DOI:
10.1039/C4AY01343C
(Paper)
Anal. Methods, 2015,
7, 99-106
A real-time immuno-PCR assay for the detection of tetrabromobisphenol A
Received
5th June 2014
, Accepted 19th August 2014
First published on 19th August 2014
Abstract
In this study, a reliable and ultra-sensitive indirect competitive real-time immuno-PCR (rt-iPCR) was established for the determination of tetrabromobisphenol A (TBBPA). The optimal hapten, artificial immunogen, coating antigen, and polyclonal anti-TBBPA antibodies were successfully prepared. For optimizing rt-iPCR, several physiochemical factors, such as optimal coupling concentration of immunogen and antibody, and the amounts of streptavidin and biotinylated DNA, are discussed. Using this proposed rt-iPCR assay, TBBPA could be determined in the range from 10 pg L−1 to 10 ng L−1, with a detection limit of 2 pg L−1. This rt-iPCR was selective, with low cross-reactivity with TBBPA analogs. Finally, seabed sediment samples were analyzed by rt-iPCR and liquid chromatography. The tested results were demonstrated to be receivable and accurate. The average recovery was between 89.8% and 113.6%. We think this developed rt-iPCR will be very useful in the field of environmental science.
1. Introduction
Tetrabromobisphenol A (TBBPA) is usually synthesized by brominating bisphenol A. As an additive or reactive flame retardant, TBBPA is the critical material in preparation of flame-retardant epoxy resin. The global consumption of TBBPA has increased from 64
000 t in 1994 to 180
000 t in 2007.1 The worldwide use of TBBPA has transferred this compound from different processes and sources into the environment. Trace concentrations of TBBPA have been detected in many abiotic and biotic media, including stationary air samples:2 <3–180 ng m−3, dust samples:3 2300–2900 pg per day, wastewater:4 0.013–0.031 ng mL−1, soils:5 3.4–32.2 ng g−1 dw, agricultural soil:5 0.3 ng g−1 dw, human tissue (serum:6 <1–3.4 pmol g−1 lw; breast milk:7 7000 ng kg−1 lw), and wild animals (birds of prey:8 13 pg g−1; dolphin fat:9 0.1–418 μg kg−1 lw). As early as 2003, TBBPA has received the attention of the world Greenpeace organization, because of its potential environmental harm. Recent studies have proved that TBBPA has the characteristics of persistence, bioaccumulation and biotoxicity.10 On long-time exposure, TBBPA will arrest the natural growth of biotic brain and bones.10 And, because its molecular structure is comparable to that of thyroxin, TBBPA is classified as an endocrine-disrupting chemical.11 In consequence, TBBPA is listed in the convention on the protection of the marine environment of the North-East Atlantic as a hazardous substance.
Commonly used methods for analysis of TBBPA are gas and liquid chromatography techniques.12–16 And these chromatography techniques have been successfully applied to detect TBBPA in various types of environmental samples. However, most of these samples were collected from relatively high concentration areas, like BFRs producing factory/department, the river flowing near industrial area, municipal sewage plants, etc. The amounts of TBBPA in other areas or samples, which contain low concentration, are reported rarely. This may be because the detection limit of chromatography methods is relatively high, and not suitable for determining trace organic pollutants. Meanwhile, chromatography techniques have some drawbacks such as they are expensive and time-consuming and they require complex pretreatment procedures. So, improving the limit-of-detection is a significant challenge in the research of analysis methods. The immunoassay, especially the real-time immuno-PCR (rt-iPCR), may be the most appropriate one.
The immuno-PCR method,17 combining the ELISA methodology with the signal amplification of PCR, has the advantages of ultra-high sensitivity and good quantification capabilities as a result of the excellent linearity and compatibility with established ELISA protocols.18 The detection has been proved to be 10
000-fold more sensitive than conventional enzyme-linked immunosorbent assay.19 This method has been used to detect antigens associated with autoimmune diseases,20 cancer,21,22 and bacterial toxin.23 However, reports about using immuno-PCR methods to detect environmental pollutants are less. The development of the instrumentation employed in the signal detection of immuno-PCR has resulted in the development of rt-iPCR. Compared to iPCR, rt-iPCR has displayed improved statistical validation of accuracy. Inter-assay error is typically 5–10% for rt-PCR vs. 15–20% for iPCR. The primary advantage of using rt-iPCR in place of iPCR is the immediate interpretation of positive data (quantification of proteins) as the PCR proceeds.24
In this study, a rt-iPCR immunoassay has been developed. Diverse TBBPA haptens, immunogens, and relative polyclonal antibodies were prepared. Procedures of this proposed rt-iPCR have been optimized, and the standard curve was established by plotting the average threshold (Ct) values against the known TBBPA concentrations. This rt-iPCR was implemented to detect TBBPA residues in seabed sediments. The tested results were comparable to those of HPLC. The accuracy and sensitivity of the testing results were good.
2. Materials and methods
2.1 Reagents and buffers
The TBBPA standard was purchased from J&K Chemical (Beijing, China). Hot Start Fluorescent PCR Core Reagent Kits (SYBR GREEN I), DNA PCR kit, UNIQ-10 PCR DNA Extraction Kit, and streptavidin were purchased from Sangon Biotech (Shanghai, China). Bovine serum albumin (BSA), ovalbumin (OVA), N,N-dimethylformamide (DMF), N-hydroxysuccinimide (NHS), N,N′-dicyclohexylcarbodiimide (DCC), and dimethyl sulfoxide (DMSO) were purchased from Sinopharm, China. All reagents were of analytical grade unless specified otherwise. Phosphate-buffered saline (PBS: NaCl 137 mmol L−1, KCl 2.7 mmol L−1, Na2HPO4 10 mmol L−1, KH2PO4 2 mmol L−1), carbonate buffer solution (CBS: 15 mmol L−1 Na2CO3, 34.9 mmol L−1 NaHCO3), and PBST (PBS with 0.05% Tween 20) were prepared in our laboratory.
2.2 Synthesis of TBBPA artificial antigens
TBBPA is a kind of small molecule organic matter, which does not contain functional groups for connecting with proteins directly. For successfully synthesizing TBBPA artificial antigens, TBBPA hapten (name: 2-(2,6-dibromo-4-(2-(3,5-dibromo-4-hydroxyphenly)propan-2-yl))acetic acid, C17H14Br4O4, m.w.: 601.92) must be prepared first. The synthetic procedures were described in our previous study25 and are illustrated in Fig. 1. For coupling TBBPA hapten with carrier protein, different coupling methods, activated ester and mixed anhydride method, were applied to covalently couple hapten to proteins, using the terminal carboxyl group on TBBPA hapten. The prepared protein–hapten conjugates, BSA–TBBPA and OVA–TBBPA, were used as the immunogen and the coating antigen, respectively. The conjugating mechanisms are displayed in Fig. 1. The prepared conjugates were characterized using a UV-vis spectrophotometer. The UV-vis spectra exhibited the qualitative differences among hapten, carrier proteins and conjugates. Meanwhile, the concentration and the coupling ratios of BSA–TBBPA and OVA–TBBPA were also determined using a UV-vis spectrophotometric method.25,26
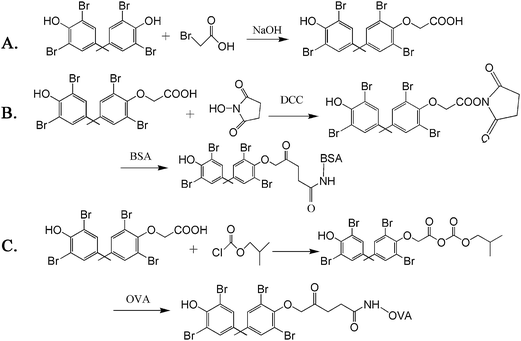 |
| Fig. 1 The synthetic route of (A) TBBPA hapten, (B) immunogen and (C) coating antigen. | |
2.3 Preparation of anti-TBBPA polyclonal antibodies and biotinylated antibody
As described in our previous studies,25 anti-TBBPA polyclonal antibodies (pAb-TBBPA) were separated and obtained from the serum of rabbits, after immunization with TBBPA–BSA for four months.
The as-prepared pAb-TBBPA yielded in our laboratory was biotinylated by biotin N-hydroxysuccinimide ester (BNHS). In brief, 5.0 mg of purified pAb-TBBPA was dissolved in 0.1 mol L−1 sodium carbonate buffer (pH 9.6) at a concentration of 1.0 mg mL−1. BNHS in DMSO was added in ten times molar excess to pAb-TBBPA solution. The mixture was stirred at room temperature for 4 h and then dialyzed against PBS for 3 days. The as-obtained biotinylated antibodies were stored at 4 °C before use.
2.4 Preparation of biotinylated reporter DNA
The biotinylated reporter DNA was prepared as per reported methods27,28 with slight modifications. The reporter 103-base-pair sequence double-stranded DNA originated from the pUC19 vectors and amplified by conventional PCR. The forward primer (5′-biotin-GTTGTAAAACGACGGCCAGT-3′) was biotinylated at the 5′ end to generate a biotin group in the DNA. The reverse primer, sequencing as 5′-GACAGTTACCAATGCTTAATCA-3′, was not modified. All reagents were prepared in the PCR tubes, containing 1 × Taq buffer, 200 μM dNTP, 1.5 mM MgCl2, 0.8 U Taq DNA polymerase, 0.4 μM forward primer, 0.4 μM reverse primer, and 25 ng template plasmid DNA (pUC19). PCR amplifying conditions were: initial denaturation at 94 °C for 4 min, followed by 30 cycles at 94 °C for 20 s, annealing at 55 °C for 20 s, and extending at 72 °C for 20 s. The final extension step was held at 72 °C for 3 min. The reporter DNA was purified and retrieved using a UNIQ-10 PCR DNA Extraction Kit.
2.5 Procedures of real-time immuno-PCR assay
The direct competitive real-time immuno-PCR assays were performed in 96-well microplates. Procedures for rt-iPCR were similar to the ELISA procedures except for adding biotinylated reporter DNA after adding avidin. The schematic diagram of rt-iPCR is displayed in Fig. 2. In order to enhance the adsorbability of the tubes, the PCR tubes were treated with 50 μL of 0.8% glutaraldehyde. The microplates were coated overnight with 20 μL per well of OVA-TBBPA diluent at 4 °C. After washing with PBST for 3 times, the unbound active sites were blocked with 200 μL per well of the blocking reagent, and were incubated at 37 °C for 60 min. After another washing step, the standard or sample solution (10 μL per well) and the biotinylated antibodies (10 μL per well) were added, and then the mixture was incubated for 60 min at 37 °C. After an additional washing step, 20 μL per well avidin was added to bind the attached biotinylated pAb-TBBPA, and then incubated for 60 min. After washing, the appropriate biotinylated template DNA was added and incubated at 37 °C for 30 min. Finally, the microplate was washed five times with PBST and five times with ultrapure water to remove unbound template DNA.
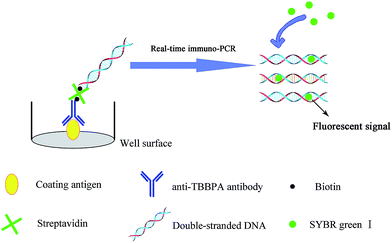 |
| Fig. 2 Diagram of the rt-iPCR principle. TBBPA coating antigen absorbed on the surface of the tube was used to compete with TBBPA in combination with biotinylated pAbs. Streptavidin was employed as a bridge between the biotinylated pAbs and biotinylated standard DNA. The DNA was amplified by the PCR process in a real-time PCR analysis system. | |
The rt-iPCR amplification process was carried out in PCR tubes directly, using a StepOnePlus™ real-time qPCR system (Applied Biosystems, USA). The PCR cycling parameters: initial degeneration at 94 °C for 4 min, followed by 35 cycles of denaturation at 94 °C for 20 s, annealing at 55 °C for 20 s, extending at 72 °C for 20 s, and another extension at 72 °C for 3 min. A melt procedure was done after the amplification process. The melt conditions: holding at 95 °C for 15 s, 60 °C for 1 min, and then an additional 0.3 °C s−1 until the temperature reached 95 °C. The fluorescence intensity was detected at the last step of each cycle during the PCR amplification process. A standard curve was determined by plotting the average Ct values against the known TBBPA concentrations.
2.6 Sample preparation
The seabed sediment samples used in this study were collected from the mouth of the Changjiang River in the southeast of China. Before treatment, these seabed sediment samples were air dried, sieved (2 mm), and stored frozen (−18 °C) in glass containers until use. Seabed sediment samples were extracted with 50 mL of n-hexane and dichloromethane (v/v = 4
:
1) for 0.5 h in an ultrasonic water bath at room temperature. The water level was adjusted to be slightly higher than the extraction solvent level. The mixture was centrifuged and extracted thrice. After being dried by anhydrous sodium sulfate, the total extract liquor was filtered (0.45 μm) and concentrated with a gentle stream of nitrogen to about 1 mL. For clean-up, 2 g Florisil was acidified with 0.5 mL of 37% HCl and mixed with anhydrous sodium sulfate. The mixture was left for 20 min at room temperature. And then, the mixture was transferred to a 20 mL glass column (20 cm × 0.8 cm), which contained two filter paper circles, 5 g Florisil and a bit of anhydrous sodium sulfate. The TBBPA transferred into the glass column was eluted by n-hexane and dichloromethane (v/v = 1
:
1). Under a gentle nitrogen stream, the eluant was evaporated to near dryness and the residue was dissolved in methanol.
2.7 HPLC analysis
The rt-iPCR tested results were verified by HPLC analysis, which was performed on an Agilent LC1100 HPLC system equipped with an Agilent Eclipse Plus C18 chromatographic column (250 mm × 4.6 mm, 5 μm), a high pressure pump, a column thermostat, a variable wavelength ultraviolet detector, and a vacuum de-air machine. The mobile phase consisted of methanol–water (80
:
20, v/v) at a flow rate of 0.3 mL min−1. The injection volume was 20 μL and detection was carried out at 280 nm. The temperature of the C18 was maintained at 35 °C.
3. Results and discussion
3.1. Suitable operating conditions and standard curves
The BSA-TBBPA and OVA-TBBPA conjugates and pAb-TBBPA were successfully synthesized in our laboratory, which had been characterized and discussed in the previous study.25 Concentrations of the prepared BSA-TBBPA, OVA-TBBPA and pAb-TBBPA were 7.69 mg mL−1, 4.24 mg mL−1 and 17.57 mg mL−1, respectively, as determined using Coomassie blue staining. The coupling ratio was 23 for BSA-TBBPA and was 16 for OVA–TBBPA.
The optimum concentrations of biotinylated pAb-TBBPA and coating antigen (OVA-TBBPA) tremendously influenced the sensitivity of rt-iPCR. According to checkerboard titration, the optimum reagent concentrations were the ones that resulted in the minimum Ct value. The results are presented in Table 1. The optimal concentrations of OVA-TBBPA and biotinylated pAb-TBBPA were 0.212 mg mL−1 and 1
:
10 dilution.
Table 1 Optimal concentrations of biotinylated antibody and OVA-TBBPA
Dilutions of biotinylated antibody |
TBBPA-OVA concentration (mg mL−1) |
0.424 |
0.212 |
0.106 |
0.053 |
10 |
13.88 |
12.60 |
13.32 |
13.61 |
20 |
13.69 |
13.76 |
13.8 |
14.55 |
40 |
14.12 |
14.24 |
14.47 |
14.69 |
80 |
15.19 |
14.97 |
14.82 |
15.15 |
The sensitivity of this proposed rt-iPCR will be affected by any nonspecific binding of assay components, such as biotinylated pAbs, streptavidin, biotinylated DNA, etc. These types of nonspecific binding could be effectively reduced by blocking the surface of PCR tubes after the absorption of the coating antigen. Therefore, different blocking reagents, containing OVA, PEG 20000, gelatin, and skim milk powder, were tested for their blocking capacity. As a result, the blocking reagent of 3% OVA in PBS exhibited the lowest background value, which was selected as the blocking agent in the subsequent experiments. Besides, concentrations of avidin and biotinylated template DNA were optimized, and the results are shown in Tables 2 and 3. All of these experiments were conducted without adding any analyte during immunoassay procedures. When concentrations of avidin and biotinylated DNA were relatively low, the Ct values decreased with increasing avidin or biotinylated DNA concentration. However, the Ct value will not decrease continually, because it was restricted by the amount of coating antigen. Therefore, in the subsequent experiments, 10 μg mL−1 of avidin and 1 μg mL−1 of biotinylated DNA were selected.
Table 2 Effects of different concentrations of avidin on the indirect competition rt-iPCR
Avidin (μg mL−1) |
1 |
2.5 |
5 |
7.5 |
10 |
12 |
15 |
C
t value |
14.53 |
13.91 |
13.54 |
13.14 |
12.59 |
12.38 |
12.36 |
Table 3 Effects of different concentrations of biotinylated DNA on the indirect competition rt-iPCR
Biotinylated DNA (μg mL−1) |
0.01 |
0.05 |
0.1 |
0.5 |
1 |
1.5 |
C
t value |
13.54 |
13.27 |
13.03 |
12.86 |
12.62 |
12.65 |
Under the optimized conditions, a series of TBBPA standard solutions (1 pg L−1 to 100 ng L−1) was used in addition to build up standard curves. When TBBPA standard solutions and biotinylated pAb-TBBPA were added into the PCR tubes, the coated OVA-TBBPA and the free TBBPA analyte competitively combined with the antibody. If the concentration of the free TBBPA increased, the amount of biotinylated pAb-TBBPA, which would combine with the coated antigen, decreased. And then, the quantity of avidin and biotinylated DNA, fixed on the tube wall, would decrease correspondingly. Later, during the PCR amplification process, the amount of template DNA released was less, it will need more cycle numbers to reach the fluorescence threshold, and the Ct value was higher. So, in this proposed rt-iPCR assay, the concentration of TBBPA could be indirectly quantified by the Ct values. The amplification curves are displayed in Fig. 3. The fluorescent threshold was automatically set up in the real-time iPCR analyzer, which was generally determined as 10 times the standard deviation of the fluorescence signal from 3–15 cycles. The threshold cycle (Ct) was a fractional cycle number at which the amplification curve crossed the fluorescent threshold. Along with the standard solutions, a negative control (NC), containing all assay compounds except template DNA, was included.
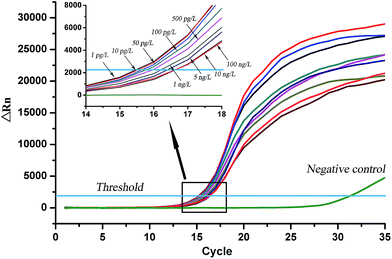 |
| Fig. 3 Amplification curves of a series of TBBPA standard solutions ranging from 1 pg L−1 to 100 ng L−1, as well as negative control. Negative control, containing all assay compounds except template DNA, did not cross the threshold until after 30 cycles. | |
The calibration curve of rt-iPCR is presented in Fig. 4. The linear working range was 10 pg L−1 to 10 ng L−1, with the linear regression equation Ct = 0.346
log
C + 16.16, R2 = 0.956. The detection limit (LOD) was 2 pg L−1 (3.3σ/S, where σ is the standard deviation of a blank sample, and S is the slope of the calibration curve).
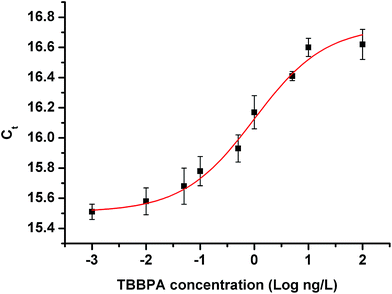 |
| Fig. 4 rt-iPCR standard curves and linear range of quantification from 10 pg L−1 to 10 ng L−1 of TBBPA. The limit of detection was determined to be 2 pg L−1. | |
In order to verify the repeatability of the proposed immunoassay, the intra- and inter-assays were conducted. The intra-assay repeatability was calculated after performing over 10 replicates, and the inter-assay repeatability was estimated over several weeks. The CV results of intra-assay and inter-assay are exhibited in Table 4. The intra-assay CVs were 1.9–5.0% and the inter-assay CVs were 5.3–10.2%. Although the CV values are relatively high, it is still acceptable for the rt-iPCR, because trace analysis is prone to higher variation when the concentration of the analyte is very low.
Table 4 The variability of intra-assay and inter-assay tests
Object |
TBBPA concentration (ng L−1) |
0.01 |
0.1 |
0.5 |
1 |
10 |
Intra-assay |
C
t (mean ± SD) |
15.58 ± 0.72 |
15.78 ± 0.61 |
15.93 ± 0.80 |
16.17 ± 0.32 |
16.6 ± 0.41 |
CV (%) |
4.6 |
3.9 |
5.0 |
1.9 |
2.5 |
Inter-assay |
C
t (mean ± SD) |
15.4 ± 0.97 |
15.80 ± 1.3 |
15.97 ± 0.91 |
16.1 ± 0.86 |
16.62 ± 1.7 |
CV (%) |
6.3 |
8.2 |
5.7 |
5.3 |
10.2 |
3.2. Specificity of rt-iPCR
Cross-reaction (CR, %) is an important factor in immunoassays, and is generally used to estimate the specificity of antibodies. The CR values were calculated according to eqn (1). |  | (1) |
Some structural analogues, 3,4-dichlorobiphenyl (PCB12), 3,4,4′-trichlorobiphenyl (PCB37), 3,3′,4,4′-tetrachlorobiphenyl (PCB77), 4,4′-isopropylidenebis(2-(2,6-dibromophenoxy)ethanol), and bisphenol A (BPA), were also tested. The chemical structures of the analogs and the CR results are shown in Table 5. All of these CR values were lower than 15%, which means that the proposed rt-iPCR assay was specific.
Table 5 The cross-reactivity of anti-TBBPA antibody with structural analogues
Analogues |
Structure |
C
t
|
Cross-reactivity (%) |
The Ct value of blank samples was 12.60.
|
TBBPA |
|
16.17 |
100 |
PCB12 |
|
12.65 |
1.6 |
PCB37 |
|
12.77 |
4.8 |
PCB77 |
|
12.90 |
8.7 |
4,4′-Isopropylidenebis(2-(2,6-dibromophenoxy)ethanol) |
|
13.01 |
11.5 |
BPA |
|
13.13 |
14.8 |
3.3 Determination of TBBPA in seafloor sediment samples and recovery tests
This proposed rt-iPCR immunoassay was applied to detect TBBPA residues in seabed sediment samples. According to the standard curves for the connection between the Ct value and the concentration, the mean quantity of each sample was obtained. All of these samples were tested six times, and the mean results are given in Table 6. Meanwhile, in order to evaluate the precision of rt-iPCR, these samples were also tested by the HPLC method, the results of which are also given in Table 6.
Table 6 Concentration of TBBPA in seabed sediment samples determined by rt-iPCR and HPLC
Sample |
rt-iPCR results (ng g−1, dw) ± SD; n = 6 |
HPLC results (ng g−1, dw) ± SD; n = 6 |
#1 |
161.1 ± 0.13 |
157.4 ± 0.090 |
#2 |
126.4 ± 0.051 |
120.9 ± 0.027 |
#3 |
186.7 ± 0.093 |
189.2 ± 0.058 |
#4 |
138.7 ± 0.036 |
137.4 ± 0.19 |
#5 |
176.1 ± 0.042 |
176.7 ± 0.084 |
#6 |
146.2 ± 0.17 |
151.6 ± 0.23 |
#7 |
21.9 ± 0.11 |
24.2 ± 0.076 |
#8 |
9.3 ± 0.089 |
<LOD |
#9 |
76.8 ± 0.045 |
81.5 ± 0.10 |
#10 |
26.5 ± 0.16 |
23.9 ± 0.025 |
Recovery studies are generally used to assess the analytical performance of immunoassay. So, untreated seabed sediment samples were previously fortified with a certain amount of target standard to reach the required concentrations and left at room temperature for 3 h to allow solvent evaporation. All of the fortified samples were tested six times using rt-iPCR. The results are exhibited in Table 7. Average recovery rates and CVs of rt-iPCR were 89.8% to 113.6% and 6.7% to 12.9%, respectively.
Table 7 Recoveries of TBBPA from spiking seabed sediment samples tested by rt-iPCR and HPLC methods
Sample |
Sample concentration (ng g−1, dw) |
Spiked level (ng g−1) |
Tested concentration (ng g−1) |
Recovery (%) |
CV (%, n = 6) |
#1 |
161.1 |
80 |
247.1 |
107.5 |
11.4 |
150 |
309.3 |
98.8 |
8.5 |
200 |
343.5 |
91.2 |
9.1 |
#2 |
126.4 |
50 |
183.2 |
113.6 |
8.2 |
100 |
228.5 |
102.1 |
10.5 |
200 |
343.8 |
108.7 |
8.9 |
#3 |
186.7 |
100 |
284.3 |
97.6 |
8.2 |
200 |
385.5 |
99.4 |
9.9 |
300 |
460.9 |
91.4 |
12.9 |
#4 |
138.7 |
50 |
183.6 |
89.8 |
6.7 |
100 |
247.8 |
109.1 |
10.2 |
200 |
334.7 |
98.0 |
7.3 |
4. Conclusions
An ultra-sensitive real-time immuno-PCR assay was constructed in this study. Greatly enhanced detection sensitivity of this proposed rt-iPCR is obtained for amplification of a 103-base-pair DNA molecule, which is coupled to the biotinylated anti-TBBPA pAbs. The detection limit of this method is 2 pg L−1, which is, as we know, the most sensitive LOD reported to date for the determination of TBBPA. The linear range was from 10 pg L−1 to 10 ng L−1, with negligible cross-reactivity to structural TBBPA analogs. This rt-iPCR was applied to test TBBPA residues in a series of seabed sediment samples and exhibited acceptable recovery rates and coefficients of variation. We believe that rt-iPCR offers the opportunity to be the most sensitive method for quantitative analysis of TBBPA. And this method will be a potential tool for detecting additional environmental contaminants with their specific antibodies.
Compliance with ethics requirements
In order to comply with the ethical requirements, all the authors of this article declare that they have no conflict of interest. The laboratory animals cared or used in the experiment followed the institutional and national guidelines. And this article does not contain any studies with human.
Acknowledgements
This study was supported by the National Natural Science Foundation of China (Project no. 21177082), the Science and Technology Commission of Shanghai Municipality in China (Key Project for Fundamental Research no. 09JC1407600), and the Science and Technology Commission of Shanghai Municipality in China (Key Project for Science and Technology Research no. 09231202805).
References
- Y. Q. Jiang, Current situation and development of bromine retardant in worldwide, Chin. J. Flame Retardant Mater. Technol, 2007, 2, 1–7 Search PubMed.
- M. S. E. Makinen, M. R. A. Makinen and J. T. B. Koistinen,
et al, Respiratory and dermal exposure to organophosphorus flame retardants and tetrabromobisphenol A at five work environments, Environ. Sci. Technol., 2009, 43, 941–947 CrossRef CAS.
- H. Takigami, G. Suzuki and Y. Hirai,
et al, Brominated flame retardants and other polyhalogenated compounds in indoor air and dust from two houses in Japan, Chemosphere, 2009, 76, 270–277 CrossRef CAS PubMed.
- R. S. Zhao, X. Wang and J. P. Yuan, Highly sensitive determination of tetrabromobisphenol A and bisphenol A in environmental water samples by solid-phase extraction and liquid chromatography-tandem mass spectrometry, J. Sep. Sci., 2010, 33, 1652–1657 CrossRef CAS PubMed.
- C. Sánchez-Brunete, E. Miguel and J. L. Tadeo, Determination of tetrabromobisphenol-A, tetrachlorobisphenol-A and bisphenol-A in soil by ultrasonic assisted extraction and gas chromatography-mass spectrometry, J. Chromatogr. A, 2009, 1216, 5497–5503 CrossRef PubMed.
- K. Jakobsson, K. Thuresson and L. Rylander,
et al, Exposure to polybrominated diphenyl ethers and tetrabromobisphenol A among computer technicians, Chemosphere, 2002, 46, 709–716 CrossRef CAS.
- C. Ronan, P. A. Jean and M. Philippe, New multiresidue analytical method dedicated to trace level measurement of brominated flame retardants in human biological matrices, J Chromatogr. A, 2005, 1100, 144–152 CrossRef PubMed.
- U. Berger, D. Herzke and T. M. Sandanger, Two trace analytical methods for determination of hydroxylated PCBs and other halogenated phenolic compounds in eggs from Norwegian birds of prey, Anal. Chem., 2004, 76, 441–452 CrossRef CAS PubMed.
- S. Morris, C. R. Allchin and B. N. Zegers,
et al, Distribution and fate of HBCD and TBBPA brominated flame retardants in North Sea Estuaries and aquatic food webs, Environ. Sci. Technol., 2004, 38, 5497–5504 CrossRef CAS.
-
IPCS, Environmental Health Criteria 172: Tetrabromobisphenol A and derivatives, World Health Organization, International Programme on Chemical Safety, Geneva, 1995, p. 139 Search PubMed.
- J. B. Fini, A. Riu and L. Debrauwer,
et al, Parallel biotransformation of tetrabromobisphenol A in Xenopus laevis and mammals: Xenopus as a model for endocrine perturbation studies, Toxicol. Sci., 2012, 125, 359–367 CrossRef CAS PubMed.
- A. Covaci, S. Voorspoels and M. A. Abdallah,
et al, Analytical and environmental aspects of the flame retardant tetrabromobisphenol-A and its derivatives, J. Chromatogr. A, 2009, 1216, 346–363 CrossRef CAS PubMed.
- Z. Xie, R. Ebinghaus and R. Lohmann,
et al, Trace determination of the flame retardant tetrabromobisphenol A in the atmosphere by gas chromatography-mass spectrometry, Anal. Chim. Acta, 2007, 584, 333–342 CrossRef CAS PubMed.
- X. L. Zhang, X. J. Luo and S. J. Chen,
et al., Spatial distribution and vertical profile of polybrominated diphenyl ethers, tetrabromobisphenol A, and decabromodiphenylethane in river sediment from an industrialized region of South China, Environ. Pollut., 2009, 157, 1917–1923 CrossRef CAS PubMed.
- Z. X. Shi, Y. N. Wu, J. G. Li, Y. F. Zhao and J. F. Feng, Dietary exposure assessment of Chinese adults and nursing infants to tetrabromobisphenol-A and hexabromocyclododecanes: occurrence measurements in foods and human milk, Environ. Sci. Technol., 2009, 43, 4314–4319 CrossRef CAS.
- P. Guerra, E. Eliarrat and D. Barceló, Simultaneous determination of hexabromocyclododecan, tetrabromobisphenol A, and related compounds in sewage sludge and sediment samples from Ebro River basin (Spain), Anal. Bioanal. Chem., 2010, 397, 2817–2824 CrossRef CAS PubMed.
- T. Sano, C. L. Smith and C. R. Cantor, Immuno-PCR: very sensitive antigen detection by means of specific antibody–DNA conjugates, Science, 1992, 258, 120–122 CAS.
- A. Schaefer, Real-time monitors wastewater treatment, Environ. Sci. Technol., 2003, 37, 51A CrossRef CAS.
- R. D. Joerger, T. M. Truby, E. R. Hendrickson, R. M. Young and R. C. Ebersole, Analyte detection with DNA-labeled antibodies and polymerase chain reaction, Clin. Chem., 1995, 41, 1371–1377 CAS.
- M. Komatsu, D. Kobayashi, K. Saito, D. Furuya, A. Yagihashi, H. Araake, N. Tsuji, S. Sakamaki, Y. Niitsu and N. Watanabe, Tumor Necrosis Factor-α in serum of Patients with Inflammatory Bowel Disease as Measured by a Highly Sensitive Immuno-PCR, Clin. Chem., 2001, 47, 1297–1301 CAS.
- J. Ren, Z. Chen, S. J. Juan, X. Y. Yong, B. R. Pan and D. M. Fan, Detection of circulating gastric carcinoma-associated antigen MG7-Ag in human sera using an established single determinant immuno-polymerase chain reaction technique, Cancer, 2000, 88, 280–285 CrossRef CAS.
- Z. Zhang, R. F. Irie, D. D. Chi and D. S. Hoon, Cellular immune-PCR. Detection of carbohydrate tumor marker, Am. J. Pathol., 1998, 152, 1427–1432 CAS.
- H. Y. Chao, Y. C. Wang, S. S. Tang and H. W. Liu, A highly sensitive immune-polymerase chain reaction assay for Clostridium botulinum neurotoxin type A, Toxicon, 2004, 43, 27–34 CrossRef CAS PubMed.
- B. Janet, Applications of real-time immune-polymerase chain reaction (rt-IPCR) for the rapid diagnoses of viral antigens and pathologic proteins, Mol. Aspects Med., 2006, 27, 224–253 CrossRef PubMed.
- D. Bu, H. S. Zhuang, X. C. Zhou and G. X. Yang, Biotin–streptavidin enzyme-linked immunosorbent assay for detecting Tetrabromobisphenol A in electronic waste, Talanta, 2014, 120, 40–46 CrossRef CAS PubMed.
- Y. Y. Yu, Q. E. Wang and H. S. Zhuang, Study on the New Fluorescence Immunoassays for the 2,4,6-Trichlorophenol in Environmental Water, Anal. Lett., 2006, 39, 937–946 CrossRef CAS.
- W. Zhang, M. Bielaszewska, M. Pulz, K. Becker, A. W. Friedrich, H. Karch and T. Kuczius, New immuno-PCR assay for detection of low concentrations of shiga toxin 2 and its variants, J. Clin. Microbiol., 2008, 46, 1292–1297 CrossRef CAS PubMed.
- R. D. Joerger, T. M. Truby, E. R. Hendrickson, R. M. Young and R. C. Ebersole, Analyte detection with DNA-labeled antibodies and polymerase chain reaction, Clin. Chem., 1995, 41, 1371–1377 CAS.
|
This journal is © The Royal Society of Chemistry 2015 |
Click here to see how this site uses Cookies. View our privacy policy here.