DOI:
10.1039/C4DT03319A
(Paper)
Dalton Trans., 2015,
44, 6358-6362
Assembling anionic Sb(V)/(III) containing polyoxostibonates stabilized by triphenyltellurium cations†
Received
28th October 2014
, Accepted 26th November 2014
First published on 26th November 2014
Abstract
Reactions of diphenyltellurium oxide with organostibonic acid and polymeric triphenylantimony oxide have been investigated independently. Single crystal X-ray diffraction studies have revealed the formation of novel and rare mixed valent Sb(V)/(III) containing polyoxostibonates {(C6H5)3Te}2{Na2(H2O)2(p-Br-C6H4SbV)10(SbIII)4[(C6H5)2Te]4(O)30(OH)4} 1 and {(C6H5)3Te}4{[(C6H5)2SbV]4(SbIII)4(O)12(OH)4} 2. Solution 125Te NMR supports the solid state structures presented. Interestingly, during the assembly of these POMs several processes happen simultaneously. Rarely observed reduction from Sb(V) to Sb(III), complete dearylation of organoantimony precursors and complexation ability of a tetraorganoditelluroxane moiety stabilizing large and novel POM structures (as in 1) along with the formation of triphenyltellurium cations which not only provide charge balance but also stabilize the POM framework (as in 2) by weak interactions are reported.
Introduction
Polyoxometalates (POMs) of transition metal ions although known since the early nineteenth century1 still continue to attract attention due to the rich structural diversity they display and also due to their variety of applications.2 On the other hand POMs which exclusively containing only main group elements are very rare although main group element substituted heteropolyoxometalates are well documented.3 Recently polynuclear bismuth clusters showing structural similarities with POMs have been reported.4 Here the synthesis and structural characterization of polynuclear bismuth oxido clusters reported by Mehring et al. need a special mention.5 On the other hand POMs based on antimony are rare.6 The first report on POMs based on organoantimony was in 2007 wherein organostibonic acids were used as starting materials for building POM frameworks encapsulating transition metal ions (Mn or Zn) in their cavity.7 Organostibonic acids are ill-defined, high molecular weight polymers whose solid state structure has been a matter of considerable debate.8 Recently, controlled hydrolysis of 2,6-Mes2C6H3SbCl4 under basic conditions leading to the isolation of the first molecular arylstibonic acid which crystallized as a dimer in the solid state has been reported.9 Further to understand in detail about the molecular structures of organostibonic acids, mass spectrometry has been observed to serve as a useful tool.10 In a similar way diorganotellurium oxides R2TeO (R = alkyl, aryl) have been utilized as mild oxygen transfer reagents in organic and organometallic synthesis.11 The work of Gabbaï et al. on the investigation of the redox properties of main group/transition metal platforms and the study of the umpolung nature of Sb–Au, Te–Au, Te–Au bonds should also be mentioned.12 It has been demonstrated that the solutions of diorganotellurium oxide along with diorganotin oxide or dialkyltellurium dihydroxide can readily absorb atmospheric CO2 to form stable tellurostanoxane carbonates or telluroxane carbonates.13 Diorganotellurium oxides are basic in aqueous solutions and in the presence of protic acids they tend to associate and form a tetraorganoditelluroxane [R2TeOTeR2] moiety.14 Continuing our interest in the investigation of the depolymerization reactions of organostibonates,15 the reactions of organostibonic acid and polymeric triphenylantimony oxide with diphenyltellurium oxide independently were carried out. Synthesis and characterization of {(C6H5)3Te}2{Na2(H2O)2(p-Br-C6H4SbV)10(SbIII)4[(C6H5)2Te]4(O)30(OH)4} 1 and {(C6H5)3Te}4{[(C6H5)2SbV]4(SbIII)4(O)12(OH)4} 2 are reported.
Results and discussion
The synthetic procedure employed for 1 is as follows (Scheme 1). p-Bromophenylstibonic acid and diphenyltellurium oxide were taken in 1
:
1 mole ratio and refluxed in toluene for 12 h resulting in a clear solution. Filtration followed by evaporation of the solvent under reduced pressure resulted in the formation of a colorless solid. Single crystals suitable for X-ray diffraction were grown using the dichloromethane/hexane diffusion method. 2 was prepared by reacting polymeric triphenylantimony oxide with diphenyltellurium oxide in 1
:
2 mole ratio under toluene reflux conditions for 12 h which resulted in the formation of a fluffy white solid (Scheme 1). Flake-like single crystals were grown by diffusion of hexane into a dichloromethane–methanol mixture. 1 and 2 were characterized by standard spectroscopic and analytical techniques. Solution NMR for 1 and 2 were performed in CDCl3 and a CD2Cl2–CD3OD mixture respectively. Solution 125Te NMR of 1 shows two resonances at δ = 1162 and 787 ppm which clearly indicates the presence of two different tellurium environments in 1. Solution 125Te NMR of 2 shows a single resonance peak at δ = 776 ppm which indicates the presence of a tellurium atom in a unique environment in 2.
 |
| Scheme 1 | |
1 and 2 have been characterized by single crystal X-ray diffraction studies. Crystallographic data and selected metric parameters for 1 and 2 are provided in ESI.†1 crystallizes in the monoclinic space group P21/n with half of the molecule in the asymmetric unit. The molecular structure of 1 represents a dianionic tetradecanuclear organoantimony oxo cluster chelated by two tetraphenylditelluroxane moieties (Fig. 1). The charge is balanced by the presence of two triphenyltellurium cations which crystallize along with the core. The tetradecanuclear organoantimony oxo cluster can be described as follows. Of the fourteen Sb atoms present, ten are present in the +5 state of oxidation displaying six coordinations and four Sb atoms are present in the +3 state of oxidation displaying a pseudo trigonal bipyramidal geometry with stereochemically active lone pair on antimony occupying one of the equatorial positions.16 The ten Sb(V) atoms are present as two triads and two diads. Each triad is formed by each polyhedron of the triad sharing an edge with the adjacent polyhedron and further all the three polyhedra sharing a common vertex. The diads are formed by two polyhedra sharing a common edge. Each triad is connected to two diads by two vertex sharing polyhedra with each of the diad. The triads are further connected on two sides to the diads through a Sb(III) atom. Of the four coordinations present for the Sb(III) atom, three are the oxygen linked to Sb(V) atoms and the fourth coordination comes from the binding tetraphenylditelluroxane unit. The ability of diorganotellurium oxides/dihalides to form tetraorganoditelluroxane [R2TeOTeR2] in the presence of protic acids is known in the literature.14,17 The novelty here is the ability of tetraorganoditelluroxane in stabilizing large POM frameworks. The Sb–O distance falls in the range of 1.946(7) Å–2.186(7) Å which are comparable to earlier Sb(V)–O distances reported in the literature.6,15
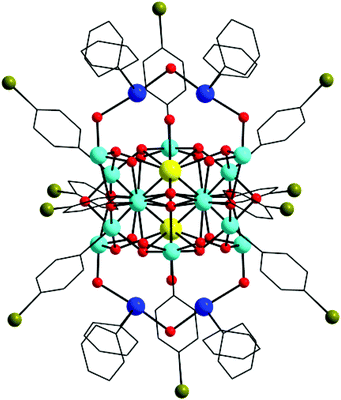 |
| Fig. 1 Solid state molecular structure of 1. Hydrogens, triphenyltellurium cations and dichloromethane solvates are omitted for clarity. | |
Interestingly mono- and di-dearylation of organoantimony compounds have been reported in the literature.18 To the best of our knowledge complete dearlytion is rare.19 Herein complete dearylation of the organoantimony precursor is reported. BVS calculations have been used for determining the oxidation state of the metal atoms present in the cluster.20 The oxidation state value in 1 for Sb present in SbO4 units corresponds to that of the +3 state of oxidation. Another interesting aspect about the structure is the partial reduction of Sb(V) to Sb(III).
The possible reason for the reduction is dealt with in the later parts of the manuscript when a similar situation is also encountered in 2. Sb(III) can readily oxidise to form Sb(V) and recently the synthesis of a mixed valent Sb(III)/Sb(V) oxido cluster in which Sb(III) is oxidised to Sb(V) by air has been reported.21 Herein reduction of Sb(V) to Sb(III) in the presence of diphenyltellurium oxide is observed. Further due to the strong affinity of arylstibonates towards Na+ ions, two Na+ ions are encapsulated in the Sb14 core. With the help of ESI-mass spectroscopy, it has been inferred that these Na+ ions originated possibly from the glassware due to leaching.10 The sodium ions are present in an eight-coordinate geometry. The Na–O bond distances fall in the range of 2.355(9) Å–2.912(8) Å. Of the 34 oxo groups present in 1, 22 are μ2-bridging and 4 are μ4-bridging while the other 8 are μ3-bridging in which 4 of them are considered to be part of hydroxyl ions for charge neutrality. The charge of the Sb14 cluster along with the two ligated tetraphenylditelluroxane motifs is dianionic. Two triphenyltellurium cations are found to crystallize outside the core stabilizing the charge on the cluster. A mixed valent Sb14 framework reported herein belongs to a novel class of POMs using main group elements as framework atoms.
2 crystallizes in the monoclinic space group P21/n with half of the molecule present in the asymmetric unit. The molecular structure of 2 corresponds to a tetraanionic octanuclear organoantimony oxo cluster with four triphenyltellurium cations stabilizing the cluster by weak interactions and also providing for charge neutrality (Fig. 2). The solid state structure of 2 can be described as a dimer of antimony tetramers connected through two μ2-oxo bridges (O5, O5#). Each tetramer consists of two Sb(III) and two Sb(V) centres arranged in an alternative manner connected through four μ2-oxo bridges to form a chair-like eight-membered ring. Further the two Sb(V) atoms are connected to one another by two μ2-oxo bridges. A polyhedral view of this dimer shows two octahedra around the antimony atoms sharing a common edge. Sb–O distances fall in the range of 1.998(4) Å–2.081(4) Å with an average of 2.036 Å which are found to be slightly higher when compared to 1. Each antimony atom in the dimer contains only two phenyl rings as a result of mono dearylation of the triphenylantimony oxide polymer, which is the starting precursor. The dimer is connected to two Sb(III) centers namely Sb2 and Sb4 through two μ2-oxo bridges (O1, O3 or O4, O6) at both sides, respectively. Interestingly the core of this tetramer resembles the hexa-μ-oxooctaphenyltetraantimony cluster reported in the literature.22 Further this tetrameric core is connected to another identical tetrameric core at the Sb4 centre through two μ2-oxo bridges namely O5 and O5# with Sb–O distances of 1.871(4) Å, and 2.361(4) Å, respectively, to form a chain like octameric antimony-oxo core with alternate Sb(III) and Sb(V) atoms. The Sb(III) atoms Sb2 and Sb4 are tri-coordinated and tetra-coordinated with O atoms, respectively. The geometry around the tri-coordinated Sb2 is considered to be trigonal pyramidal with stereochemically active lone pair occupying the axial position with Sb–O distances falling in the range of 1.886(4) Å–1.901(4) Å with an average of 1.876 Å which is marginally less when compared to 1. It has also been observed that this Sb(III) atom is involved in a secondary bonding interaction with O8 (Sb⋯O, 2.6785 Å). The geometry around tetra-coordinate Sb4 is pseudo trigonal bipyramidal with stereochemically active lone pair on antimony occupying one of the equatorial positions which is the common geometry assigned to antimony with four bonds.16 The Sb–O distances around Sb4 fall in the range of 1.871(4) Å–2.361(4) Å with an average of 2.020 Å, which is similar to 1. BVS calculations confirm the +3 oxidation state of Sb2 and Sb4 atoms.202 contains 16 μ2-bridging oxo groups. Of the 16, 4 μ2-oxo bridges which belong to two Sb(V) dimers are considered to be part of hydroxyls for charge neutrality. The overall charge of the tetraanionic octanuclear organoantimony oxo cluster is neutralized by the presence of four triphenyltellurium cations present outside the core. These triphenyltellurium cations act as counter ions in both 1 and 2.
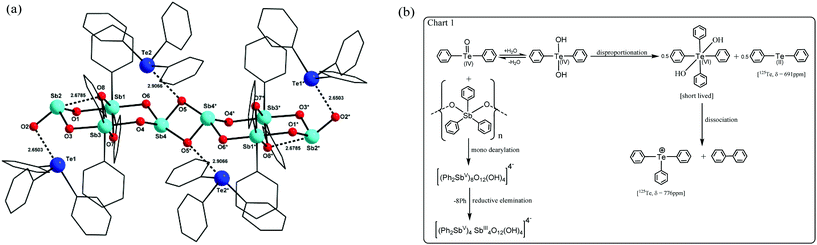 |
| Fig. 2 (a) Solid state molecular structure of 2. Hydrogen atoms are omitted for clarity. (b) Chart 1 showing the plausible mechanism for the formation of 2. | |
Diphenyltellurium oxide/diphenyl tellurium dihydroxide disproportionates23 in the presence of the organoantimony(V) oxo cluster to diphenyltelluride(II) and tetraphenyltellurium(VI) dihydroxide. Solution 125Te NMR of the filtrate (in reaction 2) shows a resonance at 691 ppm which confirms the presence of diphenyltelluride24 as a side product. A resonance corresponding to the presence of Te(VI) was not observed probably because at toluene reflux temperatures tetraphenyltellurium(VI)dihydroxide disintegrates into triphenyltellurium cations and biphenyl.25 In fact, in solution 125Te NMR a resonance corresponding to the presence of Ph3Te+ was observed at 776 ppm. This cation crystallizes as a counter ion along with the mixed valent organoantimony oxo cluster. The plausible mechanism (chart 1) for assembly of 2 can be proposed as follows: in the presence of diorganotellurium oxide, mono dearylation of the triorganoantimony precursor leading to the formation of the diorganoantimony oxo cluster [(R2SbV)8O16]4− is achieved which further undergoes partial reductive elimination on four antimony atoms leading to the formation of [(R2SbV)4SbIII4O16]4− whose solid state structure is stabilized by the presence of four triphenyltellurium cations in the crystal lattice.
Experimental section
Reagents and general procedures
(p-Bromophenyl)stibonic acid, polymeric triphenylantimony oxide and diphenyltellurium oxide were prepared according to literature reports.26 Solvents and other general reagents were purchased from commercial sources. Compounds 1 and 2 were dried under high vacuum for half an hour before being subjected to spectroscopic and elemental analysis.
Instrumentation
Infrared spectra were recorded on a Jasco-5300 FT-IR spectrometer as KBr pellets. The solution 1H, 13C, and 125Te NMR spectra were recorded on a Bruker AVANCEIII 400 instrument. Elemental analysis was performed using a Flash EA Series 1112 CHNS analyzer. Single crystal X-ray data for 1–2 were collected at 100(2) K using a Bruker Smart Apex CCD area detector system (λ(Mo Kα) = 0.71073 Å) with a graphite monochromator. The data were reduced using SAINTPLUS and the structures were solved using SHELXS-9727 and refined using SHELXL-97.28 All non-hydrogen atoms were refined anisotropically. Aryl ring hydrogens were included at calculated positions. Hydrogen atoms of two water molecules which are coordinated to sodium (in case of 1) and four hydrogens which are associated with hydroxyl ions (in cases 1 and 2) for charge neutrality were included in the final refinement.
Synthetic procedure for 1
(p-Bromophenyl)stibonic acid (0.100 g, 0.30 mmol) and diphenyltellurium oxide (0.091 g, 0.30 mmol) were refluxed in toluene for 12 h resulting in a clear solution. Dean–Stark apparatus was used to remove the water eliminated in the reaction as an azeotropic mixture. Filtration followed by evaporation of the solvent under reduced pressure resulted in a solid white precipitate which readily dissolves in dichloromethane. Single crystals suitable for X-ray diffraction were grown in dichloromethane/hexane diffusion. Yield: 0.090 g (30.9%) (based on diphenyltellurium oxide). Dec. temp: 200 °C. C144H118Br10Na2O36Sb14Te6 (5739.72): calcd C 30.13, H 2.07; found C 30.26, H 1.86. 1H NMR (400 MHz, CDCl3, ppm): δ 7.47(m, 60H), 7.32(m, 50H); 13C NMR (100 MHz, CDCl3, ppm): δ 137.99, 134.48, 131.40, 130.27, 129.52, 128.99, 127.85; 125Te NMR (126.3 MHz, CDCl3, ppm): δ 1162, 787. IR(KBr, cm−1): 3052.1(w), 2964.4(m), 1654.8(w), 1561.6(m), 1473.9(s), 1430.1(s), 1375.3(s), 1260.3(s), 1172.6(w), 1090.4(w), 1052.1(m), 1013.7(m), 800.0(s), 734.2(s), 684.9(m).
Synthetic procedure for 2
Polymeric triphenylantimony oxide (0.100 g, 0.27 mmol) and diphenyltellurium oxide (0.161 g, 0.54 mmol) were refluxed in toluene for 12 h resulting in a fluffy white solid material. The solvent was removed by filtration and the precipitate dried in air for one day. Flake-like single crystals were grown by diffusion of hexane into a dichloromethane–methanol mixture. Yield: 0.170 g (38%) (based on diphenyltellurium oxide). Dec. temp: 250 °C. C120H104O16Sb8Te4 (3286.58): calcd C 43.85, H 3.19; found C 43.76, H 3.23. 1H NMR (400 MHz, CD2Cl2–CD3OD, ppm): δ 7.87(m, 12H), 7.61(m, 62H), 7.38(m, 26H); 13C NMR (100 MHz, CD2Cl2–CD3OD, ppm): δ 134.35, 133.34, 131.92, 130.51, 128.09, 127.69, 125.36; 125Te NMR (126.3 MHz, CD2Cl2–CD3OD, ppm): δ 776. IR(KBr, cm−1): 3046.6(w), 1572.6(m), 1479.4(s), 1430.1(s), 1178.1(m), 1068.5(s), 1019.2(m), 991.8(m), 739.7(s), 684.9(m).
Conclusions
To conclude, in the presence of diorganotellurium oxide, organostibonic acid and triorganoantimony oxide self-assemble to yield mixed valent organostibonates ligated by tetraorganoditelluroxane (in 1) and stabilized by weak interactions from triaryltellurium cations (2). To the best of our knowledge this is the first instance wherein tetraorganoditelluroxane's ability to act as a ligand for stabilizing large POM frameworks has been observed. Successive reactions like mono/tri-dearylation, reductive elimination and disproportionation have taken place en route to the formation of the novel POM frameworks reported herein.
Acknowledgements
V. B. thanks the Department of Science and Technology for financial assistance under the SERB. N. K. S. thanks the Council of Scientific and Industrial Research (CSIR) for fellowship.
Notes and references
-
(a) L. Pauling, J. Am. Chem. Soc., 1929, 51, 2868 CrossRef CAS;
(b) J. F. Keggin, Proc. R. Soc. London, Ser. A, 1934, 144 Search PubMed.
-
(a) R. Neumann, Prog. Inorg. Chem., 1998, 47, 317 CrossRef CAS;
(b) S. Thomas, J. A. Bertrad and M. L. Occelli, Chem. Mater., 1999, 11, 84 CrossRef;
(c) A. Müller, S. Q. N. Shaw, H. Bögge, M. Schmidtmann, P. Kögerler, B. Hauptfleish, S. Leiding and K. Wittler, Angew. Chem., Int. Ed., 2000, 39, 1614 CrossRef;
(d)
Polyoxometalate Chemistry From Topology via Self-Assembly to Applications, ed. M. T. Pope and A. Müller, Kluwer, Dordrecht, The Netherlands, 2001 Search PubMed;
(e) T. Yamase, J. Mater. Chem., 2005, 15, 4773 RSC.
-
(a) Y. Jeannin and J. Martin-Frère, J. Am. Chem. Soc., 1981, 103, 1664 CrossRef CAS;
(b) M. Bösing, I. Loose, H. Pohlmann and B. Krebs, Chem. – Eur. J., 1997, 3, 1232 CrossRef;
(c) P. Gouzerh and A. Proust, Chem. Rev., 1998, 98, 77 CrossRef CAS PubMed;
(d) A. M. Khenkin, L. J. W. Shimon and R. Neumann, Eur. J. Inorg. Chem., 2001, 789 CrossRef CAS;
(e) U. Kortz, M. G. Savelieff, F. Y. A. Ghali, L. M. Khalil, S. A. Maalouf and D. I. Sinno, Angew. Chem., Int. Ed., 2002, 41, 4070 CrossRef CAS.
-
(a) G. Linti, W. Köstler and H. Pritzkow, Eur. J. Inorg. Chem., 2002, 2643 CrossRef CAS;
(b) K. Y. Monakhov, C. Gourlaouen, R. Pattacini and P. Braunstein, Inorg. Chem., 2012, 51, 1562 CrossRef CAS PubMed;
(c) K. Y. Monakhov, G. Linti, L. P. Wolters and F. M. Bickelhaupt, Inorg. Chem., 2011, 50, 5755 CrossRef CAS PubMed.
-
(a) P. C. Andrews, G. B. Deacon, C. M. Forsyth, P. C. Junk, I. Kumar and M. Maguire, Angew. Chem., Int. Ed., 2006, 45, 5638 CrossRef CAS PubMed;
(b) E. V. Dikarev, H. Zhang and B. Li, Angew. Chem., Int. Ed., 2006, 45, 5448 CrossRef CAS PubMed;
(c) M. Mehring, D. Mansfeld, S. Paalasmaa and M. Schürmann, Chem. – Eur. J., 2006, 12, 1767 CrossRef CAS PubMed;
(d) L. Miersch, M. Schlesinger, R. W. Troff, C. A. Schalley, T. Rüffer, H. Lang, D. Zahn and M. Mehring, Chem. – Eur. J., 2011, 17, 6985 CrossRef CAS PubMed;
(e) L. Miersch, T. Rüffer, D. Schaarschmidt, H. Lang, R. W. Troff, C. A. Schalley and M. Mehring, Eur. J. Inorg. Chem., 2013, 1427 CrossRef CAS;
(f) M. Schlesinger, A. Pathak, S. Richter, D. Sattler, A. Seifert, T. Rüffer, P. C. Andrews, C. A. Schalley, H. Lang and M. Mehring, Eur. J. Inorg. Chem., 2014, 4218 CrossRef CAS.
-
(a) B. K. Nicholson, C. J. Clark, C. E. Wright, S. G. Telfer and T. Groutso, Organometallics, 2011, 30, 6612 CrossRef CAS;
(b) B. K. Nicholson, C. J. Clark, S. G. Telfer and T. Groutso, Dalton Trans., 2012, 41, 9964 RSC;
(c) B. K. Nicholson, C. J. Clark, C. E. Wright, G. B. Jameson and S. G. Telfer, Inorg. Chim. Acta, 2013, 406, 53 CrossRef CAS PubMed.
- V. Baskar, M. Shanmugam, M. Helliwell, S. J. Teat and R. E. P. Winpenny, J. Am. Chem. Soc., 2007, 129, 3042 CrossRef CAS PubMed.
-
(a) H. Schmidt, Liebigs Ann. Chem., 1920, 421, 174 CrossRef CAS;
(b) G. O. Doak and H. G. Steinman, J. Am. Chem. Soc., 1946, 68, 1987 CrossRef CAS;
(c) G. O. Doak, J. Am. Chem. Soc., 1946, 68, 1991 CrossRef CAS;
(d) L. H. Bowen and G. G. Long, Inorg. Chem., 1978, 17, 551 CrossRef CAS.
- J. Beckmann, P. Finke, M. Hesse and B. Wettig, Angew. Chem., Int. Ed., 2008, 47, 9982 CrossRef CAS PubMed.
-
(a) C. J. Clark, B. K. Nicholson and C. E. Wright, Chem. Commun., 2009, 923 RSC;
(b) B. K. Nicholson, C. J. Clark, C. E. Wright and T. Groutso, Organometallics, 2010, 29, 6518 CrossRef CAS.
-
(a) L. Engman, Acc. Chem. Res., 1985, 18, 274 CrossRef CAS;
(b) A. Panda, Coord. Chem. Rev., 2009, 253, 1947 CrossRef CAS PubMed;
(c) C. W. Nogueira, G. Zeni and J. B. T. Rocha, Chem. Rev., 2004, 104, 6255 CrossRef CAS PubMed.
-
(a) C. R. Wade and F. P. Gabbaï, Angew. Chem., Int. Ed., 2011, 50, 7369 CrossRef CAS PubMed;
(b) C. R. Wade, T.-P. Lin, R. C. Nelson, E. A. Mader, J. T. Miller and F. P. Gabbaï, J. Am. Chem. Soc., 2011, 133, 8948 CrossRef CAS PubMed;
(c) T.-P. Lin and F. P. Gabbaï, J. Am. Chem. Soc., 2012, 134, 12230 CrossRef CAS PubMed;
(d) I.-S. Ke and F. P. Gabbaï, Inorg. Chem., 2013, 52, 7145 CrossRef CAS PubMed;
(e) H. Yang, T.-P. Lin and F. P. Gabbaï, Organometallics, 2014, 33, 4368 CrossRef CAS.
-
(a) J. Beckmann, D. Dakternieks, A. Duthie, N. A. Lewcenko and C. Mitchel, Angew. Chem., Int. Ed., 2004, 43, 6683 CrossRef CAS PubMed;
(b) J. Beckmann, J. Bolsinger and A. Duthie, Z. Anorg. Allg. Chem., 2010, 636, 765 CrossRef CAS.
- J. Beckmann, D. Dakternieks, A. Duthie, N. A. Lewcenko, C. Mitchel and M. Schürmann, Z. Anorg. Allg. Chem., 2005, 631, 1856 CrossRef CAS.
-
(a) M. S. R. Prabhu, A. K. Jami and V. Baskar, Organometallics, 2009, 28, 3953 CrossRef CAS;
(b) A. K. Jami, M. S. R. Prabhu and V. Baskar, Organometallics, 2010, 29, 1137 CrossRef CAS;
(c) A. K. Jami and V. Baskar, Dalton Trans., 2012, 41, 12524 RSC.
-
(a) D. W. Hartley, G. Smith, D. S. Sagatys and C. H. L. Kennard, J. Chem. Soc., Dalton Trans., 1991, 2735 RSC;
(b) G. Smith, D. S. Sagatys, R. C. Bott and D. E. Lynch, Polyhedron, 1992, 11, 631 CrossRef CAS.
-
(a) V. Chandrasekhar and R. Thirumoorthi, Inorg. Chem., 2009, 48, 10330 CrossRef CAS PubMed;
(b) N. K. Srungavruksham and V. Baskar, Eur. J. Inorg. Chem., 2012, 136 CrossRef CAS;
(c) V. Chandrasekhar and A. Kumar, J. Organomet. Chem., 2009, 694, 2628 CrossRef CAS PubMed.
-
(a) C. Jones, Coord. Chem. Rev., 2001, 215, 151 CrossRef CAS;
(b) V. Chandrasekhar and R. Thirumoorthi, Organometallics, 2009, 28, 2637 CrossRef CAS;
(c) N. K. Srungavruksham and V. Baskar, Eur. J. Inorg. Chem., 2013, 4345 CrossRef CAS.
- P. V. V. N. Kishore and V. Baskar, Inorg. Chem., 2014, 53, 6737 CrossRef CAS PubMed.
- S. J. Mills, A. G. Christy, E. C.-C. Chen and M. Raudsepp, Z. Kristallogr., 2009, 224, 423 CrossRef CAS.
- J. Beckmann, T. Heek and M. Takahashi, Organometallics, 2007, 26, 3633 CrossRef CAS.
- J. Bordner, G. O. Doak and T. S. Everett, J. Am. Chem. Soc., 1986, 108, 4206 CrossRef CAS.
-
(a) N. Petragnani and L. Torres, J. Organomet. Chem., 1974, 76, 241 CrossRef CAS;
(b) S. Ogawa, Y. Masutomi and N. Furukawa, Heteroat. Chem., 1992, 3, 423 CrossRef CAS;
(c) E. S. Lang, R. M. F. Junior, E. T. Silveira, U. Abram and E. M. Vázquez-López, Z. Anorg. Allg. Chem., 1999, 625, 1401 CrossRef CAS;
(d) G. N. Ledesma, E. S. Lang, E. M. Vázquez-López and U. Abram, Inorg. Chem. Commun., 2004, 7, 478 CrossRef CAS PubMed.
- J. H. E. Bailey, J. E. Drake, L. N. Khasrou and J. Yang, Inorg. Chem., 1995, 34, 124 CrossRef CAS.
-
(a) S. C. Cohen, M. L. N. Reddy and A. G. Massey, J. Organomet. Chem., 1968, 11, 563 CrossRef CAS;
(b) D. H. R. Barton, S. A. Glover and S. V. Ley, J. Chem. Soc., Chem. Commun., 1977, 266 RSC.
-
(a) H. Schmidt, Liebigs Ann. Chem., 1920, 421, 174 CrossRef CAS;
(b) G. O. Doak and H. G. Steinmann, J. Am. Chem. Soc., 1946, 68, 1987 CrossRef CAS;
(c) R. Rüther, F. Huber and H. Preut, Z. Anorg. Allg. Chem., 1986, 539, 110 CrossRef;
(d) N. W. Alcock and W. D. Harrison, J. Chem. Soc., Dalton Trans., 1982, 709 RSC.
-
G. M. Sheldrick, SHELXS-97, Program for Crystal Structure Solution, University of Göttingen, Göttingen, Germany, 1997 Search PubMed.
-
G. M. Sheldrick, SHELXL-97, Program for Crystal Structure Refinement, University of Göttingen, Göttingen, Germany, 1997 Search PubMed.
Footnote |
† Electronic supplementary information (ESI) available: Crystallographic data, selected bond lengths and bond angles, solution 125Te NMR spectra and ORTEP diagrams. CCDC 1029478 and 1029479 for 1 and 2. For ESI and crystallographic data in CIF or other electronic format see DOI: 10.1039/c4dt03319a |
|
This journal is © The Royal Society of Chemistry 2015 |