International research agency perspectives on potable water reuse
Received
30th June 2015
, Accepted 1st August 2015
First published on 4th August 2015
Abstract
As the demand for potable quality water increases, driven by increasing human populations and economic development, the drivers for indirect and direct potable reuse of water also increase in their intensity. In this paper we inspect the national situations in developed countries in the northern and southern hemispheres (the USA and Australia), and in a middle-income country (South Africa). Examples of full scale potable reuse schemes in each country are described, followed by summaries of current research focal areas and priority challenges. Finally the international research trends and commonalities are highlighted, along with the key regional differences in research objectives and application, concluding with an outline of perceived opportunities for mutual benefit by enhanced international cooperation.
Water impact
Water is a limiting resource, and the pressure exerted on surface and groundwater resources should be reduced or at best maintained, rather than increased, as the human population and industrial development increase. Water recycling and reuse is thus of increasing importance, not only in arid regions but also in cities and contaminated environments. This paper provides an international understanding to inform optimization of treatment under local conditions, operating efficiencies associated with chemical and energy inputs or recovery, and optimization of traditional wastewater treatment to complement water recycling. Large quantities of freshwater can be saved by industrial water reuse and recycling, lowering costs, reducing environmental pollution and improving carbon footprint. Reuse is a sustainable and cost-effective alternative water supply.
|
Introduction
Water recycling is almost universal. The global installed capacity of water reuse plants is around 50
000 megalitres per day (ML per d), a little more than half of which has been treated to the tertiary level (suspended particles have been removed and the water has been disinfected).1 Actual output from water reuse plants has been estimated at about 60% of capacity.
Water reuse is thus of increasing importance, not only in arid regions but also in growing cities and contaminated environments. It addresses the challenges of deteriorating quality of raw water sources and increasing instability of supply, as a complement to demand management (imposed restrictions) and seawater desalination (which generally would remain a higher cost water than reused water).
Large quantities of freshwater can be saved by industrial water reuse and recycling, lowering costs, reducing environmental pollution and improving carbon footprint. Reuse enhances industrial water supply security, which may be endangered in the future due to increased freshwater demands from other sectors including agricultural use and potable purposes. In addition to maintaining industrial processes, water reuse provides cost benefits (in most cases municipal water and desalinated seawater are the most expensive sources of water for industrial use).
Water reuse is being recognised as a more sustainable and cost-effective alternative water supply, using natural processes as well as more advanced membrane technologies with more efficient energy use than in the past. A wide variety of treatment options exists, such that health-related risks are considered manageable through the multiple barrier approach. Reuse has gone through a rapid curve of innovation and multiple technologies are now available. For example, the market volume for membranes has boomed in the last few years.
Annual expenditure on building water reuse projects is currently in the region of US$2.4 billion. This is expected to rise to US$8.4 billion in 20162 as communities look to reinforce and diversify their supply strategies.
The EU's AQUAREC project in 2006 identified over 3300 water reclamation projects across the world.3 Japan had the largest number of reuse projects (1800), followed by the USA (1600), Australia (450) and the EU (230).
Water reuse cannot take place in isolation. Communities and businesses need to start managing water differently, bringing competing demands and users together, and adopting an integrated approach to the entire catchment area. This includes treating wastewater to appropriate standards for different types of reuses (e.g. cooling, building, mining, agriculture, landscaping, aquifer recharge, and potable reuse). In Japan, most projects involved urban recycling. By contrast, projects in the USA and EU were relatively evenly split between urban and agricultural projects with a smaller number of industrial and mixed-use projects. Most EU projects were in southern Europe, predominantly in Spain, and mainly for agricultural and urban/industrial use.4
South Africa
Water is a limiting resource in South Africa, especially in areas where runoff exceeds rainfall. The pressure exerted on surface and groundwater resources should be reduced or at best maintained, rather than increased, as the human population and industrial development increase. There has thus been a lot of interest recently in direct water reclamation (direct potable reuse (DPR), and direct industrial reuse). The shortage of water is leading to large-scale interest in and application of reclamation and reuse of wastewater to sustain development and economic growth in the region. Desalination of wastewater by membrane processes, in combination with other technologies, can be used to diversify water supplies, but the details of implementation on a site-specific basis are still under investigation.
Water reclamation and reuse has been studied and practised in the region since the 1960s, which has led to the first direct water reclamation (DPR) plant being built in Windhoek, Namibia. Ongoing research and development at Windhoek has led to this plant being internationally considered as an effective multi-barrier treatment system. South Africa has taken a conscious decision to regulate water quality according to its intended use, instead of according to its source, hence all potable water supplies are required to meet the same national standard (SANS241-1:2011)5 regardless of whether the raw water was groundwater, surface water, treated wastewater, or seawater.
The National Development Plan6 charts a new development path to stimulate growth, eliminate poverty and reduce inequality amongst South Africans by 2030. The plan contains a dedicated section on Water Resources and Services stating that “before 2030, all South Africans will have affordable access to sufficient safe water and hygienic sanitation to live healthy and dignified lives”. This includes assuring water supply by investment in water infrastructure and reuse in particular. The government department which is responsible for water, the Department of Water and Sanitation (DWS, formerly the Department of Water Affairs (DWA)) released the second version of the National Water Resource Strategy (NWRS2) in 2013.7 The NWRS2 adopts a holistic approach towards water management, its availability and its use. More importantly, it defines water resources in a much broader context. It recognises that the potential for the development of conventional surface water resources such as large storage dams are limited and that other water supply requirements reconciliation options need to be implemented such as water conservation and demand management, groundwater, desalination, rainwater harvesting and water reuse. The DWA developed a National Strategy for Water Reuse,8 which provides a considered approach to the implementation of water reuse schemes.
Examples of current South African water reuse schemes
Table 1 lists a selection of recently implemented and currently planned reuse projects at the locations shown in Fig. 1. More description of some of the projects is provided in the following sections.
Table 1 Recent and planned potable water reuse schemes
Location |
Consultant |
Main contractor |
Ml per day |
Product end use |
Main process |
Implementation status |
Ultrafiltration.
Membrane bioreactor.
|
George |
RHDHV |
Aqua engineering (Ovivo) |
10 |
Indirect potable |
UFa |
Implemented |
Beaufort West |
RHDHV |
Water & waste-water engineering |
2.3 |
Direct potable |
Multi-barrier |
Implemented |
Bellville (City of Cape Town, CoCT) |
N/A |
VWS (Veolia) |
20 |
Potential reuse |
MBRb |
Under construction |
Port Elizabeth (NMBM) |
RHDHV |
N/A |
45 |
Industrial reuse, potential IPR |
MBRb |
Feasibility and tender |
Hermanus (Overberg) |
RHDHV |
N/A |
5 |
Direct potable |
Multi-barrier |
Feasibility and tender |
Zandvliet (CoCT) |
TBA |
N/A |
18 |
Potential potable reuse |
MBRb |
Inception |
Potsdam (CoCT) |
TBA |
N/A |
30 |
Potential potable reuse |
MBRb |
Inception |
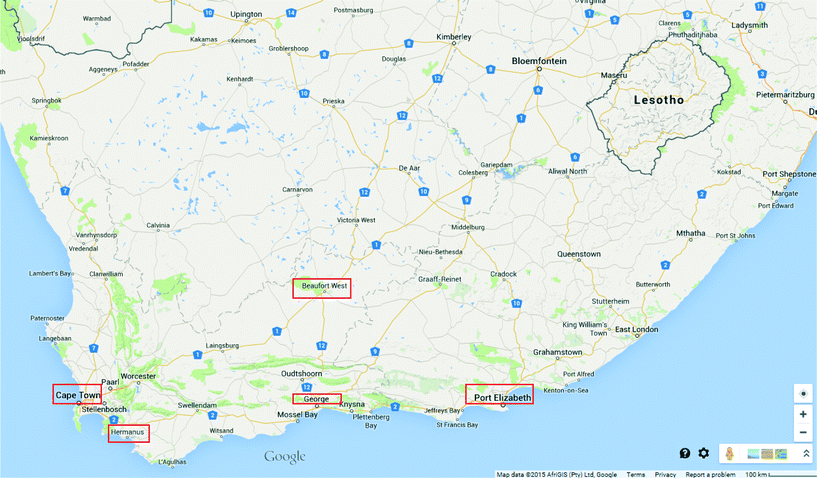 |
| Fig. 1 South African DPR and IPR installations (image ©Google Maps; data ©2015 AfriGIS Pty Ltd). | |
George – 10 Ml per day IPR plant (2009/10)
Context.
As the largest town in the popular tourism region, the Garden Route, George also faced water shortages and had decided on an indirect water reuse strategy where final effluent from its Outeniqua wastewater treatment plant (WWTP) is treated to a very high quality through UF and disinfection prior to being returned to the main storage facility, the Garden Route Dam, where it is combined with current raw water supplies. This initiative augments the existing supply by 10 Ml per day, approximately one third of the drinking water demand.
Process configuration.
Final effluent from WWTP, drum screen, UF, disinfection by chlorination, transfer to Garden Route Dam. Provision has been made for powdered activated carbon (PAC) addition at George WTW, if required as an additional operational barrier.
Product water quality.
Equivalent to Garden Route Dam water.
Beaufort West – 2.3 Ml per day DPR plant (2010)
Context.
The town of Beaufort West shows a significant population growth due to increasing economic activities. The local municipality initialised a project to supply additional SANS241-1:2011 standard water as a result of a shortage of drinking water. The proposed solution for this problem was to build a plant for the reclamation of treated sewage to deliver SANS241-1:2011 water. The reclamation plant is maintained and operated by Water and Wastewater Engineering in a 20 year agreement.
Process configuration.
Multi-barrier concept, final effluent from WWTP, sand filtration, UF, two-stage RO, permeate disinfected by ultraviolet light (UV).
Product water quality.
Better than SANS 241:2006 Class 1 water requirements.
Bellville (city of Cape Town) – 20 Ml per day membrane bioreactor (MBR) potential reuse (under construction)
Context.
The City of Cape Town is in the process of upgrading the existing Bellville South WWTP to extend capacity by adding a 20 Ml per day MBR module. The objective is to extend the design capacity of 50 Ml per day of the existing works in three consecutive 20 Ml per day phases to an ultimate capacity of 110 Ml per day on the available site. MBR technology was chosen for the first 20 Ml per day extension phase due to spatial constraints and the possibility of potential reuse of high quality treated effluent by nearby industrial users.
Process configuration.
Inlet works with coarse and fine screens, degritters, rotary sieves (protection of membranes), activated sludge reactor, separate membrane tank with capillary UF (submerged membranes), sludge dewatering.
Port Elizabeth (Nelson Mandela Bay Municipality) – 45 Ml per day Membrane Bio Reactor (MBR) industrial reuse
Context.
Port Elizabeth is a major growth point in South Africa, in particular the Coega Industrial Development Zone (IDZ). The growth of industries and subsequent population growth within the municipality creates an increased demand for treated water. Nelson Mandela Bay Municipality (NMBM) is also currently affected by severe drought conditions, which place tremendous stress on existing surface water resources. The NMBM and Royal HaskoningDHV (formerly SSI Engineers) are in the process of upgrading the existing Fishwater Flats WWTP to a 170 Ml per day treatment capacity. Planning and design are underway to provide advanced treatment in the form of MBRs and RO desalination plants to supplement the existing water resources and provide the Coega IDZ and NMBM with sustainable industrial and potable water through indirect effluent reuse. The first phase of the water reuse scheme will produce water at 45 Ml per day, which will be suitable for industrial and/or indirect potable reuse with a second phase of similar capacity to follow.
Hermanus – 5 Ml per day DPR plant
Context.
The Overstrand Municipality is experiencing drought conditions, resulting in a shortage of drinking water to the town of Hermanus. Reclamation of effluent for DPR was selected to augment the existing surface water supply. The first phase of the project entails the construction of works to reuse 2.5 Ml per day of effluent with civil works for a future increase in capacity to 5 Ml per day.
Process configuration.
Multi-barrier approach, including UF pre-treatment, RO desalination, as well as advanced oxidation and carbon filtration. The product from the reuse plant will be fed directly into the drinking water reticulation system.
Product water quality.
Better than SANS241-1:2011 water requirements.
While the list above may give the impression that direct water reuse is established practice in South Africa, in reality most if not all of the reuse schemes have developed independently from one another, and hence probably have involved much duplication of effort.
Recent South African research
The focus of attention in the past five years has been the engineering and scientific aspects of product water quality, and how to co-ordinate the national approach to DPR. Recent research has therefore investigated and published a comparison of different process trains for DPR, a decision-support and costing model for DPR schemes, and created an implementation plan for direct and indirect water reuse for domestic purposes.
Wastewater reclamation for potable reuse
The main objective of this research9,10 was to evaluate the performance of different MBRs as pre-treatment step to produce potable water.
Three MBR pilot plants were set up at Darvill WWTP in Durban. Settled sewage was pumped to a 20 kl balancing tank from where submersible pumps supplied each pilot plant. The three plants utilised different membranes: Toray (flat sheet), Norit (tubular) and Pall Corporation (hollow fibre). The Pall Corporation pilot plant was never successfully commissioned and therefore only the Toray and Norit pilot plants produced results. Daily samples were taken and analysed for one year. The MBRs were evaluated in terms of: permeate quality, fouling potential of the permeate, fouling rate (cleaning frequency), stable fluxes, and peak fluxes.
The most important criterion was the composition of permeate, because poor permeate can have a negative impact on downstream processes. The water quality was evaluated in terms of the permeate water quality being able to consistently meet set water quality objectives and standards, including the removal of Contaminants of Emerging Concern (CECs, such as endocrine disruptors, pharmaceuticals, and personal care products), for the production of potable water. Although there were minor differences in product water quality between the processes, most were compliant with the SANS241-1:2011 drinking water standard.11 A parallel bench scale comparison of five different process trains, utilising different combinations of membrane bioreactors (MBRs), reverse osmosis (RO), ultraviolet light (UV), ozone (O3), nanofiltration (NF), and granular activated carbon (GAC):
a) MBR-RO-UV
b) MBR-O3/GAC-NF-UV
c) MBR-NF-O3/GAC-UV
d) MBR-NF-UV
e) MBR-O3/GAC-UF-UV
yielded results demonstrating that streamlined process trains such as MBR-RO-UV (replicating the Singapore process) or MBR-NF-UV are equally effective as treatment trains with additional processes such as ozonation and GAC (MBR-O3/GAC-NF-UV). Cost estimates suggested that the membrane-based process MBR-RO-UV would require less capital investment than process MBR-O3/GAC-NF-UV, the ozone/GAC-based treatment process.
Decision-support model for the selection and costing of direct potable reuse systems from municipal wastewater
Water Supply Authorities (WSAs) face challenges with diversifying the mix of raw water resources they use to provide potable supplies. Numerous options are available when WSAs, the DWS, planners and funders want to improve water surety (and sustainability) or make provision for drought periods. Sufficient information is often not readily available for the planners/authorities to make informed selection of the best options for a specific situation, especially regarding technical, costing, energy and environmental data. Even if the information is obtained, comparison of the best possible options is often not feasible, because of the differences in priorities assigned to the multitude of factors making up the main components of the selection criteria. Swartz et al. (2014)12 created a decision-support system to be used to identify, evaluate, compare and select appropriate options that can be used to produce sufficient quantities of safe drinking water from available water sources. The guide also included the development of a reuse costing model, REUSECOST, and REUSEDSM, a spreadsheet-based decision support system was developed to provide a simplistic method to compare different reuse options using multi-criteria analysis. This model focused on DPR as a water-supply option to augment conventional water source in water scarce areas.
Implementation plan for DPR and IPR for domestic purposes
The NWRS213 defines water resources in a broad context. It recognises that the potential for development of additional conventional surface water resources is limited and that other reconciliation options need to be implemented such as water conservation and demand management, groundwater, desalination, rainwater harvesting and water reuse. The DWS also developed a National Strategy for Water Reuse,14 which provides a considered approach to the implementation of water reuse projects.
The National Strategy for Water Reuse has to date not been broadly communicated and consulted. The consultation process to date focussed on the core NWRS2 document and not specifically on the enabling strategies which include the Desalination Strategy and the Water Reuse Strategy.
The aim of this project was to develop a plan to bridge the gap between the strategy and implementation of water reuse for domestic/potable water use in consultation with the DWS and the WRC. The document was informed by a study of international best practices as well as a locally held workshop on water reuse.
The key strategic interventions recommended in the National Strategy for Water Reuse were unpacked, and recommended actions were developed.15
South African challenges and current research
No South African guidelines exist specifically for water supply authorities currently managing or planning DPR, in terms of what the specific health-based targets are, what to monitor (microbiological, chemical, organic micropollutants, endocrine disrupting compounds and chemicals of emerging concern), and how to undertake the process of public engagement. These uncertainties have precipitated research into creating benchmarks for water quality and operational costs, and into emerging pollutants and social licensing of DPR.
Investigation into the cost and water quality aspects of water desalination and reuse plants
With known feed water and target product water qualities, conventional WTWs are relatively standard and consistent in price. However, the infrastructure in front of (intakes, pre-treatment, etc.) and following (waste discharge, product water pumping systems), and the basic plant building block (membrane system) are major variables in determining the capital and operating costs of the selected solutions. Each location and situation has different advantages and challenges to be evaluated for making the best decisions for implementation. This project will compare and document actual cost and water quality data from various South African projects to establish a first-order knowledge base for desalination and reuse for the augmentation of water supply in a South African context.
Monitoring, management and communication of water quality and public acceptance in the direct reclamation of municipal wastewater for drinking purposes
Water reclamation plants have been constructed and are in operation in Beaufort West (DPR), George (IPR) and Mossel Bay (industrial reuse), while DPR in Durban and Hermanus is at an advanced planning stage. While considerable work has been done globally to provide a regulatory framework, including monitoring requirements, little has been done locally. The main concerns are health risks associated with the consumption of direct reclaimed wastewater. The main aim of this project is to develop a framework for DPR, consisting of health-based monitoring programmes (for compliance and operational barriers, including engineered buffers), funding sources and regulatory approval. The main impacts of implementation of the framework will be improved sustainability of supplementary and alternative drinking water supply to alleviate water scarcity, to improve health and to stimulate economic development.
An investigation into the social, institutional and economic implications of reusing reclaimed wastewater for domestic application in South Africa
Despite people's acknowledgement of water scarcity, the general public often has little knowledge of water and wastewater treatment and distribution. Current literature is almost silent on community awareness and engagement on the issue of DPR. The need to engage communities is a principle enshrined within the South African constitution and is reiterated in the water service regulation strategy, which emphasises the need for citizens' voice. The underestimation of this need cannot be more vividly illustrated than by the recent service delivery protests riddling South Africa, stemming from experiences/perceptions of unsatisfactory service delivery, with drinking water being no exception. This project will provide an understanding of the social, economic and institutional implications and consequences of DPR.
Islamic jurisprudence and conditions for acceptability of reclamation of wastewater for potable use by Muslim users – case study of eThekwini municipality
Recycling of water and water reuse are the key approaches being advocated to deal with the growing constraints of freshwater. None however, is as controversial as DPR, especially amongst the followers of Islam. A recent announcement by eThekwini municipality (Durban) on its intentions to reclaim wastewater for potable use resulted in opposition and rejection from members of the Muslim community. Yet the reality is that many places in South Africa have very little choice and out of need have opted for water reuse. In 2013 the George and Mossel Bay regions of the south coast suffered serious water shortages and used desalination to augment their water supply. The Durban objections are based on the notion that the water does not comply with religious requirements. Questions arise as to whether these sentiments are based on misperceptions: What does Islamic jurisprudence, the Quran and the Sunnah mention? More interestingly, this project is exploring whether the mindset or perceptions and attitudes of Muslims would shift to acceptance of DPR should the facts from an Islamic perspective be found to be supportive or accepting of it.
Emerging contaminants in wastewater treated for direct potable reuse: the human health risk priorities in South Africa
The possible presence of CECs in reclaimed municipal wastewater is of critical concern because of potential adverse impacts to human health. Specific health effects criteria in the evaluation of DPR include (1) primary health concerns of wastewater reuse that are the long term health outcomes of ingesting chemical contaminants found in recycled water, (2) health risks of using recycled water as a potable water supply compared against similar risk by conventional water supplies, and (3) the need for extensive toxicity programs. Much research has been done in Southern Africa and overseas on water reuse. However, to date there are no guidelines for the South African water sector. This project will identify CECs in South African reclaimed potable water, their sources, pathways and receptors, potential risk from exposure to these chemicals, performance of water reclamation treatment systems and risks for DPR in South Africa. Assessment of DPR systems for the removal of contaminants that may have negative health impacts will provide a good basis for the development of South African guidelines for implementation of barriers, monitoring programmes and assessment programmes to eliminate or minimise risks and can improve public acceptance of reclaimed water.
Endocrine disrupting compounds removal by wastewater treatment plants
Increasing attention has been focused on endocrine disrupting compounds (EDCs) as CECs in municipal wastewater. These compounds can have a negative impact on the environment, and in many cases they are not sufficiently removed in WWTWs. Moreover, their destruction and transport into the environment depend on the design and operational characteristics of these treatment systems and on the properties of the chemicals themselves. Insufficient measurements made at various stages within the WWTWs prevent adequate analyses of how each unit process contributes to degradation. Identifying the performance of each WWT process in removing EDCs will therefore assist in optimizing existing treatment technology by developing accurate relationships between operational parameters and removal of EDCs. There is also a need to consider a mass balance approach that takes into account all forms of the compound in both liquid and solid phases. Issues of potential concern in performing mass balances are little known in South Africa context. This project is focused on activated sludge, trickling filters and oxidation ponds. A comparison of these processes will reveal the most effective systems or/and the best performing stage in removing EDCs and how to optimise each stage, thereby addressing several key data gaps on the removal of EDCs in WWTWs.
Australia
In Australia, a range of urban and regional water recycling projects have been built to extend the communities initial investment in the infrastructure to collect and treat wastewater, and these projects reflect the highly urbanised and near-coastal population distribution within the country (Fig. 2).16 Although implementation of the projects commenced slowly, growth in water recycling has been almost exponential in the last 20 years as a result of a change in how water is valued by the community (related to the decline in rainfall, Fig. 3), planning to accommodate population growth and as a means of ensuring that growth can continue in areas where local fresh water supplies cannot cater for current or future demands.
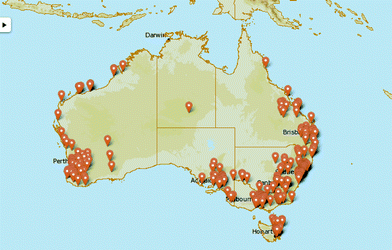 |
| Fig. 2 Water recycling locations around Australia. Reproduced with permission from AWRCE. | |
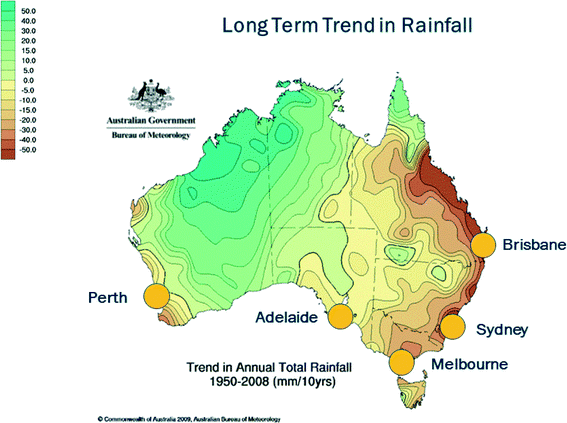 |
| Fig. 3 Annual total rainfall trends in Australia between 1950 and 2008 (© Australian Government Bureau of Meteorology). | |
From the late 1970s to the early 1990s, water recycling was promoted in Australia as an alternative to the discharge of wastewater into receiving waters.17 As the level of treatment required for discharge to the environment increased, it was recognised that it would be beneficial to use this water resource for the irrigation of crops, pasture and public gardens as well as in the maintenance of sporting fields and recreational areas. By the mid 1990s the first recycled water scheme to supply water directly to industry was commissioned at the largest power station in New South Wales (NSW), while the release and development of land for new residential developments in NSW and Victoria included provisions for a third pipe to deliver water from a centralised recycling facility to individual domiciles for outdoor use and toilet flushing. From around 2000 to 2002, which was the start of approximately seven years of drought in eastern Australia, the effects of population growth coupled with less predictable and declining yield from dams and reservoirs accelerated the development of water recycling schemes.18
During this period the motivation was to develop schemes that offset the need to supply water from the potable distribution system. The result was an increase in schemes supplying the petrochemical, building material and paper industries and third pipe schemes for both suburban Greenfield developments as well as new urban in-fill developments. In addition, recycling schemes were implemented to offset the release of surface water stored in dams and impoundments to maintain environmental flows in rivers and recharge aquifers.19
Water recycling for drinking in Australia
In addition to being the driest inhabited continent, from approximately 2000 to 2008 Australia's ‘millennium drought’ put planned recycling for augmentation of potable supplies on the agenda of communities in both metropolitan and regional Australia. Replenishment of surface waters or groundwater using water from a water recycling plant is referred to in Australia as Indirect Potable Reuse (IPR). Under an IPR scheme the water will eventually be used for drinking water, however it will be supplied indirectly through the source water storage and will receive additional treatment in a water treatment plant prior to distribution. The alternative to IPR is Direct Potable Reuse (DPR), where the water is blended into the drinking water being supplied to the consumer through the distribution system.
Indirect potable reuse in Australia
Indirect Potable Reuse has been considered for regional communities in Goulburn, NSW, the Australian Capital Territory (ACT) and Toowoomba, Queensland.20 The need for the IPR project in Goulburn was obviated by the NSW Government's decision to construct a water transfer pipeline, while the proposed IPR project for the ACT did not proceed past the planning study. In the case of Toowoomba, however, the project moved through the planning phase and attracted support from the Australian Government for A$22.9m of the A$67.8m total project cost, subject to community support in a referendum. The community, however, voted 61% to 38% against the project and the IPR project was abandoned.
Two purpose built IPR schemes have since been constructed in the Australian capital cities of Brisbane and Perth. The Western Corridor Recycled Water Scheme in South East Queensland was designed and built to produce drinking quality water suitable for release into the Wivenhoe Dam, Brisbane's principal water storage.21 The scheme consisted of three advanced water treatment plants which drew feedwater from six nutrient removal wastewater treatment plants. Each of the three advanced water recycling plants incorporated microfiltration (MF) and RO followed by an advanced oxidation system using UV light and hydrogen peroxide to remove specific disinfection by-products and non-specific low molecular weight organics.
The project had a production capacity of 232 ML per day and over 200 km of interconnecting and product water delivery pipelines. The project was delivered during the later stages of the millennium drought when the dam levels ranged from 17 to 30%, however, significant rains in early 2008 raised dam levels to over 40%. This change in dam levels, combined with a perceived reduction in public support for IPR prior to a November 2008 election resulted in a decision not to augment drinking water supplies until dam levels again fell below 40%. For several years the scheme operated at reduced capacity supplying reclaimed water to two major power stations, and is now being decommissioned.22
In Perth in Western Australia, the Western Australia Water Corporation operated a three-year demonstration project investigating the feasibility of reclaiming water from the Beenyup WWTP using membrane filtration, RO and UV disinfection prior to injection into a sub-surface aquifer. The demonstration concluded in 2012, and in 2013 the Western Australian Government agreed to a full-scale groundwater recharge scheme, which commenced construction in 2014. When complete, the full-scale facility will provide 14 Gl per annum to the aquifers supplying Perth's drinking water, with the option to expand to 28 Gl per annum in the future.
Direct potable reuse in Australia
While there are currently no full scale DPR schemes operating in Australia, the Australian Antarctic Division is investigating the option of installing a potable reuse scheme at its Davis research base in Antarctica. To enhance the quality of the marine discharge from the Davis WWTP, a number of different, proven technologies have been selected. The advanced waste water treatment plant comprises several advanced oxidation technologies including O3, UV and chlorine as well as UF, AC filtration and RO. A demonstration plant had been commissioned at a wastewater treatment plant in Tasmania, and monitoring and evaluation of reliability and treatment efficacy are currently occurring. At this stage no decision has been made about whether the water produced by the plant will be reused at Davis Station or simply discharged to the ocean. This decision will be made in the future after extended consultation with Australian Antarctic Division staff and expeditioners as well as independent assessment and regulation of the technical solution.23
Australian challenges to water recycling for drinking
In the last 20 years there has been considerable progress in expanding the scope and capacity for water recycling in Australia. There are, however, challenges to the continued development and expansion of water recycling for drinking applications, and it is important that they are identified and addressed in order for the full potential of this supply source to be realised.
In 2010 and again in 2012 the Australian Water Recycling Centre of Excellence (AWRCE) worked with Australia's government, utility, private enterprise and research sectors to identify some of the key national challenges facing the water recycling sector.24 Examples of such challenges include the current institutional capacity to deliver operate and manage water recycling schemes, poor understanding of the cost benefits of water recycling, the lack of a national/international database (or warehouse) of water recycling schemes, variable application of national guidelines, uniform and reliable analytical capacity, social and political issues and concerns. The results of these discussions are briefly summarised in a number of themes below:
Technology, efficiency and integration
The century-old concept in urban and rural water system design has been to maintain a strict separation of clean water (drinking water) and “dirty water” (wastewater) to ensure minimal human health risks due to pathogens and other contaminants crossing from the so-called dirty to the clean water stream. Over the years, the treatment technologies in both streams have improved considerably, to the point where it is now technically feasible to create water of a quality from a wastewater stream that is equivalent or superior to that of the traditional ‘clean water’.
Water recycling technologies therefore sit between the drinking and wastewater streams, as they are (typically) using wastewater as a source and generating a water quality that can be used to either replace or augment drinking water supplies. It is therefore critically important that these “new” water treatment technologies work most efficiently for the end-uses for which they are designed, and that they integrate optimally into that space between the clean and the dirty water streams.
Some of the key challenges in this Theme are seen as:
1 Improving on-line monitoring of water qualities at all stages within a water recycling scheme (source control to final product water).
2 Optimisation of existing process technologies and trains.
3 Optimal integration of water sources, users and technologies to achieve most suitable outcomes with least impacts.
4 Development of innovative technologies for water recycling.
Water quality, risk and scheme validation
The development of the Australian Guidelines for Water Recycling in 200825 provided the basis for application of a preventive risk management approach, and the principles and a framework for safe implementation of water recycling for drinking projects. The guidance, however, is not prescriptive and, within the bounds of ensuring safe drinking water, allows for flexibility in application. These national guidelines have been developed through expert scientific and industry input and have been adopted by all states and territories in Australia.
Notwithstanding the success of the guidelines, a challenge to achieving wide-scale uptake of water recycling, particularly with high quality reuse schemes such as potable reuse, has been defining (amongst practitioners, regulators and the general community) the acceptability of the risk associated with the intended form of reuse. In addition, industry validation of such schemes can be a complex and costly exercise.
Some of the challenges in this theme include:
1 Understanding acute risk from pathogens including identification, measurement and validation of their removal.
2 The risks and toxicity associated with chemicals and transformation products (single chemicals and mixtures).
3 Determining the minimum log removal for a whole treatment train that achieves risk minimisation and is not overly conservative.
Social, institutional, and economic
Successful development, implementation and continuing support for water recycling schemes will involve effective but fluid interactions between industry experts, policy makers, financial institutions and the community. To be effective will require the arguments and tools for a prioritised investment in alternate water supply portfolios, building decision support for innovative policy design and implementation, and developing implementation and evaluation programs to ensure community and industry acceptance of water recycling for drinking.
The challenges in this theme are often interdependent, and relate to the social and economic opportunities and constraints inherent in the relationships within and between government, industry and the broader community.
Some of the challenges in this theme include:
1 Understanding the direct and indirect (lifecycle) costs and triple bottom line cost effectiveness of recycling relative to other water supply investment options.
2 Clarifying and enhancing the institutional basis of public confidence in water and recycled water management.
3 Establishing the most effective methods and modes of communicating about water recycling to the public.
Current Australian research initiatives
In addition to the University research sector, Australia has several national organisations currently involved in research into urban water. They include Australia's peak organization representing water utilities, Water Services Association of Australia,26 Water Research Australia,27 the National Centre of Excellence in Desalination Australia,28 and Australia's national research agency the CSIRO.29
Guided by the discussions with industry and its strategic research plan, and funded by the Commonwealth Government, the AWRCE partnered with these organisations to invest in industry lead research initiatives to address a number of the challenges outlined above.
Two national priorities for investment that were identified by the industry and which would build confidence and support for water recycling schemes were firstly, to develop a nationally consistent approach to validation of water recycling technology; and secondly to invest in tools to assist proponents engage with the community on water recycling for drinking.
Consistent validation of technologies
Validation is confirmation that a treatment technology meets specific performance targets. Currently, there is no consistent approach to validating treatment technologies against the Australian Guidelines for Water Recycling, making existing recycled water scheme validation slow, complex and costly due to differences regarding the validation required to gain regulatory approval across jurisdictions. This project aims to deliver a national framework for validating treatment technologies that could have application in other countries. The project involves collaboration between Australia's regulatory, utility and private enterprise sectors to develop and implement an agreed national approach, and a series of research projects designed to develop protocols for validation of key technologies.
Engagement with communities
Despite increasing pressure on traditional potable water sources, there are still major challenges to successfully engaging with the community and stakeholders around water recycling for drinking. This project has investigated how to address these challenges and has developed high quality, evidence-based resources for community, government, media and industry. The project involved three research streams. Stream 1 focused on the technical aspects and performance of existing water recycling. Stream 2 focused on the social science related to community perceptions, and beliefs and factors that influence community decision making, as well as communication and regulatory aspects related to using recycled water for drinking. Stream 3 has produced a variety of products for education and communication with communities that are taking the journey towards water recycling for drinking.
Additional projects
In addition to the two significant national initiatives above, the Centre and other project partners are funding research into assessment of chemical mixtures, demonstration trials of direct potable reuse with the Australian Antarctic Division, and has released a summary of the state of direct potable recycling through the Australian Academy of Technological Sciences and Engineering.30 The Centre has also initiated several information and knowledge-based projects, such as a national water recycling and desalination website and database in partnership with the Australian Bureau of Meteorology, NCEDA and CSIRO.31 The website provides data on Australia's production and use of recycled water and desalination.
The United States of America
The US is diverse in its water availability and water management challenges, directly impacting the increased trend in the implementation of potable reuse over the last three to five years. Clearly the strongest driver is found in the Sun Belt region from Texas to California where water availability has significantly decreased as a result of record droughts (Fig. 4). The drought has left communities in situations where there are no alternatives except to implement potable reuse. While the South-eastern part of the country receives over 1500 mm per year of precipitation, they lack upland storage and are challenged by a flat topography, dense populations, and the potential for flooding, resulting in significant loses of freshwater to tide. The resulting over pumping of aquifers to maintain adequate water supplies has secondary impacts on critical environmental systems. And in areas where discharge limits drive the advanced treatment of traditional wastewater disposal into rivers and streams, the cost of such treatment is prohibitive given more appropriate uses for such water. In addition, populations continue to rise all over the country, driving the need for water supply diversification for urban water supplies, as well as agricultural and industrial supplies.
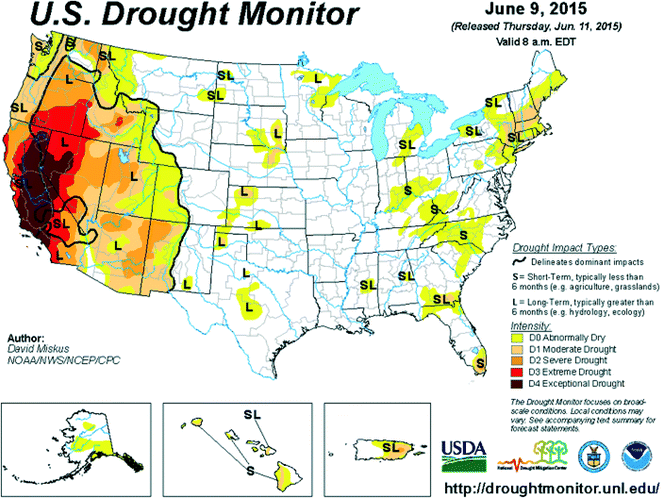 |
| Fig. 4 Reproduced with according to USDA conditions of use, United States Drought Monitor.36 | |
There is no national regulation of reuse in the US. Most individual states have developed regulations for non-potable, IPR, and/or DPR, as seen in Fig. 5. The US Environmental Protection Agency (USEPA) has developed Water Reuse Guidelines, most recently updated in 2012. It is a comprehensive document summarizing state's status and regulations, practices, and case studies. A special addendum on Potable Reuse is expected in late 2015. Additionally, with support from Water Environment Federation and American Water Works Association, WateReuse commissioned a national DPR Framework to provide context for states interested in potable reuse as a means to extend their drinking water supply. It includes issues such as public health protection, sufficient multiple barriers, risk assessment, water quality monitoring, and operation management that states can use to develop guidelines for Direct Potable Reuse.
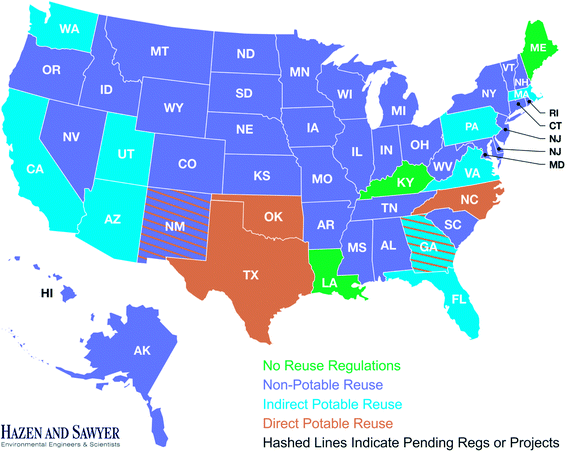 |
| Fig. 5 State of reuse in the US. Reproduced with permission from Hazen and Sawyer. | |
Potable reuse will be an essential component of a future water portfolio that must meet the needs of the projected 2030 California population of 50 million32 and USA population of 360 million.33 According to one estimate, reusing the country's 45 Gl per day of wastewater effluent that is discharged to the ocean could supply 27% of public water supply.34 A research study funded by WateReuse forecasted the amount of highly treated wastewater in California that will be discharged to the ocean or to inland waterways in 2020 that could instead be used for potable supplies. This amount (3800 Ml per day) is sufficient potable water to supply all municipal needs (including commercial and industrial uses) for over eight million Californians, or roughly one-fifth of the state's projected population for 2020, as seen in Fig. 5. It also determined the economic sensibility of potable reuse, in that it is cost competitive and often cheaper than alternate supply sources such as desalination, imported water, non-potable reuse,35 not to mention requires far less energy.
Examples of potable reuse in the USA
Indirect potable reuse
Indirect potable reuse has been practised in California since 1962 (Montebello Forebay) and since has expanded across the nation. The Upper Occoquan Service Authority in Virginia has been augmenting its surface water supply with recycled water since 1978.
The Groundwater Replenishment System (GWRS) water purification facility operated by the Orange County Water District (OCWD) has proven to be the gold standard for potable reuse. In operation since 2008, the plant uses a number of proven technologies, including MF, RO, and advanced oxidation. The resulting purified water meets or exceeds US potable drinking water standards and because all unregulated chemicals known or suspected to be of health concern are reduced to non-measurable or de minimis levels, the water is considered to be safe for direct human consumption. In California alone, there are seven permitted IPR facilities producing 244
100 Ml per year (197
916 acre feet per year) and dozens more scoped to produce 387
700 Ml per year (314
306 AFY) in planning.37 These and other IPR facilities have laid the groundwork and been the catapult to enable the removal of the environmental buffer and practice DPR.
Direct potable reuse
The first operational DPR sites in the USA are in Big Spring and Wichita Falls, both in Texas. The treatment technologies employed at these locations have been accepted by various regulatory authorities as being able to reliably produce safe potable drinking water, and the implementation of these projects has been accepted by the public. Other states are actively pursuing DPR, either through assembling expert panels, developing regulations, and/or launching pilots, including California, Georgia, North Carolina, Oklahoma, and New Mexico (Fig. 6).
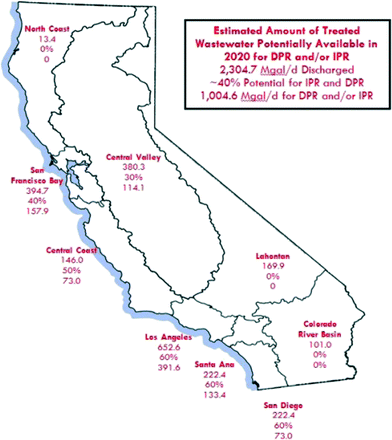 |
| Fig. 6 Potential of potable reuse in CA, adapted with permission from WRRF-14-08. | |
Current research in the USA
Indirect potable reuse has been successfully practised for decades, paving the way for DPR. As we move from IPR to DPR, we must recognize and quantify the benefit of the difference. IPR has the physical and psychological safety net of the environmental buffer, which offers dilution, blending, and natural attenuation that occur as water passes through an aquifer or a reservoir. Maybe more importantly, the environmental buffer provides time to detect and respond to treatment failures, something that may be significantly reduced in DPR. However, research suggests that enhanced treatment and monitoring can replace the need for retention time in environmental buffers, while still protecting public health.38 An illustration of this concept (Fig. 7) shows the variations of treatment, monitoring, and retention time in IPR and DPR, both equally protective of public health. Reliability in DPR requires robust and redundant treatment and monitoring – key to its success and a major research focus.
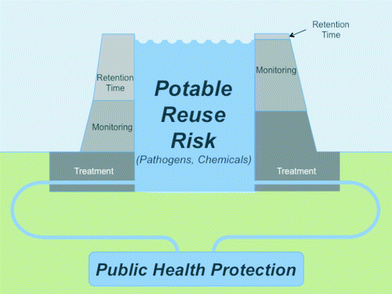 |
| Fig. 7 Illustration of IPR (left) versus DPR (right), used with permission from Trussell Technologies. | |
The WateReuse Research Foundation (WRRF) has identified DPR as a key component of future water supplies. In response to Senate Bill 918 in California, in 2012 the Foundation joined WateReuse California to launch the Direct Potable Reuse Initiative. This initiative seeks to expand the use of recycled water within California, and to aid regulators in understanding the feasibility of developing criteria for DPR. To address knowledge gaps and concerns from regulators, utilities, and customers, WateReuse has raised more than US$6 million for DPR research.
The California State Water Resources Control Board (SWRCB) has set up an Expert Panel under its Division of Drinking Water Programs (DDW) to make a recommendation on the feasibility of developing DPR criteria by 2016. All of the research under the Foundation's DPR Initiative is made available to the DDW Expert Panel for their consideration as they navigate the important task. To date, the Foundation has allocated US$6 million to fund 34 DPR research projects, a program valued at US$20 million and counting.
The WRRF's research efforts have focused on a variety of topics relating to the regulatory, utility, community concerns with DPR (Table 2). Included among the WRRF's research work are projects investigating the reliability and effectiveness of various treatment processes in their ability to remove pollutants and other impurities from a wastewater stream. The WRRF is also looking at the critical control points within a treatment system to determine what stages of the treatment process are most vulnerable. Additional work is looking at real-time monitoring systems to allow operators to detect abnormalities in the treatment system and develop solutions to such anomalies before they become a public health risk. The goal of a follow on project is to interpret and manage the vast qualities of data produced by sensors and monitors.
Table 2 Intensity of research focus on different research areas at WRRF
Research area (projects can cover multiples areas of research) |
No. of WRRF projects |
DPR vs. alternatives, economics |
4 |
Evaluation of potential DPR trains |
14 |
Demonstration of reliable, redundant treatment performance |
19 |
Critical control points |
5 |
Pathogens: surrogates, credits |
16 |
Pathogens: rapid/continuous monitoring |
10 |
Failure and resiliency |
10 |
Public perception and acceptance |
3 |
CEC removal and risk |
9 |
Operations training, framework |
5 |
Source control |
2 |
Much of the WRRF's DPR research is based on the “4R” concept (reliability, robustness, resiliency, and redundancy), fully explored by a DPR demonstration project in San Diego led by Trussell Technologies. This project leverages the state of the science and lessons learned from IPR and gathered an expert panel to develop an experimental plan for DPR. The “4Rs” are the basis: Reliability is built through the use of redundancy in both treatment and monitoring to ensure that treatment goals are more reliably met or more reliably demonstrated. Robustness allows the system to address a broad variety of contaminants—both known and unknown—while also preventing the occurrence of catastrophic failures. Responding to failures that may occur without needing to shut down operation is explored through resilience.39
While in California, the potable reuse treatment train of choice includes RO (along with microfiltration and advanced oxidation with ultraviolet light), this is not ideal for inland communities. Alternate advanced options are being studied to ensure they also meet public health requirements. Numerous projects, including a project in Gwinnet County, GA being launched summer of 2015, investigates a process including granular activated carbon (GAC) and ozone.
From the viewpoint of utility operations, WRRF research is exploring the unique operations and maintenance (O&M) needs a DPR system may face compared to traditional wastewater and drinking water treatment facilities. Requirements for engineered storage as well as blending with traditional sources of drinking water is also being investigated, acknowledging that more than just an effective treatment system is needed to fully integrate potable reuse into a complete drinking water treatment and distribution system. Aside from technology and engineering needs, economic issues associated with DPR are also being studied to help and empower utilities and communities to make informed water supply planning decisions.
Addressing community concerns represents a significant challenge in achieving the goal of widespread public acceptance for DPR. A large DPR outreach effort was initiated at the WRRF to develop community and state plans to foster DPR acceptance (these were tailored to CA, however are applicable across nation and world). Findings from an extensive literature review and one-on-one meetings with water and health professionals, legislators, and special interest groups were used to develop a set of messages, which were tested in focus groups and telephone surveys in two model communities. Public acceptance of potable reuse can be achieved by implementing a coordinated, consistent and transparent communication plan. The next phase of this effort is underway to develop messaging material and tools for implementation of the communication plans developed in phase 1, such as pamphlets, PSAs, and videos which are being prepared and will be available on a new WateReuse website with an expanded education/outreach focus. Included here will be a global map of potable reuse, developed originally by AWRCE and expanded by WateReuse.
Research needs and challenges in the USA
Water quality and public health
Direct potable reuse relies on a multi-barrier approach to remove contaminants, both microbiological and chemical. Microbes can present an acute health risk, and detection and removal is well established through surrogates. Increased sensitivity will benefit the industry, and molecular methods and genomic mapping may expand microbial monitoring and detection in the future. On the chemical side, current and future known and unknown chemicals may pose a greater challenge than pathogens to assess and manage. We currently have analytical methods to measure many chemicals at trace levels, but tying this to risk is not well established. Thousands of chemicals are being introduced annually, so monitoring many individual chemicals is difficult and expensive. There are unknown individual chemicals, mixtures, and disinfection by-products (DBPs) to understand. The chemical-by-chemical risk paradigm will likely be outpaced by the discovery of new chemicals, and is not intended to address chemical mixtures, some of which cannot be monitored using currently available methods. Therefore more research on the use of non-traditional monitoring, including bioanalytical screening tools and other techniques that may be able to detect unknowns and mixtures should be explored, while linking it to risk, to potentially supplement current monitoring.
Operations and training
Effective operations are critical for any treatment plant, and will be especially crucial for the success of DPR due to its minimal response time. Additionally, potable reuse employs advanced treatment technologies and monitoring that require sophisticated training and operation. Several entities including WRRF have projects/committees underway to develop operational O&M plans, training, and certification for DPR., It is important to work together to standardize and disseminate the O&M plans and ensure that operators are well trained and certified.
Public acceptance
The public has come a long way in terms of acceptance of recycled water for non-potable purposes, and that is thanks to the comprehensive outreach campaigns of their local utilities. With IPR, the environmental barrier often comforts people (while in reality it in fact can degrade the water quality). Direct potable reuse is a concept where the ‘yuck factor’ can be overwhelming for some. But with the right people communicating the right messages to all communities, paying special attention to those who are most reluctant and vocal, DPR has a great chance of being embraced as a sensible, safe source of supply.
Discussion and conclusions
The real value of water is often underestimated, and only apparent when there is a risk of running out of supply or a demonstrated commercial benefit. This is illustrated by projects such as the reuse initiative of Durban Water Recycling,40 the Western Corridor Project in Brisbane, or the value of reliability studies at WRRF. The drivers for increasing IPR and DPR in all three of the countries considered have been common: shortages of water in the exact locations where demand for potable quality water exceeds supply; the uniqueness of water's diseconomies of scale, inasmuch as the more volume of water is required, the further one has to go to get it; the corollary economic comparison of reuse of wastewater versus discharge to the receiving environment; and the increasing demand nationally as the human population both increases and becomes more urbanised.
At a national policy and planning level, the three countries have slightly differing approaches to providing guidance and regulatory oversight to potable recycling projects.
South Africa has elected to implement the SANS241 national standard for potable water supply regardless of the source of the raw water. Using health risk – based standards and approaches similar to those set out in the World Health Organization's Guidelines for drinking-water quality,41 the national monitoring and evaluation demand that a set list of determinands be measured in water entering the bulk supply system at set intervals, depending on the size of population or population equivalents being served by the supply. Direct and indirect reuse projects are subjected to a full environmental and social impact assessment (ESIA) including broad consultation with the affected communities and stakeholders. This process, supported by a rigorous regulatory approval, licensing and review of the technology used ensure that the public health and safety are guaranteed.42
Under the Australian constitution, much of the policy, planning and regulatory responsibility for water rests with the States, not the Commonwealth Government. To ensure there is a common, shared national approach to the protection of public health and safety, Australia has developed an agreed risk based approach to managing and protecting public and environmental health under a National Water Quality Management Strategy (NWQMS).43 The Water Quality Management Framework, used in the NWQMS outlines a step by step process for planning, implementing and managing water quality projects, including water recycling, and has been adopted into practice by all Australian States and Territories.
In the US, there is no national regulation of reuse; however USEPA has issued Water Reuse Guidelines,44 updated several times since its debut in 1980. States have developed their own regulatory frameworks for reuse to protect public health, administered by their given environmental/water regulatory body. Most states have non-potable reuse regulations in place, and many have or are exploring potable reuse regulations.
Notwithstanding the various policy and planning approaches, the USA, Australia, and South Africa appear to be focusing their current research resources on at least four common areas (in no order of priority):
1 The cost/benefits of potable reuse.
2 Appropriate testing, monitoring and evaluation to ensure consumer safety from acute (pathogen) risks.
3 Detection, monitoring and assessment of emerging contaminants of concern (CECs).
4 Public acceptance and social consequences of potable recycling projects.
Cost/benefits
As a relatively new initiative in all three countries, the costs and benefits of potable recycling are still poorly understood compared to the traditional water supply sources. Developing an improved costs and benefits understanding to inform optimization of treatment train design under local conditions, potential operating efficiencies associated with chemical and energy inputs or recovery, and optimization of the traditional wastewater treatment to complement and in future seamlessly integrate with water recycling are examples of common challenges. Further developing and applying Triple Bottom Line methodologies to characterize and evaluate potable recycling relative to other water supply options will continue to be an important tool in moving water recycling from being seen as a drought relief option to a mainstream water supply source on par with surface water storages and groundwater supplies.
Acute risks
Under its national Guidelines approach, Australia has adopted health based targets to set and assess the performance of its water treatment infrastructure, South Africa has a single potable water regulatory requirement (in SANS241-145 and SANS241-246), and the US has both national (e.g. USEPA UV) and state reuse (e.g. California regulations) guidance on the use of technologies in water and recycled water treatment. A common driver in all countries is the need to consistently and transparently demonstrate and monitor an appropriate reduction in pathogens across the treatment processes used to produce potable water. Australia has recently initiated a significant research program designed to validate water treatment technologies for pathogen reduction which is complemented by technology validation research currently sponsored by WateReuse Research Foundation in the United States. There is clearly increasing opportunities for countries to share their research about technology validation, but it will require close partnerships between groups internationally to ensure that consistent approaches to the interpretation and application of the findings occurs.
Chemicals of concern
The current research areas of all three countries places strong emphasis on addressing micro pollutants and emerging contaminants. One hundred thousand (100
000) new chemicals are developed annually, and only 2000 are being analysed for the impact on the aquatic environment and health. The current methods of detection are perceived as inadequate in the face of a rapidly increasing number and diversity of CECs, and research is turning more and more to alternative monitoring and evaluation paradigms, such as direct toxicity assessment or whole effluent toxicity measurement, quantitative structure–activity relationship models (QSAR models), and the measurement of activity rather than concentration of contaminants. The use of bioanalytical tools to characterize source waters, optimise treatment processes, and assess product water quality for human health outcomes appears to be a rapidly developing field of interest across all three countries.
Communication and engagement
The rapid development of cost-effective technology options, and our ability to sequence treatment technologies to treat waters of varying biological and chemical quality is increasing the industry's confidence that the treatment of wastewater is a viable technical option to service future water supply needs. Communicating with and engaging the broader community in a discussion of the role of potable reuse in our water future is still, however, a significant challenge across the three countries. Social research is increasingly suggesting we need to enhance our efforts to explain the fundamentals of the water cycle as we engage in discussions about the needs, risks and benefits of potable reuse. Developing communication and engagement tools and processes to help communities, politicians, governments and industry take the journey to potable recycling will continue to be an important research need for some time to come.
Research to innovation to impact
The commonalities in the current research and topics of greatest interest demonstrate that numerous opportunities for international co-operation exist and could be extremely beneficial to the research community and in terms of socioeconomic development and human and environmental health protection. Appropriate testing, monitoring and evaluation systems, benefit cost assessments, CECs, and the anthropology of social acceptance for DPR and IPR are universal, and as such present a unique opportunity for progress on a truly global level. All three of the countries considered in this paper have reported an innovation gap in the water sector, specifically relating to the scale-up and implementation of newly developed water treatment technologies and processes. The gap exists between laboratory scale research and small scale piloting of technologies,† and the end point of full scale operation, whereby new technologies are adopted by the market. This creates a situation where, at times, the sector is forced to import market ready solutions from other sectors, such as oil and gas, because of this innovation gap in our own sector. As such, the issue of water technology and process scale-up, implementation, and commercialisation was identified as a key area of challenge.
This challenge is expressed in a diversity of ways in international innovation literature. The public literature tends to focus on the issues of commercialisation gaps and challenges. In 2002 Rice et al. studied the challenges of transitioning discontinuous (as opposed to incremental) innovation projects to commercialisation and suggested several propositions for bridging the innovation gap.47 They reported that “a substantial ‘readiness gap’ exists between the [R&D] project teams and the receiving business units and that bridging this gap was more difficult than anticipated”. They identified seven propositions “for improving the effectiveness of transition management”, suggesting the potential usefulness of the following managerial approaches: conducting a transition readiness assessment; assembling a transition team; establishing an oversight board; developing a transition plan; providing transition funding from corporate sources; laying the groundwork for a big market; and engaging senior management champions.
Later, Slater and Mohr48 proposed that “in order to successfully develop and commercialize innovations, not only does the firm need to conceptualize and develop the innovation in the first place; it must also be successful in reaching more than just a niche market of innovators–early adopters. In other words, it must overcome the innovator's dilemma as well as cross the chasm. These two problems are faced by all firms—but especially those operating in high-technology markets or driven by technological innovations—and are related in that they both derive from the underlying skill set the firm brings to its marketing strategy.” Arundel and Bordoy49 concurred that “It is a common perception that European public-funded research fails to commercialize their discoveries, in contrast to the perceived success of their American counterparts. This resulted in policies aimed at improving the commercialization of European publicly-funded research, including the establishment of Technology Transfer Offices (TTOs)”.
All of these previous workers demonstrated that the issue of innovation gaps which exist in subtly different ways in differing contexts, are real in many parts of the world. The current mismatch between the end of the road for ‘pure’ research, and the first step on the path of full scale industrial development poses challenges to policy implementation, particularly where environmental authorisations are concerned, and consequently temporary relaxation of effluent discharge standards or the implementation of percentile compliance is one of the many possible positive actions that can be taken to provide enabling regulatory environments to de-risk full scale experimental work. In the current context, what will the role of research, and consequently technical journals, be? One possible answer is to increase peer reviewed publication of full scale science and engineering that can be used to exemplify good science, performed at scale, in an uncontrolled environment. Another, arguably more productive answer is to increase the mobility of staff and students between the academic and industrial research communities, so that scientific rigour and applicability of research are both promoted and maintained. Research information is of great importance for practitioners in the field of water treatment and water management (consultants, operators, regulators, administration experts, researchers and developers) and should encourage innovation. In this context “innovation” is a successful full scale application of a new development from an idea to full scale.
Research opportunities therefore exist outside the water sector, too: extending smart solutions and new approaches and increasing awareness and education (water cycle, water use hierarchy, resource efficiency/recovery and water reuse), e.g. through multi-industry fora, and cross-sectoral sharing, even outside the water space, may assist in the transfer of new knowledge and technology from research into practice.
The matter of changing monitoring and evaluation practices and as a corollary, evidence-based regulation, presents an opportunity for international collaboration, as well as collaborative work by academic researchers and water services providers in driving conservative but pragmatic regulatory measures. The engagement of the research community with the water treatment and supply industry remains the key aspect which is often missing from national programmes: practitioner feedback is essential in designing practicable monitoring systems, and academic input is necessary to inform the sector of cutting edge measurement and monitoring methods without the influence of commercial drivers. Universities, R&D organisations and research councils can play an important role in honest brokerage, especially when it comes to the independent evaluation of equipment, instruments or guidance.
Community engagement, industry guidance and regulatory development for potable recycling will increasingly demand closer co-operation between the water utility, industry, research and regulatory sectors in each country. With potable reuse being relatively novel in all three countries, the development of evidence-based, practical approaches to realising the economic, technical and social opportunities of potable reuse, also presents an opportunity for international collaboration. National organisations such as the Water Research Commission, Australian Water Recycling Centre of Excellence and WateReuse Research Foundation have a responsibility to play an independent facilitation role within their own countries, but the water sector should have an expectation that they are brokering more effective outcomes internationally as well. This paper and the collective discussions that preceded it have helped identify areas where the three organizations are now focusing their discussions on collaborative opportunities.
Acknowledgements
The authors gratefully acknowledge the technical and cost data, and other information provided by the WRC, WRRF, and AWRCE.
References
-
http://www.globalwaterintel.com/press-releases/ (Accessed 17 May 2010).
-
http://www.globalwaterintel.com/press-releases/ (Accessed 17 May 2010).
-
http://www.aquarec.org/ AQUAREC Final Report, p35, (Accessed 17 May 2010) 2006.
-
T. Wintgens, D. Bixio, C. Thoeye, P. Jeffrey, R. Hochstrat and T. Melin, Reclamation and reuse of municipal wastewater in Europe – current status and future perspectives analysed by the AQUAREC research project. Policy Brief, http://www.iwrm-net.eu/sites/default/files/Aquarec_Policy%20Brief_final_A4.pdf (Accessed 25 February 2015) 2006 Search PubMed.
-
SANS 241–1:2011 Drinking water Part 1: Microbiological, physical, aesthetic and chemical determinands, South African Bureau of Standards, Pretoria, South Africa, 2011 Search PubMed.
-
NPC, National Development Plan 2030, Available at https://nationalplanningcommission.files.wordpress.com/2015/02/ndp-2030-our-future-make-it-work_0.pdf (Accessed 25 February 2015) 2011 Search PubMed.
-
DWA. National Water Resource Strategy. Water for an Equitable and Sustainable Future. 2nd Ed. Department of Water Affairs. Available at: https://www.dwa.gov.za/nwrs/LinkClick.aspx?fileticket=u_qFQycClbI%3d&tabid=91&mid=496 and https://www.dwa.gov.za/nwrs/NWRS2013.aspx (Accessed 25 February 2015) 2013.
-
DWA, National Strategy for Water Re-use, Available at: https://www.dwa.gov.za/nwrs/LinkClick.aspx?fileticket=WIXBU9U2xzg%3D&tabid=91&mid=496 (Accessed 25 February 2015) 2011 Search PubMed.
-
G. Metcalf, L. Pillay, C. Murutu, S. Chiburi, N. Gumede and P. Gaydon, Wastewater reclamation for potable reuse Volume 1: Evaluation of membrane bioreactor technology for pre-treatment, Research Report no. 1894/1/14, Water Research Commission, Pretoria, South Africa, 2014. Also available online at http://www.wrc.org.za/Pages/DisplayItem.aspx?ItemID=11079&FromURL=%2fPages%2fKH_AdvancedSearch.aspx%3fdt%3d1%26ms%3d%26d%3dWastewater+reclamation+for+potable+reuse+Volume+1%3a+Evaluation+of+membrane+bioreactor+technology+for+pre-treatment%26start%3d1 Search PubMed.
-
G. Metcalf, L. Pillay, L. C. Murutu, S. Chiburi, N. Gumede and P. Gaydon, Wastewater reclamation for potable reuse Volume 2: Integration of MBR technology with advanced treatment processes, Research Report no. TT611/14, Water Research Commission, Pretoria, South Africa, 2014 Search PubMed.
-
SANS 241–1:2011 Drinking water Part 1: Microbiological, physical, aesthetic and chemical determinands, South African Bureau of Standards, Pretoria, South Africa, 2011 Search PubMed.
-
C. D. Swartz, C. J. Coomans, H. P. Muller, J. A. du Plessis and W. Kamish, Decision-support model for the selection and costing of direct potable reuse systems from municipal wastewater, Research Report no. 2119/1/14Water Research Commission, Pretoria, South Africa, 2014, Also available online at http://www.wrc.org.za/Pages/DisplayItem.aspx?ItemID=10780&FromURL=%2fPages%2fKH_AdvancedSearch.aspx%3fdt%3d1%26ms%3d%26d%3dDecision-support+model+for+the+selection+and+costing+of+direct+potable+reuse+systems+from+municipal+wastewater%26start%3d1 Search PubMed.
-
DWA. National Water Resource Strategy. Water for an Equitable and Sustainable Future, 2nd edn, Department of Water Affairs, Available at: https://www.dwa.gov.za/nwrs/LinkClick.aspx?fileticket=u_qFQycClbI%3d&tabid=91&mid=496 and https://www.dwa.gov.za/nwrs/NWRS2013.aspx (Accessed 25 February 2015) 2013 Search PubMed.
-
DWA, National Strategy for Water Re-use, Available at: https://www.dwa.gov.za/nwrs/LinkClick.aspx?fileticket=WIXBU9U2xzg%3D&tabid=91&mid=496 (Accessed 25 February 2015) 2011 Search PubMed.
-
A. M. van Niekerk and B. Schneider, Implementation plan for direct and indirect water reuse for domestic purposes – sector discussion document, Research Report No. KV 320/13, Water Research Commission, Pretoria, South Africa, 2014, Also available online at http://www.wrc.org.za/Pages/DisplayItem.aspx?ItemID=10623&FromURL=%2fPages%2fKH_AdvancedSearch.aspx%3fdt%3d1%26ms%3d%26d%3dImplementation+plan+for+direct+and+indirect+water+re-use+for+domestic+purposes+%e2%80%93+sector+discussion+document%26start%3d1 Search PubMed.
-
Australian Bureau of Meteorology – Climate Resilient Water Sources
http://www.bom.gov.au/water/crews/ (Accessed 19 June 2015) Search PubMed.
- J. Radcliffe, Water recycling in Australia – during and after the drought, Environ. Sci.: Water Res. Technol. 10.1039/c5ew00048c.
-
J. Radcliffe, Water Recycling in Australia, A review by the Academy of Technological Sciences and Engineering, 2004http://www.environment.gov.au/system/files/pages/5590fe3c-1a60-4558-9d14-381b98ee80d1/files/hs44radcliffe-water-recycling-australia.pdf Search PubMed.
-
Progress against the national target of 30% of Australia's wastewater
being recyclced by 2015. Report for the Department of Sustainability, Environment, Water, Population and Communities, http://www.environment.gov.au/system/files/resources/1602789a-dae0-49fe-9e6b-5d46691b9f64/files/recycled-water-target-final-report.pdf Search PubMed.
- C. Rodriguez, P. Van Buynder, R. Lugg, P. Blair, B. Devine, A. Cook and P. Weinstein, Indirect Potable Reuse: A sustainable Water Supply Alternative, Int. J. Environ. Res. Public Health, 2009, 6(3), 1174–1209 CrossRef CAS PubMed
http://www.ncbi.nlm.nih.gov/pmc/articles/PMC2672392/
.
- W. Traves, E. Gardner, B. Dennien and D. Spiller, Towards indirect potable reuse in South East Queensland, Water Sci. Technol., 2008, 58(1), 153–161, DOI:10.2166/wst.2008.635.
-
Maintenance of water infrastructure assets. Queensland Audit Office Report to Parliament 14: 2012–13, www.qao.qld.gov.au/files/file/RTP14.pdf (Accessed 21 June 2015) Search PubMed.
-
Grand wastewater designs, Australian Antarctic Magazine, Issue 25, 2013, p. 30 Search PubMed.
- Australian Water Recycling Centre of Excellence, Strategic Research Plan www.australianwaterrecycling.com.au/publications/ (Accessed 21 June 2015).
-
National Water Quality Management Strategy, Australian Guidelines for Water Recycling – Augmentation of Drinking Water Supplies – 2008http://www.environment.gov.au/water/quality/publications/nwqms-australian-guidelines-water-recycling-managing-health-phase2 (Accessed 19 June 2015) Search PubMed.
-
Water Services Association of Australia, https://www.wsaa.asn.au/pages/default.aspx (Accessed 19 June 2015).
-
Water Research Australia, http://www.waterra.com.au/ (Accessed 19 June 2015).
-
National Centre of Excellence in Desalination Australia
http://desalination.edu.au/ (Accessed 19 June 2015).
-
Commonwealth Scientific and Industrial Research Organisation
http://www.csiro.au/ (Accessed 19 June 2015).
-
Drinking Water through Recycling, The benefits and costs of supplying direct to the distribution system - a report of a study by the Australian Academy of Technological Sciences and Engineering, 2013http://www.australianwaterrecycling.com.au/research-publications.html (Accessed 19 June 2015) Search PubMed.
-
Bureau of Meteorology Climate Resilient Water Sources
www.bom.au/water/crews (Accessed 21 June 2015) Search PubMed.
-
Recycled Water Task Force, Water Recycling 2030: Recommendations of California's Recycled Water Task Force, 2003, pp. xi. Available at: http://www.water.ca.gov/pubs/use/water_recycling_2030/recycled_water_tf_report_2003.pdf Search PubMed.
- US Census Bureau, U.S. Population Projections: 2012 to 2060www.gwu.edu/~forcpgm/Ortman.pdf (Accessed 12 June 2015) Search PubMed.
-
NRC, Water Reuse: Potential for Expanding the Nation's Water Supply through Reuse of Municipal Wastewater, National Research Council of the National Academies, The National Academies Press, Washington, D.C, USA, 2012 Search PubMed.
-
R. Raucher, J. Clements, C. Donovan, D. Chapman, R. Bishop, G. Johns, M. Hanemann, S. Rodkin and J. Garrett, The Value of Water Supply Reliability in the Residential Sector, WateReuse Research Foundation, Alexandria, VA USA, 2013 Search PubMed.
-
United States Drought Monitor
http://droughtmonitor.unl.edu/(Accessed 12 June 2015) Search PubMed.
-
WateReuse California, 2015.
-
G. Tchobanoglous, H. Leverenz, M. Nellor and J. Crook, Direct Potable Reuse: A Path Forward, WateReuse Research Foundation, WateReuse California, Alexandria, VA. http://www.watereuse.org/product/direct-potable-reuse-pathforward 2011. Available at: https://www.watereuse.org/product/direct-potable-reuse-path-forward Search PubMed.
- B. Pecson, S. Trussell, A. Pisarenko and R. Trussell, J. - Am. Water Works Assoc., 2015, 107, 45–58 CrossRef.
- Water & Sanitation Africa, Nov/Dec 2011. South Africa's award-winning seawater desalination plant 29–33; Celebrating a decade of achievement 4–5.
-
World Health Organization, Guidelines for drinking-water quality, 4th edn, 2011 Search PubMed.
-
A. M. van Niekerk and B. Schneider, Implementation plan for direct and indirect water reuse for domestic purposes – sector discussion document, Research Report No. KV 320/13, Water Research Commission, Pretoria, South Africa, 2014, Also available online at http://www.wrc.org.za/Pages/DisplayItem.aspx?ItemID=10623&FromURL=%2fPages%2fKH_AdvancedSearch.aspx%3fdt%3d1%26ms%3d%26d%3dImplementation+plan+for+direct+and+indirect+water+re-use+for+domestic+purposes+%e2%80%93+sector+discussion+document%26start%3d1 Search PubMed.
-
National Water Quality Management Strategy
http://www.environment.gov.au/water/quality/national-water-quality-management-strategy (Accessed 19 June 2015) Search PubMed.
-
U.S. EPA, Water
Reuse Guidelineshttp://nepis.epa.gov/Adobe/PDF/P100FS7K.pdf (Accessed 12 June 2015) Search PubMed.
-
SANS 241–1:2011 Drinking water Part 1: Microbiological, physical, aesthetic and chemical determinands, South African Bureau of Standards, Pretoria, South Africa, 2011 Search PubMed.
-
SANS 241–2:2011 Drinking water Part 2: Application of SANS 241–1, South African Bureau of Standards, Pretoria, South Africa, 2011 Search PubMed.
- M. P. Rice, R. Leifer and G. C. O'Connor, Commercializing Discontinuous Innovations: Bridging the Gap From Discontinuous Innovation Project to Operations, IEEE Trans. Eng. Manage., 2002, 49(4), 330–340 CrossRef.
- S. F. Slater and J. J. Mohr, Successful Development and Commercialization of Technological Innovation: Insights Based on Strategy Type, J. Prod. Innovat. Manag., 2006, 23, 26–33 CrossRef PubMed.
-
A. Arundel and C. Bordoy, Developing internationally comparable indicators for the commercialization of publicly-funded research. United Nations University UNU-MERIT Working Paper Series, 2008, #2008-075 Search PubMed.
Footnote |
† Technologies here are broadly defined as software, firmware, documented methodology or process, documentation or other material including, specifications, business rules or requirements, user manuals, user guides, operations manuals, training materials and instructions. |
|
This journal is © The Royal Society of Chemistry 2015 |