DOI:
10.1039/C5CE00139K
(Communication)
CrystEngComm, 2015,
17, 8796-8800
Single-crystal-to-single-crystal transformation of an anion exchangeable dynamic metal–organic framework†
Received
21st January 2015
, Accepted 5th March 2015
First published on 9th March 2015
Abstract
A three dimensional (3D) cationic Metal–Organic Framework (MOF) has been fabricated from a neutral N-donor ligand and Cd(ClO4)2. The cationic MOF shows guest triggered inherent dynamic behaviour at room temperature. A single-crystal-to-single-crystal (SCSC) transformation experiment enabled the guest dependent structural dynamism to be well understood. The framework also displays facile anion exchange behaviour and anion dependent structural dynamism.
Guest molecules in a coordination space have a profound role in directing the structures of metal–organic frameworks (MOFs)/coordination polymers (CPs).1 Especially in the domain of dynamic MOFs, the guest molecules residing in the porous aperture can regulate structures to impart flexibility to the framework.2 Soft porous MOFs are well known to exhibit such guest dependent structural dynamism.2a,3 Moreover, the framework flexibility may depend upon the nature of guest molecules. MOFs entrapping high boiling solvents often show extrinsic dynamic nature, as an external stimulus like high temperature is required for conveying these molecules out of the framework.4 On the other hand, presence of low boiling guests in a framework may generate inherent framework flexibility which forbids the necessity of an external stimuli, except air-drying conditions.5 Manifestation of structural dynamism/flexibility by cationic MOFs is very easy compared to other MOFs.5d,6 By employing appropriate low-boiling solvent systems for synthesis, the combination of a neutral N-donor ligand with metal ions yields cationic MOFs which usually are occluded with low boiling solvents and extra framework anions.7 When keeping such MOFs away from mother liquor, the confined guests may easily leave the framework and create possible structural changes in the system. Such structural modulations, upon guest removal at room temperature, renders inherent dynamism to the cationic MOFs.5c,d Recently, we have demonstrated the guest dependent dynamic behaviour of few cationic MOFs.5d,8 Such structural modifications can be well understood from their single-crystal-to-single-crystal (SCSC) transformation studies.2a,9 In addition to these guest induced effects, extra framework anions of such cationic MOFs can also be exchanged with foreign anions of varying size, shape and coordinating tendencies.7c,d,10 Substitution of such anions with other anions may also lead to structural and physical changes of the host systems.5d,7e,11 Herein, we report a three dimensional (3D) cationic MOF built from a neutral N-donor ligand (L) and Cd(II), ClO4− anions and free guests. The framework displays inherent structural dynamism through the loss of guest solvent molecules upon air-drying. The guest dependent structural changes are well understood from the SCSC transformation studies (Scheme 1). Extra framework anions in the compound can be easily exchanged with other anions of different sizes and coordinating tendencies. The compound also shows anion dependent structural dynamism.
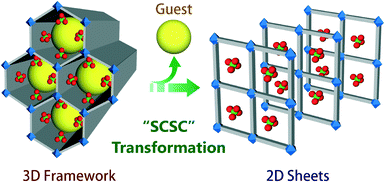 |
| Scheme 1 Schematic representation of a guest driven dynamic structural transformation from a 3D framework to 2D sheets. | |
Reaction of the ligand12 (1, 4-bis (4-pyridyl)-2, 3-diaza-1, 3-butadiene) with Cd(ClO4)2 in a solvent combination of CH2Cl2/tetrahydrofuran/methanol gave yellow colored rod shaped crystals of compound 1 [{Cd(L)3·(ClO4)2}·xG]n (where G are disordered low boiling solvent molecules). Compound 1 formed a 3D kagome-like structure with 1D porous channels as revealed from a single-crystal structural analysis. A very interesting aspect of compound 1 is that crystals of 1 undergo drastic structural transformation upon air drying without losing their single-crystalline nature. Single-crystal analysis of the new phase showed a remarkable one step dimensionality reduction to form a two-dimensional (2D) sheet-like structure 2[{Cd(L)2(OH2)2·(ClO4)2}·(THF)]n. A drastic lowering in unit cell volume also supports the formation of non-porous structure (2) from the porous (1) parent framework. It is very interesting to note that low boiling guests easily come out from the framework (1) without any external stimuli such as temperature or pressure and lead to the guest driven inherent dynamic nature of the cationic framework (1) (Fig. S13, ESI†).
A single-crystal X-ray diffraction study (SC-XRD) revealed that compound 1 crystallize in a trigonal crystal system with space group R
c. An asymmetric unit of compound 1 contains half Cd(II), one and half ligands (L), one ClO4− anion and disordered solvent molecules (Fig. S1, ESI†). The SQUEEZE routine of PLATON has been used to remove highly disordered guest molecules in compound 1. Each Cd(II) shows connections with six N-pyridyl moieties of six different ligands forming a six coordinated distorted octahedral geometry. Each ligand connects two metal centres via its terminal N-pyridyl functionality to form an extended 3D cationic structure. Single net packing of the compound results in a kagome-like structure with a 1D pore channel along c axis (Fig. 1A). Out of six ligands in a complete set of coordination of a metal centre, two show trans geometry and four of them exhibit distorted cis geometry. Thus, the overall packing of 1 shows the presence of free ClO4− anions in the interstitial voids of the cationic framework (Fig. 1B). SCSC structural transformation revealed abrupt changes in crystal system (monoclinic) in compound 2 from 1. Complete single-crystal structural analysis of 2 shows formation of 2D cationic sheets with free ClO4− anions in the framework lattice (Fig. S4, S11, ESI†). It is very worth noting that two coordinated ligands in 1 are replaced by two water molecules in 2 leading to reduction of dimensionality by one step (3D → 2D) and resulting in the formation of 2D sheet-like structures (Fig. 2). Close examination of the structure of 2 shows that two ligands orient in cis-form and two are in trans-form around each Cd(II) centre. Moreover, the oxygen atom of ClO4− anion forms H-bonds with coordinated water molecules. In addition, guest THF molecules in the structure are found to form various non-covalent interactions with C–H, ClO4− anions, and coordinated water molecules. Powder X-ray diffraction (PXRD) patterns of 2 show that it is stable at room temperature as evident from variable time PXRD (Fig. S14, ESI†). It is very important to note that 1 upon air drying changed to 2 by partial loss of guest molecules. Due to presence of such above mentioned non-covalent interactions between THF and the framework, compound 2 is stable and does not lose any further guests on standing at room temperature (Fig. S12, ESI†). Thermogravimetric analysis (TGA) of 2 shows ~8% wt loss around 100 °C and does not show any further wt loss up to 290 °C (Fig. S18, ESI†). Framework dynamic behaviour was also observed during the replacement of host anions by some incursive anions of different sizes, shapes and coordinating tendencies. From the above structural description it is evident that compound 2 contain free ClO4− anions in its lattice. To inspect the anion-driven framework's dynamic behaviour anion exchange experiments were performed. Strongly coordinating anions (viz. N3− and SCN−) of different sizes and shapes were chosen for the same. In a typical experiment, crystals of compound 2 were separately dipped in an aqueous solution of NaN3 and KSCN for ~7 days. FTIR was used as a spectroscopic tool to monitor the anion-exchange studies. It showed that complete replacement of anions by incursive anions took place in ~7 days. Strong bands related to ClO4− (~1100 cm−1) in compound 2 almost completely vanished and in their place new bands appeared at ~2050 cm−1 for 2 ⊃ N3− and at ~2080 cm−1 for 2 ⊃ SCN− respectively (Fig. 3). The exchanged compounds (2 ⊃ N3− and 2 ⊃ SCN−) showed differences in PXRD patterns compared to compound 2 (Fig. S15, ESI†). Such differential PXRD patterns emerge owing to the different coordinating tendencies size and shapes of foreign anions, thus suggesting the dynamic nature of the framework. During the course of anion exchange we made several attempts to get X-ray quality single crystals of exchanged compounds, but unfortunately we were unable to obtain them. During the course of anion exchange, we observed that the supernatant solutions of individual anion precursors remained colorless and transparent indicating MOF particles did not dissolve and recrystallize from the solvent. Owing to the differential PXRD patterns of anion exchanged products, the compound shows anion-responsive structural dynamism. Reversibility of the anion exchange experiment was also performed by taking a NaClO4 solution (1 mM/10 mL H2O). No strong bands at ~1100 cm−1 for ClO4− appeared in re exchanged products as revealed in FTIR spectra, thus indicating reversibility could not be achieved in these cases.
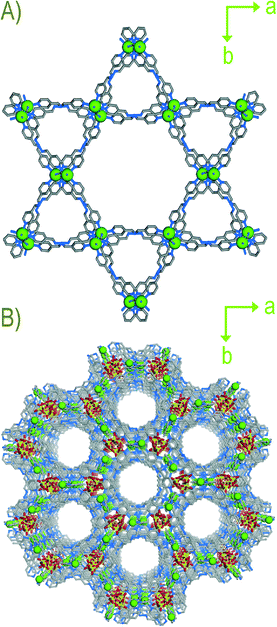 |
| Fig. 1 A) Single net packing of compound 1 along c axis. B) Perspective view of overall packing of compound (1) along c axis (free solvent molecules are hidden). | |
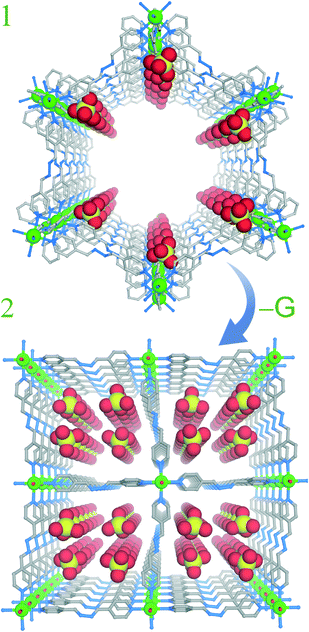 |
| Fig. 2 Guest driven structural transformation upon air drying from 1 (3D) to 2 (2D) (free solvent molecules are hidden). | |
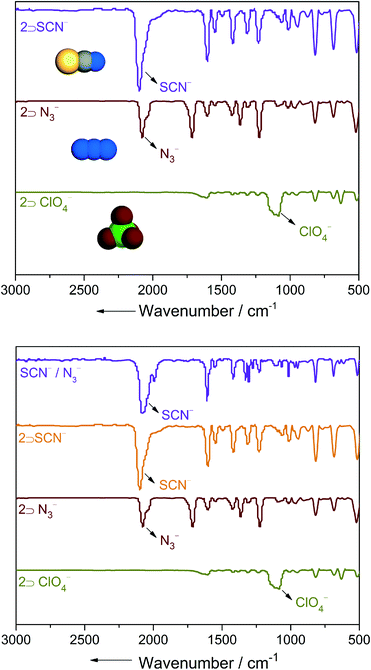 |
| Fig. 3 FTIR spectra of compound 2 and its various anion exchanged products with highlighted bands of corresponding anions (top). FTIR spectra of different binary mixtures of anions of compound 2 (bottom). | |
Encapsulation of a particular anion by a cationic moiety in presence of other competing anions is very important. Hence, to perform the guest anion affinity by cationic framework 2, we investigated an anion exchange experiment by using a binary mixture of anions: N3−/SCN−. In a representative experiment, crystals of compound 2 were immersed in an aqueous solution of equimolar mixtures of NaN3 and KSCN. An investigation of FTIR spectra showed a strong band related to SCN− at ~2080 cm−1 suggesting preferential uptake of SCN− over N3− (Fig. 3).
Conclusions
To conclude, we have synthesized a 3D cationic MOF using a N-donor ligand. The cationic MOF showed inherent dynamic behaviour owing to the loss of low boiling solvent at room temperature. Guest driven dynamic behaviour of the cationic framework was well validated by a SCSC transformation experiment. Apart from the guest driven dynamic behaviour, the cationic MOF also exhibited anion-responsive structural dynamism. The compound showed easy anion-exchange behaviour with strongly coordinating anions of different sizes and shapes in an aqueous medium. Moreover, different guest anion-affinity was also achieved with the cationic framework.
Acknowledgements
B.M is thankful to CSIR for research fellowship. We are thankful to IISER Pune, DST (project no. GAP/DST/CHE-12-0083) for the financial support. We are also thankful to DST-FIST (SR/FST/CSII-023/2012) for micro focus SC-XRD facility.
Notes and references
-
(a) H.-C. Zhou, J. R. Long and O. M. Yaghi, Chem. Rev., 2012, 112, 673–674 CrossRef CAS PubMed;
(b) A. C. McKinlay, R. E. Morris, P. Horcajada, G. Ferey, R. Gref, P. Couvreur and C. Serre, Angew. Chem., Int. Ed., 2010, 49, 6260–6266 CrossRef CAS PubMed;
(c) J. Y. Lee, O. K. Farha, J. Roberts, K. A. Scheidt, S. T. Nguyen and J. T. Hupp, Chem. Soc. Rev., 2009, 38, 1450–1459 RSC;
(d) S. Kitagawa, R. Kitaura and S. I. Noro, Angew. Chem., Int. Ed., 2004, 43, 2334–2375 CrossRef CAS PubMed;
(e) I. H. Park, A. Chanthapally, Z. Zhang, S. S. Lee, M. J. Zaworotko and J. J. Vittal, Angew. Chem., Int. Ed., 2014, 53, 414–419 CrossRef CAS PubMed;
(f) A. Shigematsu, T. Yamada and H. Kitagawa, J. Am. Chem. Soc., 2012, 134, 13145–13147 CrossRef CAS PubMed;
(g) M. C. Das and P. K. Bharadwaj, J. Am. Chem. Soc., 2009, 131, 10942–10949 CrossRef CAS PubMed;
(h) S. Sen, S. Neogi, K. Rissanen and P. K. Bharadwaj, Chem. Commun., 2015, 51, 3173–3176 RSC;
(i) J.-P. Zhang, P.-Q. Liao, H.-L. Zhou, R.-B. Lin and X.-M. Chen, Chem. Soc. Rev., 2014, 43, 5789–5814 RSC;
(j) R. Matsuda, R. Kitaura, S. Kitagawa, Y. Kubota, T. C. Kobayashi, S. Horike and M. Takata, J. Am. Chem. Soc., 2004, 126, 14063–14070 CrossRef CAS PubMed;
(k) S. K. Ghosh and S. Kitagawa, CrystEngComm, 2008, 10, 1739–1742 RSC;
(l) B. Chen, Y. Yang, F. Zapata, G. Lin, G. Qian and E. B. Lobkovsky, Adv. Mater., 2007, 19, 1693–1696 CrossRef CAS;
(m) M. C. Das, S. K. Ghosh, E. C. Sañudo and P. K. Bharadwaj, Dalton Trans., 2009, 1644–1658 RSC;
(n) W. Zhang, Y. Hu, J. Ge, H.-L. Jiang and S.-H. Yu, J. Am. Chem. Soc., 2014, 136, 16978–16981 CrossRef CAS PubMed;
(o) J.-W. Zhang, H.-T. Zhang, Z.-Y. Du, X. Wang, S.-H. Yu and H.-L. Jiang, Chem. Commun., 2014, 50, 1092–1094 RSC;
(p) K. F. Konidaris, C. N. Morrison, J. G. Servetas, M. Haukka, Y. Lan, A. K. Powell, J. C. Plakatouras and G. E. Kostakis, CrystEngComm, 2012, 14, 1842–1849 RSC;
(q) K. S. Asha, K. Bhattacharyya and S. Mandal, J. Mater. Chem. C, 2014, 2, 10073–10081 RSC.
-
(a) S. Horike, S. Shimomura and S. Kitagawa, Nat. Chem., 2009, 1, 695–704 CrossRef CAS PubMed;
(b) G. Ferey and C. Serre, Chem. Soc. Rev., 2009, 38, 1380–1399 RSC;
(c) N. Yanai, K. Kitayama, Y. Hijikata, H. Sato, R. Matsuda, Y. Kubota, M. Takata, M. Mizuno, T. Uemura and S. Kitagawa, Nat. Mater., 2011, 10, 787–793 CrossRef CAS PubMed;
(d) P. K. Thallapally, P. Mcgrail, S. J. Dalgrano, H. T. Schaef, J. Tian and J. L. Atwood, Nat. Mater., 2008, 7, 146–150 CrossRef CAS PubMed;
(e) P. K. Thallapally, J. Tian, M. Radha Kishan, C. A. Fernandez, S. J. Dalgarno, P. B. McGrail, J. E. Warren and J. L. Atwood, J. Am. Chem. Soc., 2008, 130, 16842–16843 CrossRef CAS PubMed.
-
(a) C. Serre, C. M. Draznieks, S. Surblé, N. Audebrand, Y. Filinchuk and G. Férey, Science, 2007, 315, 1828–1831 CrossRef CAS PubMed;
(b) S. K. Ghosh, S. Bureekaew and S. Kitagawa, Angew. Chem., Int. Ed., 2008, 47, 3403–3406 CrossRef CAS PubMed;
(c) S. K. Ghosh, J.-P. Zhang and S. Kitagawa, Angew. Chem., Int. Ed., 2007, 46, 7965–7968 CrossRef CAS PubMed;
(d) B. Joarder, A. K. Chaudhari, S. S. Nagarkar, B. Manna and S. K. Ghosh, Chem. – Eur. J., 2013, 19, 11178–11183 CrossRef CAS PubMed;
(e) J.-H. Wang, M. Li and D. Li, Chem. Sci., 2013, 4, 1793–1801 RSC.
-
(a) Y. Takashima, V. M. Martinez, S. Furukawa, M. Kondo, S. Shimomura, H. Uehara, M. Nakahama, K. Sugimoto and S. Kitagawa, Nat. Commun., 2011, 2, 168 CrossRef PubMed;
(b) D. N. Dybtsev, H. Chun and K. Kim, Angew. Chem., Int. Ed., 2004, 43, 5033–5036 CrossRef CAS PubMed.
-
(a) A. Kondo, H. Noguchi, L. Carlucci, D. M. Proserpio, G. Ciani, H. Kajiro, T. Ohba, H. Kanoh and K. Kaneko, J. Am. Chem. Soc., 2007, 129, 12362–12363 CrossRef CAS PubMed;
(b) A. Kondo, H. Kajiro, H. Noguchi, L. Carlucci, D. M. Proserpio, G. Ciani, K. Kato, M. Takata, H. Seki, M. Sakamoto, Y. Hattori, F. Okino, K. Maeda, T. Ohba, K. Kaneko and H. Kanoh, J. Am. Chem. Soc., 2011, 133, 10512–10522 CrossRef CAS PubMed;
(c) K. Biradha and M. Fujita, Angew. Chem., Int. Ed., 2002, 41, 3392–3395 CrossRef CAS;
(d) B. Manna, A. K. Chaudhari, B. Joarder, A. Karmakar and S. K. Ghosh, Angew. Chem., Int. Ed., 2013, 52, 998–1002 CrossRef CAS PubMed;
(e) R. Kotani, A. Kondo and K. Maeda, Chem. Commun., 2012, 48, 11316–11318 RSC.
- T. K. Maji, R. Matsuda and S. Kitagawa, Nat. Mater., 2007, 6, 142–148 CrossRef CAS PubMed.
-
(a) K. S. Min and M. P. Suh, J. Am. Chem. Soc., 2000, 122, 6834–6840 CrossRef CAS;
(b) Y.-Q. Chen, G.-R. Li, Z. Chang, Y.-K. Qu, Y.-H. Zhang and X.-H. Bu, Chem. Sci., 2013, 4, 3678–3682 RSC;
(c) X. Li, H. Xu, F. Kong and R. Wang, Angew. Chem., Int. Ed., 2013, 52, 13769–13773 CrossRef CAS PubMed;
(d) J.-P. Ma, Y. Yu and Y.-B. Dong, Chem. Commun., 2012, 48, 2946–2948 RSC;
(e) B. Manna, B. Joarder, A. V. Desai, A. Karmakar and S. K. Ghosh, Chem. – Eur. J., 2014, 20, 12399–12404 CrossRef CAS PubMed;
(f) S. Muthu, J. H. K. Yip and J. J. Vittal, J. Chem. Soc., Dalton Trans, 2001, 3577–3584 RSC.
-
(a) A. Karmakar, B. Manna, A. V. Desai, B. Joarder and S. K. Ghosh, Inorg. Chem., 2014, 53, 12225–12227 CrossRef CAS PubMed;
(b) B. Manna, S. Singh and S. K. Ghosh, J. Chem. Sci., 2014, 126, 1417–1422 CrossRef CAS.
-
(a) T. K. Maji, K. Uemura, H.-C. Chang, R. Matsuda and S. Kitagawa, Angew. Chem., Int. Ed., 2004, 43, 3269–3272 CrossRef CAS PubMed;
(b) M. P. Suh, J. W. Ko and H. J. Choi, J. Am. Chem. Soc., 2002, 124, 10976–10977 CrossRef CAS PubMed;
(c) H.-X. Li, Z.-G. Ren, D. Liu, Y. Chen, J.-P. Lang, Z.-P. Cheng, X.-L. Zhu and B. F. Abrahams, Chem. Commun., 2010, 46, 8430–8432 RSC;
(d) W. M. Bloch and C. J. Sumby, Chem. Commun., 2012, 48, 2534–2536 RSC;
(e) W. M. Bloch, A. Burgun, C. J. Coghlan, R. Lee, M. L. Coote, C. J. Doonan and C. J. Sumby, Nat. Chem., 2014, 6, 906–912 CrossRef CAS PubMed;
(f) Y.-P. Cai, X.-X. Zhou, Z.-Y. Zhou, S.-Z. Zhu, P. K. Thallapally and J. Liu, Inorg. Chem., 2009, 48, 6341–6343 CrossRef CAS PubMed.
- A. Aijaz, P. Lama and P. K. Bharadwaj, Inorg. Chem., 2010, 49, 5883–5889 CrossRef CAS PubMed.
-
(a) B. Manna, S. Singh, A. Karmakar, A. V. Desai and S. K. Ghosh, Inorg. Chem., 2015, 54, 110–116 CrossRef CAS PubMed;
(b) T. K. Maji, R. Matsuda and S. Kitagawa, Nat. Mater., 2007, 6, 142–148 CrossRef CAS PubMed;
(c) B. Chen, L. Wang, F. Zapata, G. Qian and E. B. Lobkovsky, J. Am. Chem. Soc., 2008, 130, 6718–6719 CrossRef CAS PubMed.
- D. M. Ciurtin, Y.-B. Dong, M. D. Smith, T. Barclay and H.-C. Z. Loye, Inorg. Chem., 2001, 40, 2825–2834 CrossRef CAS PubMed.
Footnote |
† Electronic supplementary information (ESI) available: Characterization, Figures, FT-IRspectra, TGA, SC-XRDdetails, PXRD, Anion exchnage experiments. CCDC 1044688 is for compound 1; compound 2 CCDC 1044689. See DOI: 10.1039/c5ce00139k |
|
This journal is © The Royal Society of Chemistry 2015 |