiang Province (No. 2012C13017-1), Doctoral Program of Higher Education (No. 2012332211001), and Zhejiang Provincial Program for the Cultivation of Medical Rookies.
References
- J. Zhu, M. H. Koken, F. Quignon, M. K. Chelbi-Alix, L. Degos, Z. Y. Wang, Z. Chen and H. de Thé, Arsenic-induced PML targeting onto nuclear bodies: implications for the treatment of acute promyelocytic leukemia, Proc. Natl. Acad. Sci. U. S. A., 1997, 94, 3978–3983 CrossRef CAS.
- P. Bobé, D. Bonardelle, K. Benihoud, P. Opolon and M. K. Chelbi-Alix, Arsenic trioxide: a promising novel therapeutic agent for lymphoproliferative and autoimmune syndromes in MRL/lpr mice, Blood, 2006, 108, 3967–3975 CrossRef PubMed.
- R. W. Ahn, F. Chen, H. Chen, S. T. Stern, J. D. Clogston, A. K. Patri, M. R. Raja, E. P. Swindell, V. Parimi, V. L. Cryns and T. V. O'Halloran, A novel nanoparticulate formulation of arsenic trioxide with enhanced therapeutic efficacy in a murine model of breast cancer, Clin. Cancer Res., 2011, 16, 3607–3617 CrossRef PubMed.
- H. L. Kindler, M. Aklilu, S. Nattam and E. E. Vokes, Arsenic trioxide in patients with adenocarcinoma of the pancreas refractory to gemcitabine: a phase II trial of the University of Chicago Phase II Consortium, Am. J. Clin. Oncol., 2008, 31, 553–556 CrossRef CAS PubMed.
- C. C. Lin, C. Hsu, C. H. Hsu, W. L. Hsu, A. L. Cheng and C. H. Yang, Arsenic trioxide in patients with hepatocellular carcinoma: a phase II trial, Invest. New Drugs, 2007, 25, 77–84 CrossRef CAS PubMed.
- K. Rehman and H. Naranmandura, Double-edged effects of arsenic compounds: anticancer and carcinogenic effects, Curr. Drug Metab., 2013, 14, 1029–1041 CrossRef CAS.
- W. W. Wang, Y. F. Lan, K. Rehman, Y. H. Jiang, Y. Maimaitiyiming, D. Y. Zhu and H. Naranmandura, Effect of Arsenic Compounds on the in Vitro Differentiation of Mouse Embryonic Stem Cells into Cardiomyocytes, Chem. Res. Toxicol, 2014 DOI:10.1021/tx500286t.
- D. J. Thomas, The die is cast: arsenic exposure in early life and disease susceptibility, Chem. Res. Toxicol., 2013, 26, 1778–1781 CrossRef CAS PubMed.
- V. Charoensuk, W. P. Gati, M. Weinfeld and X. C. Le, Differential cytotoxic effects of arsenic compounds in human acute promyelocytic leukemia cells, Toxicol. Appl. Pharmacol., 2009, 239, 64–70 CrossRef CAS PubMed.
- K. Rehman, Y. J. Fu, Y. F. Zhang, Q. Q. Wang, B. Wu, Y. Wu, X. Y. Zhou, W. H. Sun, T. F. Sun and H. Naranmandura, Trivalent methylated arsenic metabolites induce apoptosis in human myeloid leukemic HL-60 cells through generation of reactive oxygen species, Metallomics, 2014, 6, 1502–1512 RSC.
- J. Wu, C. Henderson, L. Feun, P. Van Veldhuizen, P. Gold, H. Zheng, T. Ryan, L. S. Blaszkowsky, H. Chen, M. Costa, B. Rosenzweig, M. Nierodzik, H. Hochster, F. Muggia, G. Abbadessa, J. Lewis and A. X. Zhu, Phase II study of darinaparsin in patients with advanced hepatocellular carcinoma, Invest. New Drugs, 2010, 28, 670–676 CrossRef CAS PubMed.
- T. Hayashi, T. Hideshima, M. Akiyama, P. Richardson, R. L. Schlossman, D. Chauhan, N. C. Munshi, S. Waxman and K. C. Anderson, Arsenic trioxide inhibits growth of human multiple myeloma cells in the bone marrow microenvironment, Mol. Cancer Ther., 2002, 1, 851–860 CAS.
- N. C. Munshi, Arsenic trioxide: an emerging therapy for multiple myeloma, Oncologist, 2001, 2, 17–21 CrossRef PubMed.
- M. Baumgartner, S. Sturlan, E. Roth, B. Wessner and T. Bachleitner-Hofmann, Enhancement of arsenic trioxide-mediated apoptosis using docosahexaenoic acid in arsenic trioxide-resistant solid tumor cells, Int. J. Cancer, 2004, 112, 707–712 CrossRef CAS PubMed.
- P. Liu, S. Xu, M. Zhang, W. W. Wang, Y. F. Zhang, K. Rehman, H. Naranmandura and Z. Chen, Anticancer activity in human multiple myeloma U266 cells: synergy between cryptotanshinone and arsenic trioxide, Metallomics, 2013, 5, 871–878 RSC.
- D. S. Shin, H. M. Kim, K. D. Shin, Y. J. Yoon, S. J. Kim, D. C. Han and B. M. Kwon, Cryptotanshinone inhibits constitutive signal transducer and activator of transcription 3 function through blocking the dimerization in DU145 prostate cancer cells, Cancer Res., 2009, 69, 193–202 CrossRef CAS PubMed.
- H. M. Huang, H. Zhang, H. C. Ou, H. L. Chen and G. E. Gibson, alpha-keto-beta-methyl-n-valeric acid diminishes reactive oxygen species and alters endoplasmic reticulum Ca2+ stores, Free Radical Biol. Med., 2004, 37, 1779–1789 CrossRef CAS PubMed.
- W. Zhang and H. T. Liu, MAPK signal pathways in the regulation of cell proliferation in mammalian cells, Cell Res., 2002, 12, 9–18 CrossRef PubMed.
- B. Bruno, M. Rotta, L. Giaccone, M. Massaia, A. Bertola, A. Palumbo and M. Boccadoro, New drugs for treatment of multiple myeloma, Lancet Oncol., 2004, 5, 430–442 CrossRef CAS.
- J. Zhou, Y. Zhang, J. Li, X. Li, J. Hou, Y. Zhao, X. Liu, X. Han, L. Hu, S. Wang, Y. Zhao, Y. Zhang, S. Fan, C. Lv, L. Li and L. Zhu, Single-agent arsenic trioxide in the treatment of children with newly diagnosed acute promyelocytic leukemia, Blood, 2010, 115, 1697–1702 CrossRef CAS PubMed.
- K. B. Kim, A. Y. Bedikian, L. H. Camacho, N. E. Papadopoulos and C. McCullough, A phase II trial of arsenic trioxide in patients with metastatic melanoma, Cancer, 2005, 104, 1687–1692 CrossRef CAS PubMed.
- A. A. Tarhini, J. M. Kirkwood, H. Tawbi, W. E. Gooding, M. F. Islam and S. S. Agarwala, Safety and efficacy of arsenic trioxide for patients with advanced metastatic melanoma, Cancer, 2008, 112, 1131–1138 CrossRef CAS PubMed.
- B. Ardalan, P. R. Subbarayan, Y. Ramos, M. Gonzalez, A. Fernandez, D. Mezentsev, I. Reis, R. Duncan, L. Podolsky, K. Lee, M. Lima and P. Ganjei-Azar, A phase I study of 5-fluorouracil/leucovorin and arsenic trioxide for patients with refractory/relapsed colorectal carcinoma, Clin. Cancer Res., 2010, 16, 3019–3027 CrossRef CAS PubMed.
- L. Podolsky, M. Oh, P. R. Subbarayan, D. Francheschi, A. Livingstone and B. Ardalan, 5-Fluorouracil/Leucovorin and arsenic trioxide for patients with refractory/relapsed colorectal carcinoma: a clinical experience, Acta Oncol., 2011, 50, 602–605 CrossRef CAS PubMed.
- J. C. Yang and G. A. Cortopassi, Induction of the mitochondrial permeability transition causes release of the apoptogenic factor cytochrome c, Free Radical Biol. Med., 1998, 24, 624–631 CrossRef CAS.
- X. Jiang and X. Wang, Cytochrome c promotes caspase-9 activation by inducing nucleotide binding to Apaf-1, J. Biol. Chem., 2000, 275, 31199–31203 CrossRef CAS PubMed.
- M. Narita, S. Shimizu, T. Ito, T. Chittenden, R. J. Lutz, H. Matsuda and Y. Tsujimoto, Bax interacts with the permeability transition pore to induce permeability transition and cytochrome c release in isolated mitochondria, Proc. Natl. Acad. Sci. U. S. A., 1998, 95, 14681–14686 CrossRef CAS.
- M. Woo, R. Hakem, M. S. Soengas, G. S. Duncan, A. Shahinian, D. H. Kagi, A. Hakem, M. McCurrach, W. Khoo, S. A. Kaufman, G. Senaldi, T. Howard, S. W. Lowe and T. W. Mak, Essential contribution of caspase 3/CPP32 to apoptosis and its associated nuclear changes, Genes Dev., 1998, 12, 806–819 CrossRef CAS.
- H. Naranmandura, X. Chen, M. Tanaka, W. Wang, K. Rehman, S. Xu, Z. Chen, S. Q. Chen and N. Suzuki, Release of apoptotic cytochrome c from mitochondria by dimethylarsinous acid occurs through interaction with voltage-dependent anion channel in vitro, Toxicol. Sci., 2012, 128, 137–146 CrossRef PubMed.
|
This journal is © The Royal Society of Chemistry 2015 |
Click here to see how this site uses Cookies. View our privacy policy here.