DOI:
10.1039/C5AY00042D
(Technical Note)
Anal. Methods, 2015,
7, 6443-6448
Quantification of N-hydroxysuccinimide and N-hydroxysulfosuccinimide by hydrophilic interaction chromatography (HILIC)†
Received
7th January 2015
, Accepted 16th June 2015
First published on 22nd June 2015
Abstract
N-Hydroxysuccinimide (NHS) esters are the most important activated esters used in many different bioconjugation techniques, such as protein labelling by fluorescent dyes and enzymes, surface activation of chromatographic supports, microbeads, nanoparticles, and microarray slides, and also in the chemical synthesis of peptides. Usually, reactions with NHS esters are very reliable and of high yield, however, the compounds are sensitive to air moisture and water traces in solvents. Therefore, the quantification of NHS would be a very helpful approach to identify reagent impurities or degradation of stored NHS esters. No robust and sensitive method for the detection of NHS (or the more hydrophilic sulfo-NHS) has been reported yet. Here, a chromatographic method based on HILIC conditions and UV detection is presented, reaching a detection limit of about 1 mg L−1, which should be sensitive enough for most of the applications mentioned above. Exemplarily, the hydrolytic degradation of a biotin-NHS ester and a purity check of a fluorescent dye NHS ester are shown. An important advantage of this approach is its universality, since not the structurally variable ester compound is monitored, but the constant degradation product NHS or sulfo-NHS, which avoids the necessity to optimize the separation conditions and facilitates calibration considerably.
1. Introduction
A multitude of NHS esters are commercially available, mainly for bioconjugation purposes. In relation to other active compounds, NHS esters are relatively stable and can be stored for months under dry conditions. However, in practice, hydrolysis of NHS esters is a frequent reason for suboptimal conjugation results or even a complete failure of the respective derivatization reactions. Therefore, quality control of new batches of chemicals or the test of already opened vials of NHS esters is highly recommendable. This can be achieved by the quantitative determination of NHS esters and their respective carboxylic acids.
More than 50 years ago, N-hydroxysuccinimide (NHS) was proposed by the group of Anderson for the preparation of active esters.1–3 These compounds are extremely useful for peptide synthesis and the preparation of all kinds of bioconjugates. The favorable reactivity of NHS esters, their relative stability towards hydrolysis, good crystallizability and low toxicity have made NHS esters some of the most widely used chemical reagents. In Fig. 1 the structures of the compounds of interest are shown and in Table 1, some properties are collected.
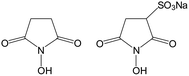 |
| Fig. 1 Chemical structures of N-hydroxysuccinimide (NHS) and N-hydroxysulfosuccinimide (sulfo-NHS), sodium salt. | |
Table 1 Properties of NHS and sulfo-NHS
|
N-Hydroxysuccinimide (NHS) |
N-Hydroxysulfosuccinimide (sulfo-NHS) |
Molecular formula |
C4H5NO3 |
C4H4NO6SNa (sodium salt) |
CAS no. |
6066-82-6 |
106627-54-7 |
Molecular Mass |
115.09 g mol−1 |
217.13 g mol−1 |
pKa |
6.0 (ref. 13) |
|
Extinction coefficient |
8200 mol L−1 cm−1 (ref. 14) |
|
8600 mol L−1 cm−1 (ref. 15) |
|
9700 mol L−1 cm−1 (ref. 16) |
|
Some of the most important NHS ester reagents are biotin-NHS and other esters of biotin derivatives. Furthermore, nearly all fluorescent dyes (fluoresceins, rhodamines, cyanins, bodipy dyes, coumarins, and others) used in bioanalysis can be purchased as NHS esters, often as a simple reagent kit. Another popular application is their use as activated carrier materials in affinity chromatography or affinity extraction. Cross-linked beaded agarose (known as Sepharose) is one of the most frequently used materials, which can be obtained as NHS-preactivated beads or columns. In addition, NHS-activated magnetic beads are commercially available. NHS esters are used for a multitude of scientific purposes in biochemistry, biotechnology and bioanalysis. A typical method is the activation of a hapten carboxylic acid for conjugation on a protein carrier to obtain a suitable immunogen for antibody generation.4,5 Even complete virus particles have been selectively modified with NHS esters.6 Cross-linking of proteins or biochemical complexes are other applications of NHS (esters). The selectivity of NHS esters towards different amino acids and the applicability of NHS cross-linkers to protein–protein interactions were examined by mass spectrometry.7–9
Anderson et al. emphasized the excellent water solubility of NHS, which facilitates peptide synthesis under aqueous conditions. However, in most cases, the corresponding esters are by far less polar than NHS itself. Therefore, most NHS esters can be analyzed or purified on reversed phase columns without difficulty. For nonpolar carboxylic acids, the resulting NHS esters are nearly insoluble in water. Therefore, more hydrophilic alcohols were developed to improve the solubility of the respective esters. N-Hydroxysulfosuccinimide (sulfo-NHS) is the most popular one, which was introduced by Staros.10 It is often used for the catalysis of aqueous condensation reactions with water-soluble carbodiimides, such as EDC (1-ethyl-3-(3-dimethylaminopropyl)carbodiimide).11 Another application was presented by Grumbach and Veh, who prepared aqueous stock solutions of a long-chain derivative of biotin, activated by sulfo-NHS.12 Organic solvents are completely avoided with these reagents. Other valuable reagents based on sulfo-NHS are hydrophilic protein cross-linkers.10
In most cases, analytical efforts are focused on the carboxylic part of the NHS ester or the ester itself. The released NHS is considered to be a byproduct or an impurity. In some studies, either the product (e.g. an amide) was determined or the reaction was quenched with slightly acidic buffers, which enabled the determination of residual NHS esters.
Also, some quite indirect methods have been reported, as in a recent paper, in which the residual amine was determined by a spectrophotometric method based on 2,4,6-trinitrobenzenesulfonic acid (TNBS) derivatization.17 Others used titration of carboxylate groups, elemental analysis and depletion methods for the monitoring of NHS ester kinetics.18 Even biochemical assays (e.g. BSA conjugation) have been used to determine the hydrolysis rate of biotin sulfo-NHS esters.12 In the paper by Cuatrecasas and Parikh,19 tritium-labelled alanine in combination with liquid scintillation was used to determine the hydrolysis rate of NHS activated agarose. Alternatively, amino acid analyses were performed after the hydrolysis with constant-boiling hydrochloric acid.
Only a few researchers have realized that the determination of free NHS is a powerful and very general method to monitor any reaction based on NHS or sulfo-NHS esters.
Miron and Wilchek presented a spectrophotometric method for the determination of NHS at 260 nm.16 In this protocol, ammonium hydroxide is used to adjust the optimal pH. However, a very basic pH (e.g. a NaOH solution) leads to the destruction of NHS. The authors already mentioned that UV absorbing substances, such as aromatic amino acids might cause some interference. Nevertheless, under favorable conditions, this method can be useful.17,20
In the textbook Bioconjugate Techniques, the author Greg T. Hermanson states: “Unfortunately, the sensitivity of assays using absorption usually does not allow for measuring the rate of reaction in an actual cross-linking procedure”.21 In addition, the strong UV absorbance of NHS at 260 nm may also lead to severe interference with any protein or DNA determination based on UV. Furthermore, it was reported that sulfo-NHS even interferes with the popular and usually robust BCA protein test.22
The literature overview shows that NHS and sulfo-NHS are determined only rarely and with complicated and mostly indirect means. The major reason for this surprising situation might be the difficulty to analyze NHS or sulfo-NHS by using conventional chromatographic techniques. Therefore, we wanted to develop a robust and convenient method to determine these analytes by HPLC. Due to the extremely high polarity of both compounds, there is essentially no retention on reversed-phase columns. In contrast, the use of hydrophilic interaction liquid chromatography (HILIC),23 introduced by Alpert around 1990,24 seems to be a good choice to resolve this separation problem. Our aim was to develop a chromatographic method for the determination of NHS or sulfo-NHS compatible with UV detection.
2. Materials and methods
2.1. Chemicals
NHS (98%, # 130672, Sigma-Aldrich), sulfo-NHS, and sodium salt (≥98%, # 56485, Sigma-Aldrich) have been used. Ultrapure water, which was used for samples and mobile phases, was purified by using a stationary MilliQ system (Merck-Millipore). For the analysis of the real samples, biotin-NHS (Thermo Scientific) and Cyanine5 NHS esters (95%, Lumiprobe Life Science Solutions, # 13020; similar to the dye Cy5) have been used. For the preparation of mobile phases for chromatographic analysis, acetonitrile (Fischer Chemicals, LC-MS grade), MilliQ water, ammonium acetate (for HPLC, ≥99%, Fluka, # 17836) and 10% ammonium hydroxide in water (HPLC grade, Fluka, # 17837) were used. Molecular sieves (4 Å, # 208604, beads, 8–12 mesh) were obtained by Sigma-Aldrich.
2.2. Columns and chromatography
A silica-based Thermo Syncronis HILIC column (150 × 3 mm) with a particle size of 3 μm was used for experiments at pH values of up to 8. A polymer-based SeQuant ZIC-pHILIC column from Merck (150 × 2.1 mm, 5 μm) was used for experiments in a pH range between 10 and 11 (for results see ESI†). All HPLC measurements were performed on an Agilent 1200 HPLC system with UV detection at 220 nm and 260 nm.
2.3. Chromatographic method
A zwitterionic silica-based HILIC column (Thermo Syncronis HILIC, 150 mm × 3 mm, 3 μm) was used for the isocratic separation of NHS and sulfo-NHS samples in a 10 mM ammonium acetate buffer (pH 7.5). The mobile phase consisted of 90% acetonitrile and 10% of 10 mM aqueous ammonium acetate (pH 7.5 before mixing). The column temperature was set to 30 °C. The system was equilibrated for 10 minutes before each run. The flow rate was 0.4 mL min−1 and the injection volume was 1 μL. Detection was performed by UV at 220 and 260 nm; and the total run time was 20 min. The detection limit was defined as a signal exceeding 3 times the noise of the baseline and the limit of quantitation as 6 times the noise (rounded values).
2.4. Analysis of NHS impurities in biotin NHS ester and Cyanine5 NHS ester
For the analysis of real samples, NHS esters of biotin and Cyanine5 were chosen. A silica-based column (Thermo Syncronis HILIC, 150 × 3 mm, 3 μm) was used with the protocol given above. The samples were dissolved in 10 mM ammonium acetate buffer, pH 7.5. The injection volume was 1 μL. Detection was performed at 220 nm.
2.5. Analysis of biotin NHS ester hydrolysis
The biotin NHS ester samples were dissolved in 10 mM ammonium acetate buffer adjusted to pH 7.0 with acetic acid to control the hydrolysis rate. At pH 6, NHS esters can be considered to be stable for some hours (not shown). The injection volume was 1 μL.
2.6. Analysis of biotin NHS ester spiked with additional NHS
For spiking experiments, solutions of NHS and biotin-NHS esters with the same concentration (1 g L−1) were prepared in dried ACN. For drying, molecular sieves with a pore size of 4 Å were employed. Samples, containing 0% to 10% of NHS solution and 100% to 90% of biotin-NHS ester solution respectively, have been prepared. A Thermo Syncronis HILIC column (15 cm × 3 mm, 3 μm) was employed with a mobile phase consisting of 90% acetonitrile and 10% of 10 mM aqueous ammonium acetate solution with a pH of 7.5. A column temperature of 30 °C, a flow rate of 0.4 mL min−1, an injection volume of 1 μL and detection by UV absorbance at 220 nm were used.
3. Results and discussion
3.1. Determination of NHS and sulfo-NHS
Based on the standard conditions used in HILIC separation, useful isocratic conditions were found to lead to convenient retention times. A neutral ammonium acetate buffer with a high concentration of acetonitrile proved to be suitable. Due to isocratic conditions and low injection volumes, equilibration issues, which are occasionally observed in HILIC separations, are avoided. NHS and sulfo-NHS could be completely separated with retention times of about 5.3 min and 12 min (Fig. 2). Based on the new method, calibration lines for the concentration range between 0.5 and 100 mg L−1 for NHS and 1 and 100 mg L−1 for sulfo-NHS have been determined. In addition, limits of detection (LOD) of 1 mg L−1 and 0.5 mg L−1, respectively, and limits of quantification (LOQ) of 3 mg L−1 and 1.5 mg L−1, respectively, have been calculated.
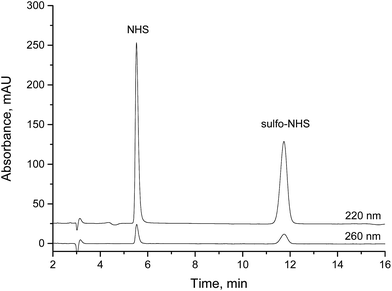 |
| Fig. 2 Separation of NHS and sulfo-NHS on a HILIC column (isocratic, 10% 10 mM ammonium acetate, pH 7.5, 90% acetonitrile, 30 °C), sample was 1 μL of 0.1 g L−1 NHS and 0.1 g L−1 sulfo-NHS. | |
3.2. Calibration lines of NHS and sulfo-NHS and validation
A calibration for NHS and sulfo-NHS was performed on the silica-based HILIC column, (Fig. 3a and b). By preliminary experiments it was found that a linear range of about 1–100 mg L−1 could be expected. Concentrations above 100 mg L−1 lead to non-linear calibration curves (not shown), which in some cases might be still acceptable. The detection limit of 1 or 0.5 mg L−1 is in the range, which can be expected with a standard UV-detector and a compound with extinction coefficients as given in Table 1.
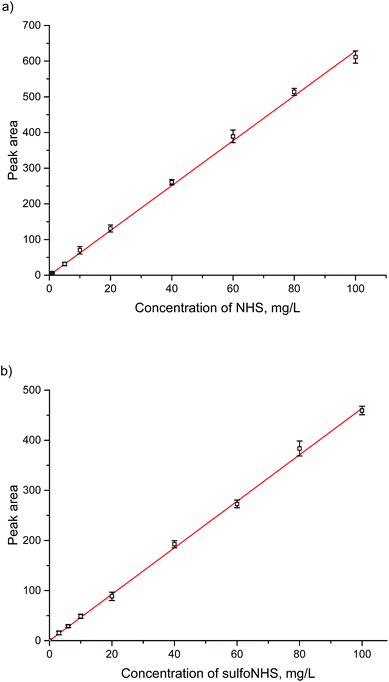 |
| Fig. 3 Calibration lines for NHS (a) and sulfo-NHS (b) obtained on a silica-based HILIC column. Limit of detection 1 mg L−1 (NHS) and 0.5 mg L−1 (sulfo-NHS); Limit of quantification: 3 mg L−1 and 1.5 mg L−1, respectively. | |
The reproducibility of the method was tested in terms of intra-assay precision (Table 2) and day-to-day precision (Table 3) at three different concentration levels. The latter is based on three replicates per day and a series of five consecutive days.
Table 2 Intra-assay reproducibility (NHS)
Concentration [mg L−1] |
Replicates n |
Mean [peak area] |
SD (s) [peak area] |
Relative SD [%] |
20 |
10 |
130.0 |
1.9 |
1.5 |
100 |
10 |
655.5 |
1.6 |
0.24 |
400 |
10 |
2576.6 |
4.7 |
0.18 |
Table 3 Day-to-day reproducibility (NHS)
Concentration [mg L−1] |
Replicates n [days] |
Mean [peak area] |
SD (s) [peak area] |
Relative SD [%] |
20 |
5 |
124.7 |
5.4 |
4.3 |
100 |
5 |
651.5 |
14.3 |
2.2 |
400 |
5 |
2603.6 |
45.4 |
1.7 |
3.3. Determination of NHS as a degradation product in NHS esters
Under weakly acidic conditions, chromatographic analysis of many NHS esters can be performed on standard RP columns. However, some compounds, such as highly sulfonated fluorescent dyes and other hydrophilic compounds, might be difficult to analyze in this way. In addition, a new method might be required for each different NHS ester. A faster and more general method seems to be the determination of NHS in a NHS ester sample. Since most NHS esters are delivered as purified fine chemicals, initially, the content of free NHS should be very low. Degradation due to wrong or extended storage would be always accompanied by a corresponding increase of the content of free NHS. In addition, quantitation is facilitated, since only one cheap calibration substance (NHS or sulfo-NHS) is necessary, not the purified NHS esters or their corresponding carboxylic acids, which are not available in defined purities in most cases. The quantitative determination of NHS in NHS esters should be a quick and reliable method to verify the quality of the respective active ester. We have analyzed biotin-NHS and a NHS ester of a fluorescent dye (Cyanine5) by our method. The NHS content in spiked samples (Fig. 4) and partially hydrolyzed NHS esters (Fig. 5) could be quantified. Finally, the examination of a commercial fluorescent dye is shown in Fig. 6. All relevant compounds, the activated ester, the carboxylic acid and NHS (both degradation products) could be identified. The purity determination based on NHS and its external calibration leads to a value in good accordance with the declaration of the manufacturer.
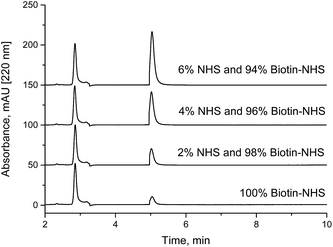 |
| Fig. 4 Spiking experiments based on commercial biotin-NHS with added NHS in different ratios. The percentages are given as nominal values. This means that 100% biotin-NHS already contained some NHS as the impurity. The biotin-NHS peak can be seen at 2.8 min. | |
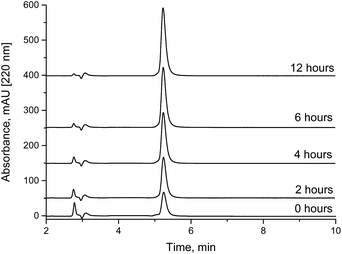 |
| Fig. 5 Analysis of a freshly prepared (0 hours) and a partially hydrolyzed (lines above) biotin-NHS ester solution. The main peak shows the impurity or the degradation product N-hydroxysuccinimide (NHS). The sample was 1 μL of 0.1 g L−1 biotin-NHS in 10 mM ammonium acetate (pH 7.0), in which the hydrolysis was performed. It can be seen that the biotin-NHS peak at 2.8 min decreases with time, whereas the NHS peak at 5.3 min increases. | |
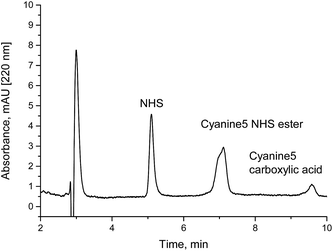 |
| Fig. 6 Analysis of a freshly prepared Cyanine5 NHS ester solution (activated fluorescent dye). The peak at about 5 minutes shows the impurity N-hydroxysuccinimide (NHS). The sample was 1 μL of 0.1 g L−1 Cyanine5 NHS in 10 mM ammonium acetate (pH 7.5). Based on an external calibration (Fig. 3) a NHS content of 5.7% was calculated. The manufacturer claims a purity of 95%. | |
4. Conclusions
The chromatographic determination of the very hydrophilic compounds NHS and sulfo-NHS was considered to be difficult. It could be shown that the application of HILIC columns with mobile phases preferentially at neutral pH is a simple, robust and sensitive method for many experiments, in which (sulfo-)NHS esters are involved. A UV detection at 220 nm and 260 nm is possible, the latter being potentially less prone to spectral interference. The new method might be useful for the purity determination of NHS esters, monitoring of homogeneous or heterogeneous bioconjugations, such as the biotinylation of proteins, the immobilization of biomolecules on polymeric carriers and the labeling of proteins with fluorescent dyes. An exceptional benefit of this approach is its universality. Since (sulfo-)NHS is the analyte of interest and not the variable part of the reaction, the separation conditions do not have to be optimized and a standard protocol might be applied routinely, particularly for quality control purposes.
Acknowledgements
This work was supported by the BAM Federal Institute for Materials Research and Testing, Berlin, Germany, which belongs to the German Federal Ministry for Economic Affairs and Energy. We would like to thank Dr. A. Lehmann, BAM Div. 1.8, for providing the silica-based HILIC column.
References
- G. W. Anderson, J. E. Zimmerman and F. M. Callahan,
N-Hydroxysuccinimide esters in peptide synthesis, J. Am. Chem. Soc., 1963, 85, 3039 CrossRef CAS
.
- G. W. Anderson, J. E. Zimmerman and F. M. Callahan, The use of esters of n-hydroxysuccinimide in peptide synthesis, J. Am. Chem. Soc., 1964, 86, 1839–1842 CrossRef CAS
.
- G. W. Anderson, The use of activated esters in peptide synthesis, Metabolism, 1964, 13, 1026–1031 CrossRef CAS
.
- M. G. Weller, L. Weil and R. Niessner, Determination of triazine herbicides by ELISA – Optimization of enzyme tracer synthesis, Fresenius. J. Anal. Chem., 1992, 343, 51–52 CrossRef
.
- S. Ramin and M. G. Weller, Extremely sensitive and selective antibodies against the explosive 2,4,6-trinitrotoluene by rational design of a structurally optimized hapten, J. Mol. Recognit., 2012, 25, 89–97 CrossRef CAS PubMed
.
- A. Chatterji, W. F. Ochoa, M. Paine, B. R. Ratna, J. E. Johnson and T. W. Lin, New addresses on an addressable virus nanoblock: Uniquely reactive lys residues on cowpea mosaic virus, Chem. Biol., 2004, 11, 855–863 CrossRef CAS PubMed
.
- S. Mädler, C. Bich, D. Touboul and R. Zenobi, Chemical cross-linking with NHS esters: A systematic study on amino acid reactivities, J. Mass Spectrom., 2009, 44, 694–706 CrossRef
.
- S. Mädler, M. Seitz, J. Robinson and R. Zenobi, Does Chemical Cross-Linking with NHS Esters Reflect the Chemical Equilibrium of Protein–Protein Noncovalent Interactions in Solution?, J. Am. Soc. Mass Spectrom., 2010, 21, 1775–1783 CrossRef
.
- S. Kalkhof and A. Sinz, Chances and pitfalls of chemical cross-linking with amine-reactive N-hydroxysuccinimide esters, Anal. Bioanal. Chem., 2008, 392, 305–312 CrossRef CAS
.
- J. V. Staros,
N-Hydroxysulfosuccinimide active esters: Bis(N-hydroxysulfosuccinimide) esters of two dicarboxylic acids are hydrophilic, membrane-impermeant, protein cross-linkers, Biochemistry, 1982, 21, 3950–3955 CrossRef CAS
.
- J. V. Staros, R. W. Wright and D. M. Swingle, Enhancement by N-hydroxysulfosuccinimide of water-soluble carbodiimide-mediated coupling reactions, Anal. Biochem., 1986, 156, 220–222 CrossRef CAS
.
- I. M. Grumbach and R. W. Veh, Sulpho-N-hydroxysuccinimide activated long chain biotin, J. Immunol. Methods, 1991, 140, 205–210 CrossRef CAS
.
- D. E. Ames and T. F. Grey, The synthesis of some N-hydroxyimides, J. Chem. Soc., 1955, 631–636 RSC
.
- J. Carlsson, H. Drevin and R. Axen, Protein thiolation and reversible protein-protein conjugation - N-Succinimidyl 3-(2-pyridyldithio)propionate, a new heterobifunctional reagent, Biochem. J., 1978, 173, 723–737 CAS
.
- O. Adalsteinsson, A. Lamotte, R. F. Baddour, C. K. Colton, A. Pollak and G. M. Whitesides, Preparation and magnetic filtration of polyacrylamide gel beads containing a ferrofluid and covalently immobilized proteins, J. Mol. Catal., 1979, 6, 199–225 CrossRef CAS
.
- T. Miron and M. Wilchek, A spectrophotometric assay for soluble and immobilized n-hydroxysuccinimide esters, Anal. Biochem., 1982, 126, 433–435 CrossRef CAS
.
- A. Gao, X. L. Yang, C. Zhang, G. B. Long, J. Pu, Y. H. Yuan, H. B. Liu, Y. L. Li and F. Liao, Facile spectrophotometric assay of molar equivalents of N-hydroxysuccinimide esters of monomethoxyl poly-(ethylene glycol) derivatives, Chem. Cent. J., 2012, 6, 142 CrossRef CAS PubMed
.
- G. A. J. Besselink, T. Beugeling and A. Bantjes,
N-Hydroxysuccinimide-activated glycine–sepharose - Hydrolysis of activated groups and coupling of amino-compounds, Appl. Biochem. Biotechnol., 1993, 43, 227–246 CrossRef CAS
.
- P. Cuatrecasas and I. Parikh, Adsorbents for affinity chromatography - Use of N-hydroxysuccinimide esters of agarose, Biochemistry, 1972, 11, 2291–2299 CrossRef CAS
.
- H. S. Kim, Y. S. Kye and D. S. Hage, Development and evaluation of N-hydroxysuccinimide-activated silica for immobilizing human serum albumin in liquid chromatography columns, J. Chromatogr. A, 2004, 1049, 51–61 CrossRef CAS
.
-
G. T. Hermanson, Bioconjugate Techniques, Academic Press, Amsterdam, 3rd edn, 2013, p. 278 Search PubMed
.
- S. K. Vashist, B. B. Zhang, D. Zheng, K. Al-Rubeaan, J. H. T. Luong and F. S. Sheu, Interference of N-hydroxysuccinimide with bicinchoninic acid protein assay, Anal. Biochem., 2011, 417, 156–158 CrossRef CAS PubMed
.
- P. Jandera, Stationary and mobile phases in hydrophilic interaction chromatography: a review, Anal. Chim. Acta, 2011, 692, 1–25 CrossRef CAS PubMed
.
- A. J. Alpert, Hydrophilic-interaction chromatography for the separation of peptides, nucleic acids and other polar compounds, J. Chromatogr., 1990, 499, 177–196 CrossRef CAS
.
Footnote |
† Electronic supplementary information (ESI) available. See DOI: 10.1039/c5ay00042d |
|
This journal is © The Royal Society of Chemistry 2015 |