DOI:
10.1039/C4LC00944D
(Focus)
Lab Chip, 2015,
15, 947-957
Angry pathogens, how to get rid of them: introducing microfluidics for waterborne pathogen separation to children†
Received
13th August 2014
, Accepted 19th November 2014
First published on 19th November 2014
Abstract
The purpose of this paper is to present a new approach for introducing to a non-scientific audience a major public health issue: access to safe drinking water. Access to safe drinking water is a privilege in developed countries and an urgent need in the third world, which implies always more efficient and reliable engineering tools to be developed. As a major global challenge it is important to make children aware of this problem for understanding (i) what safe drinking water is, (ii) how ingenious techniques are developed for this purpose and (iii) the role of microfluidics in this area. This paper focuses on different microfluidic-based techniques to separate and detect pathogens in drinking water that have been adapted to be performed by a young audience in a simplified, recreational and interactive way.
I. Introduction
Diarrhoea is often considered in developed countries as a classical gastrointestinal symptom, while not enjoyable nor usually too serious. However, this illness results in 1.5 million deaths each year, most of which involve children, and is mainly due to the ingestion of pathogens through water, food or unclean hands. The observation in (Prüss-Üstün et al., 2008)1 highlights the high privilege in developed countries to have access to specific water treatments, resulting in the delivery of safe drinking water. However, and despite these treatments, several outbreaks are reported every month. The Drinking Water Inspectorate2 reports around 60 significant events caused by pathogens in water supplies in England and Wales in 2012 whose sources are not always clearly identified. The main difficulties when dealing with pathogens are first to deal with the large variety of existing harmful pathogens (viruses, bacteria and protozoa) and second to detect their presence as they are flowing at extremely small concentrations in large volumes of water. Their separation and detection are thus time-consuming tasks (days are typically needed) that require an experienced staff.3 As a consequence, only three microbiological parameters are set by the European regulation to reflect the water quality: E. coli, Enterococci and Pseudomonas aeruginosa, all set to 0 bacteria per 100 mL of sample (per 250 mL for bottle water).4 Current limitations are thus the correlation between these parameters and the concentration of all waterborne pathogens and the delay of detection of a pathogen than can be long enough to affect a significant part of the population. One could easily imagine how serious the situation could be in the presence of dangerous pathogens resistant to treatment. Cryptosporidium for instance has already been detected in water despite the absence of these indicators,3,5 and is routinely tested for in UK waters. The development of new approaches is thus a growing and necessary research area leading to several new national, European and international projects. For instance, Aquavalens (http://aquavalens.org/) is a European project launched in April 2013 that “is centred on the concept of developing suitable platforms that harness the advances in new molecular techniques to permit the routine detection of waterborne pathogens and improve the provision of hygienically safe water for drinking and food production that is appropriate for large and small systems throughout Europe”. Some of the techniques adapted in this paper for the comprehension of children are funded by this project, which highlights how the proposed public engagement is close to current laboratory techniques under investigation. Both within this project and other research initiatives, many different detection schemes have been proposed3 and sample processing research is also developing. Microfluidics has recently been applied to both sample processing and detection within waterborne pathogen monitoring6,7 with promising results. This paper focuses on how to introduce the existing approach and microfluidic alternatives to children in an interactive and recreational way.
II. Teaching objectives and workflow
There are a lot of different techniques that can be used for pathogen separation and detection. Detection can be based on growing cultures or highly specific biosensors for instance.3 Presenting all the existing techniques would be a tedious task beyond the scope of this activity, and we here focus on emerging microfluidic approaches.
Laursen et al.8 evaluated the impact of scientists in a classroom and features that enhance positive student outcome regarding a specific activity. These features include: (i) equipment and materials that enable science learning experiences, (ii) interesting science topics and (iii) style of presentation with hands-on and inquiry approaches. The proposed activity tries thus to encompass these parameters by selecting some specific separation and detection techniques that can be reproduced easily and handled by children in a recreational but educational approach.
As presented in Fig. 1, this paper focuses on the introduction to waterborne pathogen detection through a set of different modules dedicated to the standardized Immuno-Magnetic Separation (IMS), two microfluidic based separation techniques (IMS and Deterministic Lateral Displacement) and then to pathogen detection by fluorescent labelling. All or a selection of modules could be delivered according to the age of participants, learning objectives, time available, cost, etc. in either schools or as an outreach activity at science festivals. Indicative costs are given for each module independently, however some materials are common to multiple modules, thus reducing total costs. Each module employs familiar and widely available materials. To highlight the feasibility of these modules, each activity has been performed “in-house” without laboratory facilities. Cartoons are also proposed throughout the paper to illustrate the different topics introduced here and to broaden the spectrum of the audience to a non-scientific arena.
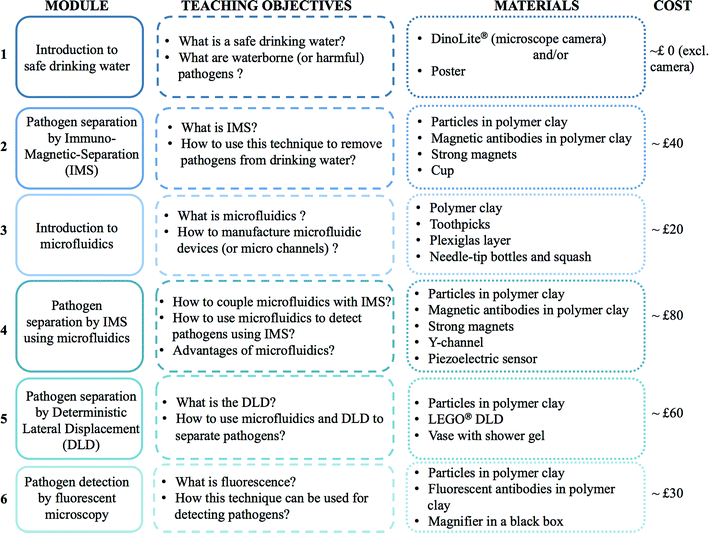 |
| Fig. 1 Workflow of the proposed activity. | |
On one hand, the Immuno-Magnetic Separation is a well-known and efficient technique to separate and concentrate specific biological matters. This technique is part of the standard protocol (USEPA Method 1623) developed for recovery and detection of protozoa. To the best of the authors' knowledge, there is no activity relating this technique for public engagement. On the other hand, microfluidics is an increasingly growing research area whose applications for drinking water are quite scarce, though increasing in recent years.7 Due to its success in research laboratories, literature for introducing microfluidics to students is flourishing as well.9–13 However, most of these papers are targeting middle school, high school or undergraduate students. The audience of the proposed activity is young children, to enhance their interest in science, technology, engineering and mathematics (STEM) and to promote the next workforce generation. By coupling microfluidics to waterborne pathogen detection, an interesting approach is proposed to children for understanding what microfluidics is and how relevant it could be for a concrete application.
III. How it works in research labs…
Fig. 2 shows a standardised method for separating and detecting Cryptosporidium, a well-known and highly resistant pathogen encountered in water systems. This method incorporates five concentration steps with two stages of filtration and elution followed by centrifugation, to minimize the volume of liquid and thus concentrate particles.
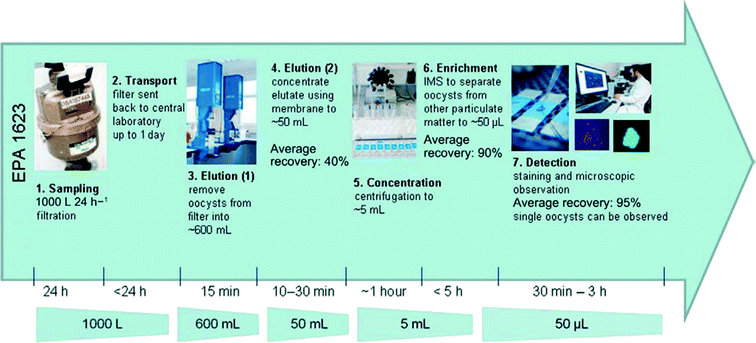 |
| Fig. 2 Overview of the USEPA 1623 method for the detection of Cryptosporidium. Extracted from Bridle et al.6 | |
The filtration steps rely on the size of particles to remove them from the water sample. All particles larger than the pore size of the filter will be trapped while the smaller particles will remain in water. As a consequence, a mix of different particles can be present after the concentration steps as long as they present a diameter larger than the filter pore size, only some of which will be pathogenic. Specific techniques are therefore needed to identify which particles are present to evaluate the water quality and if consumers can safely use this water. The next paragraph presents one of them, namely the Immuno-Magnetic Separation. The accompanying support poster proposed for introducing in a simplified manner notions of waterborne pathogens and their separation is shown in Fig. 3.
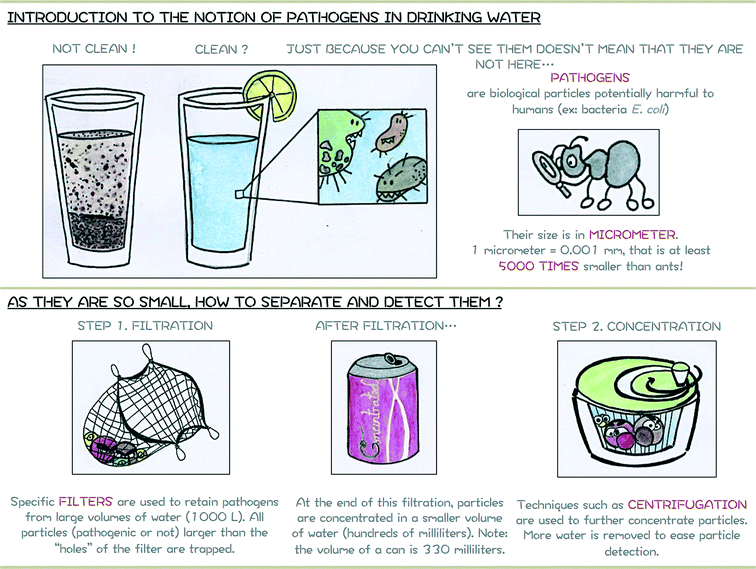 |
| Fig. 3 Module 1 support poster or how to introduce notion of safe drinking and pathogens to a young audience. | |
A. Standardized Immuno-Magnetic Separation (IMS)
The principle of the Immuno-Magnetic Separation (IMS) is schematically represented in Fig. 4. It relies on the addition of specific magnetic beads coated with antibodies14 (e.g. anti-Cryptosporidium if the presence of Cryptosporidium needs to be confirmed). Particles in the sample are only captured if they correspond to the specific anti-bodies coated on the magnetic beads and can then easily be removed using a strong magnet. Although IMS is a powerful technique for separating specific biological particles such as pathogens, the standard protocol is usually limited to small volumes of samples and requires the intervention of experienced staff. Microfluidic-based techniques are a growing topic for proposing smart alternatives to water issues, and one approach that has been taken is to perform on-chip IMS.15–18
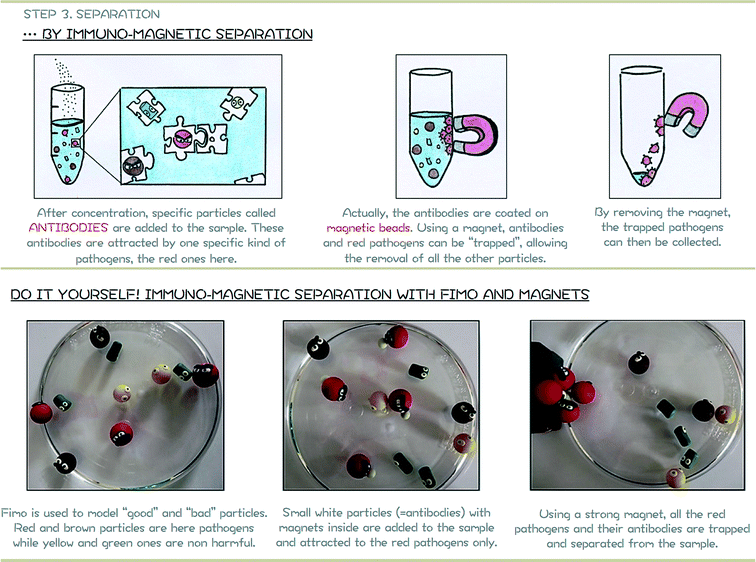 |
| Fig. 4 Module 2 support poster. Top: schematic representation of separation by Immuno-Magnetic Separation (IMS). Bottom: hands-on activity to reproduce standard IMS. | |
B. Microfluidic based separation techniques
Microfluidics is defined as “the science and technology of systems that process or manipulate small (10−9 to 10−18 litres) amounts of fluids, using channels with dimensions of tens to hundreds of micrometres”.19Fig. 5 proposes a cartoon for introducing the notion of microfluidics and the manufacturing procedure of microchannels. The module related to microfluidics (module 3) is of prime importance for allowing children to have a better representation of systems that are presented in the following modules (modules 4 and 5).
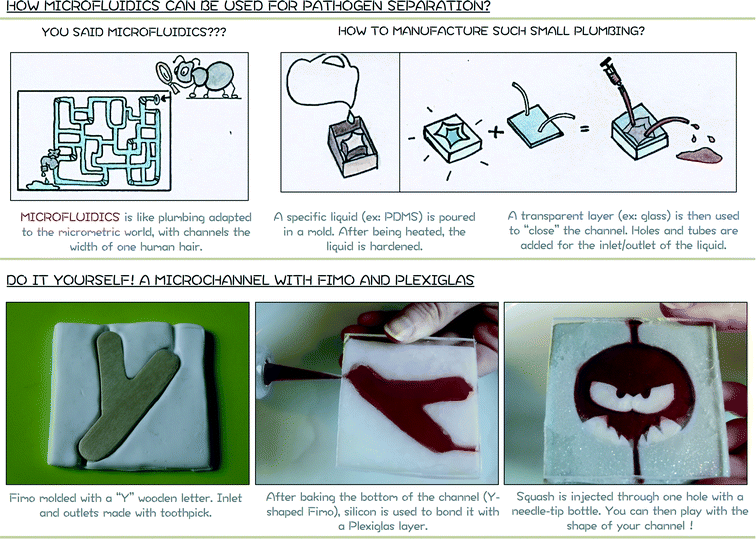 |
| Fig. 5 Module 3 support poster. Top: schematic representation of a microfluidic system and the manufacturing procedure. Bottom: hands-on activity to produce a “micro” channel. | |
B.1. Microfluidic based Immuno-Magnetic Separation.
Microfluidics can offer several advantages to the standardized IMS, which explains the wide range of publications related to this topic.15–18 A microfluidic-based IMS is more automated, can deal with larger volumes of sample than standard IMS and miniaturize the procedure into one on-chip unit. One other main advantage is the possibility to integrate several other procedures within the same device such as the detection of trapped particles. Techniques based on fluorescence detection are for instance proposed in the literature for identifying on-chip the presence of pathogens.18
In order to introduce simply the notion of “multitask” chip, a microfluidic-based IMS is coupled with a piezoelectric sensor (Fig. 6). For pathogen detection, antibodies are usually immobilized onto the surface of a piezoelectric sensor. When pathogens are trapped, a shift in the resonance frequency of the sensor is detected and correlated to the mass of pathogens blocked at the surface.20,21 This approach is here extended to the detection of antibody-coated magnetic beads and pathogens onto the magnet. For a simple realisation, the piezoelectric sensor will detect the vibration due to the impact of particles onto the magnet that will turn on a red LED.
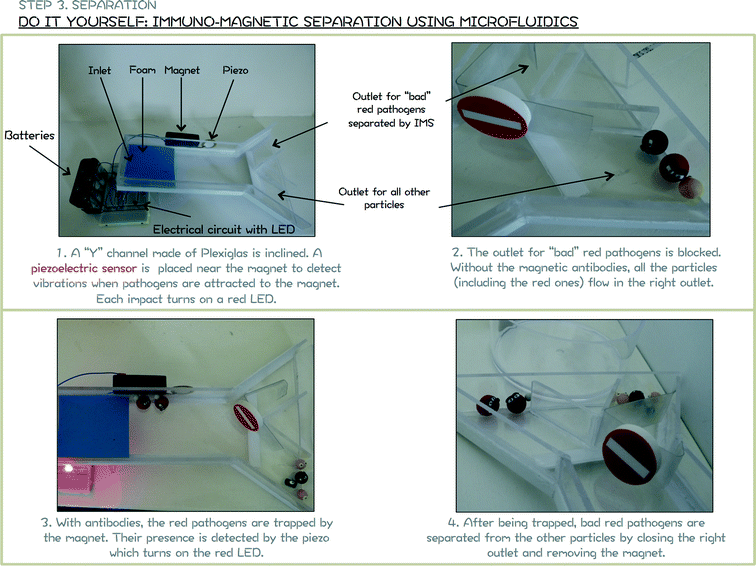 |
| Fig. 6 Module 4 support poster. Hands-on activity to mimic a microfluidic based IMS with detection of trapped pathogens. | |
Immuno-Magnetic Separation provides excellent recovery rates but remains specific to one particle/antibody combination. This procedure has to be iterated if different particles have to be detected and requires the corresponding specific antibodies, which are not always readily available and can be very expensive. When applied to drinking water purposes, this iterative procedure is a limiting step to the fast detection of all the potential harmful pathogens. Moreover, smaller pathogens such as viruses are not concentrated by the centrifugation step (step 5 in Fig. 2), they will remain in the supernatant and require further specific and expensive steps to be separated such as ultracentrifugation.
B.2. Deterministic Lateral Displacement (DLD).
Different techniques have been developed in the literature for sorting particles using microfluidic devices.7,22 This paper only focuses on one of these techniques, referred to as Deterministic Lateral Displacement (DLD), initiated by the work of Huang et al. in 200423 and easily reproducible at a macroscopic scale with LEGO®,24–26 thus highly suitable for manipulation/visualisation by children. The basic idea of DLD is to separate particles by changing their trajectory within a channel depending on their size. “Large” particles (i.e., particles larger than a critical diameter defined below) are deviated from their initial position due to the presence of posts placed in the microchannel.
These posts are designed within a specific geometry and periodicity in order to separate particles above a desired critical diameter Dc:27
with
G the distance between two posts (see
Fig. 7) and
ε defined as
|  | (2) |
where
d is the shift between two successive vertical posts,
λ is the centre-to-centre distance between two successive horizontal posts (see
Fig. 7),
N is the periodicity of the post array and
θ is the angle of deviation of the posts.
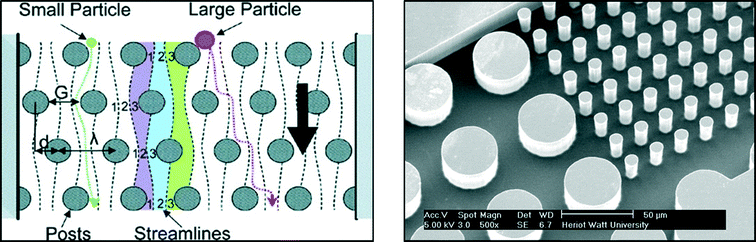 |
| Fig. 7 Example of microfluidic channel for particle separation based on DLD. Left: schematic representation of DLD principle extracted from Davis et al.28 Right: magnified view of a DLD device used for separating bacteria from blood – IB3 and MISEC group at Heriot Watt University. | |
Due to the specific fluid motion present in devices containing posts, particles above the critical diameter are deviated while small particles follow an ultimately straight path. This technique is relevant for introducing the safe drinking water challenge since pathogens present different characteristic sizes depending on their kingdom (nanometres for viruses, around a micrometre for bacteria and several micrometres for protozoa). Although studies focusing on the separation of non-spherical biological particles are limited and need further investigation before this method can be fully applied to waterborne pathogens, DLD devices can be produced at a macroscopic scale with LEGO®. This offers an excellent interactive approach to introduce current research aims to children and is easy to implement in schools or during outreach activities for example. The support poster proposed for introducing the notion Deterministic Lateral Displacement is proposed in Fig. 8.
 |
| Fig. 8 Module 5 support poster. Top: schematic representation of the DLD principle. Bottom: hands-on activity to mimic a size-based separation using DLD. | |
C. Detection
The last step of the process to be introduced to children is the detection of the separated pathogens. This process usually relies on the labelling of pathogens with specific fluorescent antibodies. Using a fluorescent microscope, pathogens conjugated with fluorescent antibodies can then easily be detected and counted (Fig. 9).
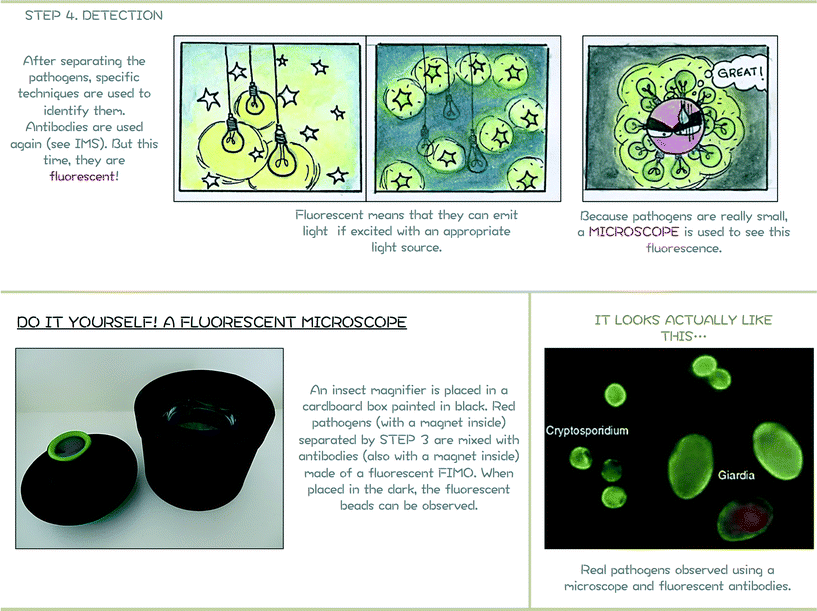 |
| Fig. 9 Module 6 support poster. Top: schematic representation of the fluorescence phenomenon. Bottom: hands-on activity to visualize fluorescent particles using a low-cost microscope. Image with real pathogens reproduced from Bouzid et al.29 | |
IV. How it works with children…
Now that the challenge of pathogen separation and detection has been introduced, this paper presents an easy and interactive way to reproduce and illustrate these different techniques with children. Detailed explanations to reproduce all the experiments are proposed in the ESI.†
A. Immuno-Magnetic Separtion with FIMO®
Pathogens and other biological particles are represented in a simplified and magnified manner using FIMO® clay (Fig. 4-bottom). FIMO® is a soft polymer clay, available in a large range of colours, that can be easily shaped and then hardened after baking for 30 minutes in an oven at 110 °C.
In this paper and for ease of children's understanding, two kinds of particles have been represented:
– “Bad” particles, red and brown particles in Fig. 4, 6 and 8. “Bad” particles represent waterborne pathogens, defined by the Environmental Agency as microorganisms capable of causing disease that may be transmitted via water and acquired through ingestion, bathing or by other means. The size of red and brown particles is roughly the same (diameter around 1.6 cm). In order to let children identify which are these “bad” particles, they are represented with angry faces. Note that faces could be directly painted on baked polymer clay by children. In this paper, angry faces are also made with the polymer clay. To reproduce the Immuno-Magnetic Separation, small magnetic beads are incorporated inside the red “bad” particles (Fig. 4-bottom) before baking.
– “Good” particles defined as non-harmful for humans. These particles are the yellow and green ones throughout the paper. These particles are smaller than the bad ones to be then separated using DLD which, as mentioned previously, is a separation technique considering the particle size as the sorting parameter.
Note that this representation of “good” and “bad” particles with different sizes is obviously largely simplified in comparison to the reality. Even within a same “family” of pathogens, some are harmful while some others are not. Challenges for researchers are still to define the pathogenic characteristics of these particles, a problematic far too complex to be introduced within minutes to children.
Assuming this simplification, the Immuno-Magnetic Separation focuses here on the removal of red particles. The magnetic antibodies are represented in Fig. 4 by small fluorescent beads made also with FIMO® (fluorescent FIMO® no. 04). Small magnetic beads are also incorporated in these fluorescent beads before baking to be attracted toward the red particles. Children only have to incorporate these fluorescent beads in the sample and observe that they are directly attracted by the red “bad” particles. A strong magnet is then used to remove all (and only) the red “bad” particles.
B. Microfluidics
In order to understand the notion of microfluidics and its relevance to waterborne pathogen separation, a simple procedure based again on FIMO® is proposed. Using a block of FIMO® that is flattened with a book or a rolling pin, a channel is created by using a mould, a Y wooden letter for example here. A Y-channel is produced to complement the Y-channel proposed for the microfluidic-based IMS (module 4), although this approach allows an infinite number of designs to be created (see angry pathogen device bottom right of Fig. 4). Three smaller channels are then produced using a toothpick to allow the liquid to enter and exit the device. To close the channel, a piece of Plexiglas is used. After baking the FIMO® block, transparent silicon for bathrooms is finally used to bond it to the Plexiglas layer. Using a needle-tip bottle, red liquid (e.g. squash or food dye) is incorporated through one of the hole.
Yang et al.10 proposed in their paper an interactive and hands-on activity for manufacturing magnified microfluidic devices with Jell-O® dessert. This fun and simplified approach, closer to the actual procedure of manufacturing, can directly be related to the proposed activity if time is available. However, the FIMO® approach allows children to easily touch and mould their own device during an outreach activity for instance.
B.1. Microfluidic based Immuno-Magnetic Separation.
For the microfluidic-based IMS, a Y-shaped channel (29 cm in length, 5 cm in width and 3.5 cm in height) made of Plexiglas is inclined. A similar device made with a plastic bottle is also proposed in the ESI† for reducing the costs of the activity. The outlets of the channels (two branches of the Y-channel) can be let opened to allow the fluid and particles to be collected in two different cups. A small support is fixed on the wall of the channel to hold the magnet while being easily removable by children. A piezoelectric sensor is then placed next to the magnet with transparent blue tack to detect the shock of trapped particles against the magnet. For safety reasons, the magnet and piezoelectric sensor are placed outside the channel to avoid any contact with water. A small piece of foam (see Fig. 6) is placed at the bottom of the channel inlet to absorb the shock when particles are entering the channel and to avoid false detections by the piezoelectric sensor. Each shock detected by the sensor propagates a current through an electrical circuit (cf. ESI†) to finally here turn on a light (LED). Extensions of this system can easily be imagined by placing a buzzer, several LEDs to know the force of the impact against the magnet, etc. At the beginning of the experiment, a set of particles is poured in the device just above the foam (Fig. 6). Since the device is inclined, particles will roll down by gravity. The outlet on the left of the channel is initially closed, here by a piece of flexible plastic from a plastic bottle. All the particles will then flow in the right outlet of the device. A second experiment is performed with, this time, magnetic beads incorporated inside the red bad pathogens and by adding antibodies also with magnets (similarly to the standard IMS). Red pathogens and antibodies are attracted to each other and, while flowing in the device, will be deviated by the magnet. When trapped, the piezoelectric sensor will detect the shock that will then turn on the light to warn of pathogen presence. Once pathogens are detected, the right outlet of the channel is closed, the left one opened and the magnet removed. All the trapped particles will finally flow in the left outlet thus are separated from the other particles.
B.2. Separation of all the “bad” particles using DLD and LEGO®.
After this first separation and detection step, children should notice that other “bad” particles (brown particles in Fig. 4 and 6) remain in the water sample and cannot be separated by IMS since they don't have the corresponding antibodies in this activity. The last step of this experience thus consists of trying to remove all the “bad” particles with another technique, the Deterministic Lateral Displacement (DLD) presented previously. Microchannels and posts used in our laboratory are here represented by a rectangular vase (IKEA®, Rektangel) and LEGO® board with cylindrical LEGO® posts of diameter D = 7.8 mm to shape the obstacles (Fig. 8).
The positions of the posts are crucial to separate “good” from “bad” particles. In this paper, the following configuration is proposed:
– Gap between two posts G = 1.7 cm.
– ε = 0.37. This parameter can easily be determined by measuring the angle θ between the first blue line with the vertical axis. ε can be deduced given θ and based on eqn (2).
Based on eqn (1), the critical diameter of this system is thus 1.47 cm. As presented in Fig. 8, red and brown particles with a diameter around 1.6 cm are larger than the critical diameter and are thus deviated in the device to follow the blue path. Yellow (1.1 cm in diameter) and green (0.8 cm in diameter) particles are smaller than the critical diameter and follow a straight path within the LEGO® device.
It can be noted that such macroscopic experiments cannot be performed in water. Microfluidics is characterized by laminar flow and thus slow fluid motion. To reproduce this phenomenon, viscous media have to be considered. While glycerol is considered in some studies,24–26 in the present paper and for safety reasons, diluted shower gel is used. Depending on the product used and especially its viscosity, it can be used pure, without dilution, but if the viscosity is too high, particles will need a very long time to pass through the LEGO® device. If so, a slight dilution with tap water can solve the problem. The shower gel should be carefully introduced in the vase to avoid air bubbles to be trapped in the liquid. Due to the high viscosity of the solution, air bubbles require a long time to rise and hinder any visualization in the vase. The liquid should be carefully introduced by using for instance the LEGO® board to pour the liquid against and avoid bubble formation. Finally, it is important to mention the higher the device, the larger the displacement between large and small particles.
C. Detection with insect magnifier
After separation, the number of “bad” particles trapped by IMS are counted by fluorescence. All the trapped particles are placed within a fake fluorescent microscope composed of an insect magnifier for children placed in a black-painted cardboard box to see the fluorescence of the fluorescent magnetic beads (the fluorescence of beads is hardly visible with daylight) (Fig. 9).
Even though simplified in comparison with the real process for labelling pathogens, this approach allows children to be introduced to complex notions such as antibodies, fluorescence, microscopy while being able to run the whole experiment on their own.
V. Conclusion
This paper presents a new and original approach to introduce children major scientific challenges. A recreational and interactive procedure is proposed to define notions of safe drinking water, pathogens, separation, detection and microfluidics. By simplifying and magnifying laboratories procedures, the next work-force generation can enjoy being part of the research world by visualizing, testing, running experiments and analysing results related to this water problem. The procedure has been developed as a story, starting from the presence of particles in water that require magnifying techniques to be visualized, then a first separation procedure (Immuno-Magnetic Separation) specific to one particle/antibody combination. The several advantages offered by microfluidics are then introduced in the context of waterborne pathogen separation. Once all the components containing in this activity are completed (FIMO® beads, LEGO® board, etc.), the duration of this “story” is about 30 minutes. The activity can easily be shortened by not presenting all the modules proposed in the paper. The total cost of each module is kept as low as possible (around £15 for the vase, £20 for the LEGO®, £10 for FIMO®, £20 for the magnetic beads, £20 for the shower gel, £10 for the cardboard box and the insect magnifier, £25 for the piezoelectric sensor). The activity presented in this paper is easy to run and can involve children from the beginning (particle modelling, etc.) to introduce complex notions in a fun and interactive manner. Such activities are of prime interest to master children with the science world, interesting and increasingly growing research topics and perhaps promote scientific vocations.
List of abbreviations:
DLD |
Deterministic Lateral Displacement
|
IMS |
Immuno-Magnetic Separation
|
LED |
Light-Emitting Diode
|
STEM |
Science, technology, engineering and mathematics
|
USEPA |
US Environmental Protection Agency
|
Conflict of interest statement
The authors declare no commercial or financial conflict of interest.
Acknowledgements
HB would like to acknowledge The Royal Academy of Engineering/EPSRC for her research fellowship. Both HB and MJ would like to acknowledge EU funding for the project “AQUAVALENS: protecting the health of Europeans by improving methods for the detection of pathogens in drinking water and water used in food preparation”.
References
-
A. Prüss-Üstün, R. Bos, F. Gore and J. Bartram, Safer water, better health: costs, benefits and sustainability of interventions to protect and promote healt, World Health Organization, Geneva, 2008 Search PubMed
.
- Drinking Water Inspectorate ( 2012), Drinking Water 2012.
-
H. Bridle, Waterborne Pathogens: Detection Methods and Applications, Elsevier Science, 2013 Search PubMed
.
- World Health Organisation, Guidelines for drinking-water quality, 2011.
-
G. Zuccheri and N. Asproulis, Detection of Pathogens in Water Using Micro and Nano-Technology, IWA Publishing, 2012 Search PubMed
.
- H. Bridle, M. Kersaudy-Kerhoas, B. Miller, D. Gavriilidou, F. Katzer and E. A. Innes,
et al. Detection of Cryptosporidium in miniaturised fluidic devices, Water Res., 2012, 46(6), 1641–1661 CrossRef CAS PubMed
.
- H. Bridle, B. Miller and M. P. Desmulliez, Application of microfluidics in waterborne pathogen monitoring: A review, Water Res., 2014, 55(0), 256–271 CrossRef CAS PubMed
.
-
S. Laursen, C. Liston, H. Thiry and J. Graf, What good is a scientist in the classroom? Participant outcomes and program design features for a short-duration science outreach intervention in K-12 classrooms, CBE—Life Sciences Education, ed. B. Schulz, 2007, vol. 6, pp. 49–64 Search PubMed.
- K. L. Berkowski, K. N. Plunkett, Q. Yu and J. S. Moore, Introduction to photolithography: Preparation of microscale polymer silhouettes, J. Chem. Educ., 2005, 82(9), 1365 CrossRef CAS
.
- C. W. T. Yang, E. Ouellet and E. T. Lagally, Using inexpensive jell-o chips for hands-on microfluidics education, Anal. Chem., 2010, 82(13), 5408–5414 CrossRef CAS PubMed
.
- D. Nguyen, J. McLane, V. Lew, J. Pegan and M. Khine, Shrink-film microfluidic education modules: Complete devices within minutes, Biomicrofluidics, 2011, 5(2), 022209 CrossRef PubMed
.
- M. Hemling, J. A. Crooks, P. M. Oliver, K. Brenner, J. Gilbertson, G. C. Lisensky and D. B. Weibel, Microfluidics for high school chemistry students, J. Chem. Educ., 2014, 91(1), 112–115 CrossRef CAS
.
- P. A. E. Piunno, A. Zetina, N. Chu, A. J. Tavares, M. O. Noor, E. Petryayeva, U. Uddayasankar and A. A. Veglio, Comprehensive microfluidics device construction and characterization module for the advanced undergraduate analytical chemistry laboratory, J. Chem. Educ., 2014, 91(6), 902–907 CrossRef CAS
.
- J. Y. Lee and R. A. Deininger, Detection of E. coli in beach water within 1 hour using immunomagnetic separation and ATP bioluminescence, Luminescence, 2004, 19, 31–36 CrossRef CAS PubMed
.
- O. Laczka, J. M. Maesa, N. Godino, J. del Campo, M. Fougt-Hansen, J. P. Kutter and E. Baldrich, Improved bacteria detection by coupling magneto-immunocapture and amperometry at flow-channel microband electrodes, Biosens. Bioelectron., 2011, 26(8), 3633–3640 CrossRef CAS PubMed
.
- Q. Ramadan, L. Christophe, W. Teo, L. ShuJun and F. H. Hua, Flow-through immunomagnetic separation system for waterborne pathogen isolation and detection: Application to Giardia and Cryptosporidium cell isolation, Anal. Chim. Acta, 2010, 673(1), 101–108 CrossRef CAS PubMed
.
- O. Rotariu, I. D. Ogden, M. MacRae, L. E. Udrea and N. J. Strachan, Multiple sample flow through immunomagnetic separator for concentrating pathogenic bacteria, Phys. Med. Biol., 2005, 50(12), 2967 CrossRef PubMed
.
- J. Qiu, Y. Zhou, H. Chen and J. M. Lin, Immunomagnetic separation and rapid detection of bacteria using bioluminescence and microfluidics, Talanta, 2009, 79(3), 787–795 CrossRef CAS PubMed
.
- G. M. Whitesides, The origins and the future of microfluidics, Nature, 2006, 442, 368–373 CrossRef CAS PubMed
.
- G. A. Campbell and R. A. Mutharasan, Method of measuring Escherichia coli O157: H7 at 1 cell/mL
in 1 liter sample using antibody functionalized piezoelectric-excited millimeter-sized cantilever sensor, Environ. Sci. Technol., 2007, 41(5), 1668–1674 CrossRef CAS
.
- X. L. Su and Y. Li, A self-assembled monolayer-based piezoelectric immunosensor for rapid detection of Escherichia coli O157: H7, Biosens. Bioelectron., 2004, 19(6), 563–574 CrossRef CAS
.
- N. Pamme, Continuous flow separations in microfluidic devices, Lab Chip, 2007, 7(12), 1644–1659 RSC
.
- L. R. Huang, E. C. Cox, R. H. Austin and J. C. Sturm, Continuous particle separation through deterministic lateral displacement, Science, 2004, 304(5673), 987–990 CrossRef CAS PubMed
.
- M. Balvin, E. Sohn, T. Iracki, G. Drazer and J. Frechette, Directional locking and the role of irreversible interactions in deterministic hydrodynamics separations in microfluidic devices, Phys. Rev. Lett., 2009, 103, 078301 CrossRef
.
- J. Frechette and G. Drazer, Directional locking and deterministic separation in periodic arrays, J. Fluid Mech., 2009, 627, 379–401 CrossRef
.
- T. J. Bowman, G. Drazer and J. Frechette, Inertia and scaling in deterministic lateral displacement, Biomicrofluidics, 2013, 7(6), 064111 CrossRef PubMed
.
-
J. A. Davis, Microfluidic separation of blood components through deterministic lateral displacement, Princeton University, 2008 Search PubMed
.
- J. A. Davis, D. W. Inglis, K. J. Morton, D. A. Lawrence, L. R. Huang, S. Y. Chou, J. C. Sturm and R. H. Austin, Deterministic hydrodynamics: Taking blood apart, Proc. Natl. Acad. Sci. U. S. A., 2006, 103(40), 14779–14784 CrossRef CAS PubMed
.
- M. Bouzid, D. Steverding and K. M. Tyler, Detection and surveillance of waterborne protozoan parasites, Curr. Opin. Biotechnol., 2008, 19(3), 302–306 CrossRef CAS PubMed
.
Footnote |
† Electronic supplementary information (ESI) available. See DOI: 10.1039/c4lc00944d |
|
This journal is © The Royal Society of Chemistry 2015 |