DOI:
10.1039/C4DT03462G
(Communication)
Dalton Trans., 2015,
44, 2953-2955
Divalent ytterbium complexes with crown and heterocrown ethers†‡
Received
11th November 2014
, Accepted 1st December 2014
First published on 2nd December 2014
Abstract
Several unusual complexes of ytterbium(II) stabilised by coordination to mixed O4X2-donor macrocyclic ligands (X = O, NH, S or Se) are described. Distorted 8-coordination is evident from the crystal structure of the neutral [YbI2([18]aneO4Se2)], forming the first reported example of Yb(II)-selenoether coordination.
The chemistry of the lanthanides (4f elements) is dominated by the 3+ oxidation state,1 and apart from the fluorides and oxides of Pr4+ and Tb4+, only cerium forms an extensive range of compounds in the 4+ state.1–3 For many years lower oxidation states were limited to some divalent samarium, europium and ytterbium complexes, and compounds such as LaI2 or GdS, which were formulated as “metallic” Ln(III) species with the extra electron delocalised in a conduction band.2 Early uses of Eu(II) as a one electron reductant were followed by the development of a similar role for SmI2 and [(Cp*)2Sm(thf)2].4 Recent work aimed at developing suites of lanthanide(II) complexes with varying reactivities for use in organic reductions, couplings, polymerisation and dinitrogen activation has led to new organometallic complexes of all the M(II) (except for Pm) as [K(macrocycle)][Ln(C5H4SiMe3)3] (macrocycle = 18-crown-6 or 222-crypt),§ although samarium(II) reagents remain the most widely used in organic chemistry.5
We recently reported studies of Ln(
III) complexes of oxa-thia, oxa-selena macrocycles and crown ethers, focused on La and Lu complexes, including X-ray structural characterisation of rare examples of Ln-thioether and the first examples of Ln-selenoether coordination.
6 The metal iodides were used for much of this work,
6 since for both the lanthanide and some early transition metal complexes
7 the metal iodides are more soluble than lighter halide analogues in modestly polar organic solvents, presumably due to lower lattice energies, which facilitates synthesis and solution studies. Here we report studies of similar macrocyclic ligand complexes of ytterbium(
II) iodide. The f
14 Yb(
II) permits direct comparison with the isoelectronic Lu(
III) complexes,
6 as well as with recently reported complexes of these ligands with Ca and Sr,
8 whilst its diamagnetism aids solution NMR studies.
Reaction of anhydrous YbI2 with the ligands 18-crown-6, [18]aneO4S2, [18]aneO4Se2 and [18]aneO4N2 in anhydrous MeCN gave modest yields of white to pale yellow species, [YbI2(ligand)] (Scheme 1); the formulation was confirmed by microanalysis.
 |
| Scheme 1 | |
The IR spectra showed the presence of the ligand and absence of MeCN (and H2O). The complexes are moderately soluble in anhydrous MeCN, and very readily hydrolysed and oxidised by exposure to air. Very small crystals of [YbI2([18]aneO4Se2)] were obtained after storing an MeCN solution of the complex in a freezer (−18 °C).
The complex has crystallographic two-fold symmetry and is isomorphous with [CaI2([18]aneO4Se2)],8 based upon hexadentate coordination of the macrocycle, with two mutually cis iodide ligands completing the distorted 8-coordination at Yb(II) (Fig. 1). The bond lengths and angles are also very similar to the Ca(II) species. This is as expected given their similar ionic radii (Yb2+ = 1.16 Å, Ca2+ = 1.14 Å).9 The neutral [YbI2([18]aneO4Se2)] molecule is also structurally very similar to the cation in the isoelectronic [LuI2([18]aneO4Se2)]I·2MeCN,6 albeit with slightly shorter corresponding bonds (by ∼0.1–0.15 Å) in the latter, reflecting the smaller radius resulting from the higher charge (Lu3+ ionic radius 1.00 Å). In the Yb(II) complex, the Se–M–Se angle is some 13° wider than in the Lu(III) analogue, and the I–M–I angle ∼5° wider. The larger La3+ ion (ionic radius = 1.22 Å)9 forms nine-coordinate [LaI3(macrocycle)] with these hexadentate macrocycles.6
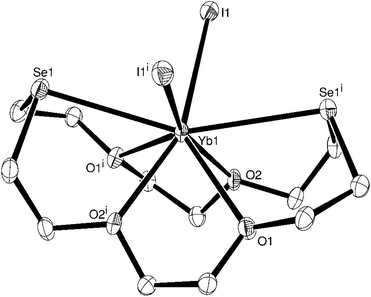 |
| Fig. 1 View of the structure of [YbI2([18]aneO4Se2)] showing the atom labelling scheme. The displacement ellipsoids are drawn at the 50% probability level and H atoms are omitted for clarity. Symmetry operation: i = −x, y, 1/2 − z. Selected bond lengths (Å) and angles (°): Yb1–I1 = 3.1699(5), Yb1–Se1 = 3.1036(7), Yb1–O1 = 2.624(3), Yb1–O2 = 2.472(3), O1–Yb1–O1i = 117.98(14), O1–Yb1–O2 = 76.42(10), O1i–Yb1–Se1 = 65.00(7), O2i–Yb1–Se1 = 70.00(7), Se1–Yb1–Se1i = 152.86(2), I1–Yb1–I1i = 100.488(19). | |
Although we were unable to obtain diffraction-quality crystals of [YbI2(18-crown-6)], [YbI2([18]aneO4S2)] or [YbI2([18]aneO4N2)], on the basis of microanalytical and spectroscopic data it seems highly probable that they also contain eight-coordinate Yb(II).
The complexes are very poorly soluble in CH2Cl2, however, they are moderately soluble in MeCN, affording yellow solutions. 1H NMR spectra of [YbI2([18]aneO4S2)] and [YbI2([18]aneO4Se2)] in anhydrous CD3CN show broad OCH2 and S(Se)CH2 resonances, with smaller coordination shifts than observed in the corresponding Lu(III) cations.6 We were unable to observe a 77Se NMR resonance from [YbI2([18]aneO4Se2)], contrasting with [LuI2([18]aneO4Se2)]I which exhibited a sharp resonance with a low frequency coordination shift (Δ) of −32 ppm. The NMR data are consistent with the macrocycles exhibiting dynamic processes in solution and weaker binding to the Yb(II), compared with the isoelectronic Lu(III) analogues. In 10−3 mol dm−3 solutions in anhydrous MeCN, the complexes have high molar conductivities, in the range characteristic of 1
:
2 electrolytes,10 which suggests that like the Ln(III) analogues,6 the iodide ligands are displaced by MeCN in dilute solution, although MeCN is not present in the solid complexes isolated from this solvent.
Cyclic voltammetry experiments were performed to probe the redox responses from these complexes. Owing to their poor solubility in non-coordinating solvents such as CH2Cl2, electrochemistry experiments were initially attempted using 0.1 mol dm−3 [NnBu4]I in anhydrous MeCN as supporting electrolyte. However, the presence of excess iodide significantly suppressed the solubility of the complexes. Therefore, subsequent experiments used 0.1 mol dm−3 [NnBu4][BF4]–MeCN as supporting electrolyte. Under these conditions the complexes each show voltammetric peaks attributed to the electro-oxidation of free iodide. The relative peak heights indicated the liberation of one iodide ion per Yb(II) complex. No other peaks associated with Yb(II)/(III) redox couples were evident under these conditions over the potential range −2 to +1.5 V.
Experimental
Details of the spectroscopic and electrochemical measurements and X-ray crystallography are given in the ESI.‡
Synthesis
[YbI2(18-crown-6)].
YbI2 (0.075 g, 0.176 mmol) was dissolved in acetonitrile (10 mL). 18-crown-6 (0.047 g, 0.176 mmol) dissolved in acetonitrile (10 mL) was then added and the reaction mixture was stirred for ca. 10 min. The solvent was removed in vacuo and the residual very pale yellow solid was washed with hexane (10 mL) and dried in vacuo. Yield 0.102 g, 84%. Required for C12H24I2O6Yb (691.2): C, 20.85.19; H, 3.50. Found C, 21.02; H, 3.39%. 1H NMR (CD3CN, 300 K): δ 3.80 (s) ppm. ΛM (MeCN 10−3 mol dm−3) = 189 Ω−1 cm2 mol−1.
[YbI2([18]aneO4S2)].
YbI2 (0.050 g, 0.117 mmol) was dissolved in acetonitrile (10 mL). [18]aneO4S2 (0.035 g, 0.117 mmol) also dissolved in acetonitrile (10 mL) was then added and the reaction mixture was stirred for ca. 10 min. After reducing the solvent volume by a quarter in vacuo, toluene (20 mL) was added. This led to precipitation of a small amount of off-white precipitate. The flask was then placed in the freezer for ca. 2 h which increased the amount of precipitate. This was collected by filtration and dried in vacuo. Yield: 0.030 g, 36%. Required for C12H24I2O4S2Yb (723.3): C, 19.93; H, 3.34. Found C, 19.82; H, 3.26%. 1H NMR (CD3CN, 300 K): δ 3.80 (br s, [16H] OCH2), 2.80 (br s, [8H], SCH2CH2O) ppm. ΛM (MeCN 10−3 mol dm−3) = 220 Ω−1 cm2 mol−1.
[YbI2([18]aneO4Se2)].
Made similarly to the [18]aneO4S2 complex above, using YbI2 (0.050 g, 0.117 mmol) and [18]aneO4Se2 (0.046 g, 0.117 mmol). Pale yellow solid. Yield: 0.030 g, 32%. Required for C12H24I2O4Se2Yb (817.1): C, 17.64; H, 2.96. Found C, 17.49; H, 2.85%. 1H NMR (CD3CN, 300 K): δ 3.83 (br t, J = 6 Hz, [8H], SeCH2CH2O), 3.72 (s, [8H], OCH2CH2O), 2.84 (t, J = 6 Hz, [8H], SeCH2CH2O) ppm. 77Se NMR (CH3CN, 295 K and 230 K): not observed. Crystals were obtained by storing an MeCN solution of the complex for several weeks in a freezer at −18 °C. ΛM (MeCN 10−3 mol dm−3) = 255 Ω−1 cm2 mol−1.
[YbI2([18]aneO4N2)].
YbI2 (0.050 g, 0.117 mmol) was dissolved in acetonitrile (10 mL). [18]aneO4N2 (0.031 g, 0.117 mmol) was also dissolved in acetonitrile (10 mL) and added to the YbI2 solution. The reaction mixture was stirred for ca. 10 min. by which time a small amount of pale yellow precipitate had formed. The solvent volume was reduced by 50% in vacuo, increasing the amount of precipitate. This was then collected by filtration and dried in vacuo. Yield: 0.044 g, 54%. Required for C12H26I2N2O4Yb (689.2): C, 20.91; H, 3.80, N, 4.06. Found: C, 20.87; H, 3.73; N, 4.13%. IR (Nujol mull): 3220 cm−1 νNH. 1H NMR (CD3CN, 300 K): δ 3.78 (v.br m, [8H] OCH2), 3.59 (v.br m, [8H] OCH2CH2N), 2.95 (v.br s, [4H] CH2N), 2.68 (v.br s, [4H], CH2N), 2.40 (v.br s, [2H], NH) ppm. ΛM (MeCN 10−3 mol dm−3) = 225 Ω−1 cm2 mol−1.
Conclusions
The synthesis, spectroscopic and structural characterisation of rare heterocrown complexes of a divalent lanthanide, specifically Yb(II), has been achieved. Coordination of soft thioether and selenoether functions to Yb(II) has been demonstrated. The properties of these complexes are intermediate between those of Ln(III) and Ca(II)/Sr(II) analogues. More generally, although the coordination of the soft donors is clearly aided by the presence of O-donors in the macrocycle, the study suggests that a wider range of coordination complexes derived from divalent lanthanides with neutral ligands, including other soft Lewis bases, should be accessible with judicious choice of solvent, reaction conditions and anion.
Acknowledgements
We thank EPSRC for support (EP/1033394/1). The SCFED Project (http://www.scfed.net) is a multidisciplinary collaboration of British universities investigating the fundamental and applied aspects of supercritical fluids.
Notes and references
-
S. Cotton, in Comprehensive Coordination Chemistry II, ed. J. A. McCleverty and T. J. Meyer, Elsevier, Oxford, 2004, vol. 4, p. 93 Search PubMed.
- D. A. Johnson, Adv. Inorg. Chem. Radiochem., 1977, 20, 1 CrossRef CAS.
- N. A. Piro, J. R. Robinson, P. J. Walsh and E. J. Schelter, Coord. Chem. Rev., 2014, 260, 21 CrossRef CAS.
-
(a) W. J. Evans, Coord. Chem. Rev., 2000, 206–207, 263 CrossRef CAS;
(b) M. N. Bochkarev, Coord. Chem. Rev., 2004, 248, 835 CrossRef CAS.
-
(a) M. Szostak and D. J. Procter, Angew. Chem., Int. Ed., 2012, 51, 9238 CrossRef CAS PubMed;
(b) M. R. MacDonald, J. E. Bates, J. W. Ziller, P. Furche and W. J. Evans, J. Am. Chem. Soc., 2013, 135, 9857 CrossRef CAS PubMed.
- M. J. D. Champion, P. Farina, W. Levason and G. Reid, Dalton Trans., 2013, 42, 13179 RSC.
-
(a) W. Levason, M. L. Matthews, B. Patel, G. Reid and M. Webster, Dalton Trans., 2004, 3305 RSC;
(b) S. D. Reid, A. L. Hector, W. Levason, G. Reid, B. J. Waller and M. Webster, Dalton Trans., 2007, 4769 RSC.
- P. Farina, W. Levason and G. Reid, Dalton Trans., 2013, 42, 89 RSC.
-
F. A. Cotton, G. Wilkinson, C. A. Murillo and M. Bochmann, Advanced Inorganic Chemistry, Wiley, New York, 6th edn, 1999 Search PubMed.
- 1
:
1 Electrolytes have ΛM (MeCN 10−3 mol dm−3) ∼120–160 and 1
:
2 electrolytes ∼220–300 Ω−1 cm2 mol−1. W. J. Geary, Coord. Chem. Rev., 1971, 6, 81 CrossRef.
Footnotes |
† Dedicated to the memory of Professor Ken Wade FRS in recognition of his many contributions to inorganic chemistry. |
‡ Electronic supplementary information (ESI) available: Further experimental and crystallographic details for the structure of [YbI2([18]aneO4Se2)]. CCDC 1024072. For ESI and crystallographic data in CIF or other electronic format see DOI: 10.1039/c4dt03462g |
§ [18]aneO4S2 = 1,4,10,13-tetraoxa-7,16-dithiacyclooctadecane, [18]aneO4Se2 = 1,4,10,13-tetraoxa-7,16-diselenacyclooctadecane, [18]aneN2O4 = 1,4,10,13-tetraoxa-7,16-diazacyclooctadecane, 18-crown-6 = 1,4,7,10,13,16-hexaoxacyclooctadecane. |
|
This journal is © The Royal Society of Chemistry 2015 |