DOI:
10.1039/C5AY90036K
(AMC Technical Brief)
Anal. Methods, 2015,
7, 4844-4847
Raman spectroscopy in cultural heritage: Background paper
Received
7th May 2015
First published on 4th June 2015
Abstract
In the last 30 years Raman spectroscopy has become an essential experimental tool for the analysis of a wide variety of artists' materials, often in a non-destructive, non-invasive manner. The Raman effect provides a quick and relatively straightforward molecular identification of a material under examination. A Raman spectrum can be considered as a fingerprint that could be used for compound identification when a database of standard spectra is available for comparison purposes.
Its use has become widespread because Raman spectroscopy is a non-contact technique and, provided the correct experimental conditions are used by an expert operator, the materials under examination are not damaged in any way. Because of this, the technique has now become mainstream in heritage science. There is no single type of Raman instrument that can be used for all purposes: users now have a choice of instruments and operating conditions, depending on the analytical requirements and the type of art objects to be analysed.
What is Raman good for?
Types of materials
When Raman experienced a renaissance in the 1980s, its main application in cultural heritage was for the analysis of traditional artists' materials, mostly pigments of mineral origin. This is still one of its main applications, although the technique is also used for the identification of a few natural organic dyes (for example indigo and gamboge), many modern synthetic dyes, most gems and semi-precious stones, minerals in general, and corrosion products. Other cultural heritage materials that have been examined more recently include plastics, parchment, paper, textile fibres, resins, bone and bone-like materials and varnishes. In the investigation of artists' materials the success and speed of the analysis often depends on what medium is mixed with the pigments (see “Pitfalls and drawbacks – fluorescence” below).
Types of objects
With conventional bench-top instruments coupled with a microscope, only relatively small, flat items that fit under the microscope can be analysed. For this reason, it is customary to use the technique with small samples or loose fragments or materials either individually or after mounting as a cross section. Paper- or parchment-based objects, such as small whole manuscripts, single illuminated pages and portrait miniatures are also easy to examine. Other items that can be analysed with relative ease include painted textiles, textile fibres (both natural and synthetic), gems still encased in jewellery and small, flat sculptures or other painted objects.
Types of instruments
Bench-top microscope
This is the most common Raman instrument found in a laboratory and is the instrument with the highest performance in terms of speed, signal intensity, spatial and spectral resolution, stability and freedom from disruptive vibrations (Fig. 1). A wide variety of lasers can be associated with such an instrument (see below). The use of a microscope also ensures that a very small area is analysed each time, less than a micrometre across and a few micrometres in depth, depending on the specifications of the microscope objectives used. This helps in limiting the interference of surrounding materials.
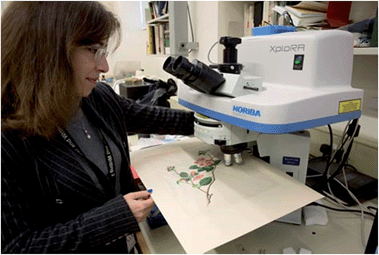 |
| Fig. 1 A 19th century Chinese watercolour analysed with a bench-top Raman microscope. | |
Probe
This is an easily transportable piece of equipment that, contrary to the better-performing bench-top microscope, can be used on site during an excavation, or on unmoveable objects such as wall paintings, cave paintings, mosaics etc. When compared to a bench-top microscope, the drawbacks of a probe include reduced signal intensity, spatial and spectral resolution, a limited choice in terms of lasers, a less-than-ideal ability to view and evaluate with a proper microscope the sample under examination, and the presence of vibrations that can hinder the analysis.
Handheld
This type of instrument is easy to use and is especially suited for the analysis of inorganic materials, for example during a mineral survey in the field. However it has an even more limited spatial and spectral resolution compared to the probe. It may also be difficult to set the power intensity at the sample to a suitable level, and having no microscope objective at the end of the instrument, a handheld Raman probe does not allow the analyst to inspect and choose the target region carefully.
Types of lasers
Most of the lasers commonly found in a cultural heritage laboratory are in the visible range: blue, green, red and far red. A laser in the near infrared can also be used, and lasers in the UV are becoming more common in Raman instruments. When choosing a laser in the visible range, a general rule of thumb is to choose the laser with a colour as similar as possible to that of the sample under examination. The Raman effect is based on light scattering, and absorption of the laser beam by the sample needs to be limited as much as possible: the more a laser beam is absorbed by the sample, the less of it is left for any scattering effect, and the weaker the Raman bands. Moreover, a significant absorption of a laser beam by a pigment or a dye is also likely to cause local overheating, which in turn can degrade or even burn the microscopic portion of the sample under examination. This is a very confined damage, as usually the burnt area is only a few micrometres across and is invisible to the naked eye, but it is damage nonetheless. The laser-degraded area may also give rise to a Raman spectrum which can be mistaken for a different material.
The intensity of the Raman signal is inversely proportional to the excitation wavelength: for example, a sample will give a stronger Raman signal if it is analysed with a blue laser rather than a red laser.
There are of course exceptions to the following ‘rules’, but in general for a red or orange pigment it would be best to use a He–Ne laser (632 nm) or a krypton ion laser (647 nm) or any other true-red solid state laser. It is not normally advisable to use a green laser. For blue pigments it is best to use a blue (argon ion laser, 488 nm) or a green laser (argon ion, 514.5 nm; Nd:YAG, 532 nm; or any other solid state green laser). White and yellow pigments can usually be analysed with any type of laser. Far red (solid state lasers usually between 780 and 785 nm) and near infrared lasers (Nd:YAG, 1064 nm, usually found on FT-Raman spectrometers) are particularly suitable for dyes and organic materials, but tend to burn dark inorganic materials as the latter absorb rather than scatter such laser radiation.
How to set up a protocol
It is advisable to do a preliminary test on the specimen under the microscope using a very low laser power (well below 1 mW). The laser power can be progressively increased as needed to obtain a good spectrum, always making sure that the sample is not being damaged by the laser irradiation. Commercial Raman microscopes usually come equipped with a set of neutral density filters; these allow fine-tuning of the laser power at the sample reducing it from 100% to below 1% of the maximum intensity. Please remember that laser-induced damage to the sample can occur even if no obvious change is visible under the microscope: if the Raman bands change on the screen within a second or two from the start of the analysis, it is likely that the specimen is being adversely affected by the laser beam.
Pitfalls and drawbacks
Fluorescence
The laser beam can excite electronic transitions that may mask the Raman signal; if any Raman peaks are visible at all, they appear as if they are just coming out of a very bumpy or hilly baseline. In some cases, fluorescence can be reduced by using a laser beam with a longer wavelength. Many organic dyes give rise to significant fluorescence and are not usually analysed by Raman. An oil-based binding medium often generates enough fluorescence to hide most of the Raman spectral features of the pigment or dye mixed with it (see Fig. 2).
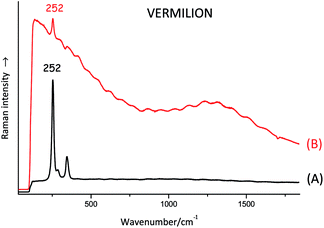 |
| Fig. 2 (A) Raman spectrum of the red pigment vermilion on plaster, showing no fluorescence (note the flat baseline) and clearly defined bands; (B) Raman spectrum of vermilion in an oil paint, showing significant fluorescence (raised and ‘bumpy’ baseline); the main peak of vermilion only just emerges from the fluorescent baseline. | |
Stability and focusing issues
One of the most frustrating practical aspects in the Raman analysis of relatively light objects is a loss of focus during the analysis, due to small movements of the object under the microscope. For manuscripts for example, unless the page under observation is kept still, any air movement or floor vibration can alter the position of the page under the laser. It is critical to a successful outcome of the Raman analysis that the single particle under observation is kept in focus at all times. To avoid vibrations, or at least reduce them as much as possible, a variety of aids can be used. These include padded snake weights and glass weights, which can be positioned on the page under analysis as close as possible to the microscope objective in order to keep the area under observation flat and still.
Size restrictions
If only a traditional microscope set up is available for the analysis, then only relatively small objects can be analysed because large ones simply cannot fit under the microscope. This problem is more easily overcome if an open architecture system is available or if a fibre optic probe or hand-held Raman instrument can be used, as discussed at the beginning of this brief.
Interference
As mentioned above, if an oil binding medium is present in the pigment mixture, it can cause fluorescence which may mask the Raman signal of the pigment. It is much easier to analyse pigments mixed with a water-based binding medium (gum Arabic or animal glue for example).
Miscellaneous limitations
A Raman microscope using visible excitation is not particularly suitable for the analysis of binding media, whose spectrum is usually very weak and is swamped by that of the pigments or dyes. It is also of limited usefulness with many very dark materials that absorb most of the incident light, as they are prone to local overheating and laser-induced degradation.
Analysis of spectra
Identifying compounds on the basis of their Raman bands can be a complex operation, which requires a detailed knowledge of group theory and involves lengthy calculation. Realistically, this is rarely done and usually artists' materials are identified with the help of already existing spectral databases. Many of these are available in the published domain and can be used as a reference (see list at the end of this brief). Fig. 3 shows an example of a Raman spectrum from an unknown material matched with the Raman spectra of relevant reference materials.
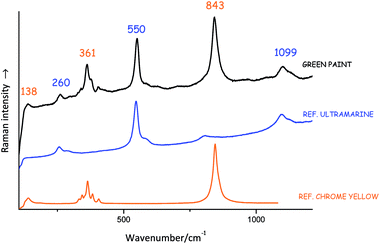 |
| Fig. 3 Example of Raman spectrum produced from a sample of green paint (top) compared to the spectra of two reference materials (middle and bottom). By overlapping the spectra it is possible to observe the correspondence of the peaks. | |
Lucia Burgio (Victoria and Albert Museum)
This Technical Brief was prepared by the Heritage Science Subcommittee and approved by the AMC on 24/04/15.
Further reading
- R. J. H. Clark, Chem. Soc. Rev., 1995, 24, 187 RSC.
- G. D. Smith and R. J. H. Clark, Raman Microscopy in Art History and Conservation Science, Rev. Conserv., 2001, 2, 92–106 CAS.
- G. D. Smith and R. J. H. Clark, Raman Microscopy in Archaeological Science, J. Archaeol. Sci., 2004, 31, 1137–1160 CrossRef PubMed.
-
L. Burgio, Analysis of Pigments on Manuscripts by Raman Spectroscopy: Advantages and Limitations, in The Technological Study of Books and Manuscripts as Artefacts – Research questions and analytical solutions, ed. S. Neate, D. Howell, R. Ovenden and A. M. Pollard, Archaeopress, 2011 Search PubMed.
- I. M. Bell, R. J. H. Clark and P. J. Gibbs, Raman Spectroscopic Library of Natural and Synthetic Pigments (pre- ∼1850 AD), Spectrochim. Acta, Part A, 1997, 53, 2159–2179 CrossRef.
- L. Burgio and R. J. H. Clark, Library of FT-Raman spectra of pigments, minerals, pigment media and varnishes, and supplement to existing library of Raman spectra of pigments with visible excitation, Spectrochim. Acta, Part A, 2001, 57, 1491–1521 CrossRef CAS.
- M. Bouchard and D. C. Smith, A Catalogue of 45 Reference Raman Spectra of Minerals concerning research in Art History or Archaeology (corroded metals & stained glass), Spectrochim. Acta, Part A, 2003, 59(10), 2247–2266 CrossRef CAS.
- P. Vandenabeele, L. Moens, H. G. M. Edwards and R. Dams, Raman spectroscopic database of azo pigments and application to modern art studies, J. Raman Spectrosc., 2000, 31, 507–517 CrossRef.
- D. Bersani, Raman database of minerals, University of Parma, http://www.fis.unipr.it/phevix/ramandb.php, accessed 18 December 2013.
- P. Vandenabeele, B. Wehling, L. Moens, H. Edwards, M. De Reu and G. Van Hooydonk, Analysis with micro-Raman spectroscopy of natural organic binding media and varnishes used in art, Anal. Chim. Acta, 2000, 407, 261–274 CrossRef CAS.
- N. C. Scherrer, S. Zumbuehl, F. Delavy, A. Fritsch and R. Kuehnen, Synthetic organic pigments of the 20th and 21st century relevant to artist's paints: Raman spectra reference collection, Spectrochim. Acta, Part A, 2009, 73(3), 403–580 CrossRef PubMed.
- P. Ropret, S. A. Centeno and P. Bukovec, Raman identification of yellow synthetic organic pigments in modern and contemporary paintings: Reference spectra and case studies, Spectrochim. Acta, Part A, 2008, 69, 486–497 CrossRef PubMed.
- E. Huang, Raman spectroscopy study of 15 gem minerals, J. Geol. Soc. China, 1999, 42, 301–308 CAS.
- C. M. Schmidt and K. A. Trentelman, 1064 dispersive Raman micro-spectroscopy for the in situ identification of organic red colorants, e-Preserv. Sci., 2009, 6, 10–21 CAS.
|
This journal is © The Royal Society of Chemistry 2015 |