DOI:
10.1039/C5AN00589B
(Tutorial Review)
Analyst, 2015,
140, 6467-6472
Key steps towards the oriented immobilization of antibodies using boronic acids
Received
25th March 2015
, Accepted 8th August 2015
First published on 10th August 2015
Abstract
Oriented immobilization of antibodies using boronic acids shows a strong potential for improving immunoassay performance but is not yet widely used, possibly because of the difficulties encountered in its implementation. How to choose the boronic acid structure and how should it be attached to the surface? How to choose an antibody that will bind to the boronic acid? Under which conditions should the binding take place for an effective oriented antibody immobilization? How to make sure that the antibody stays on the surface? This tutorial review provides answers to these questions through analysis of the literature and personal suggestions, and thereby intends to facilitate the development of this promising antibody immobilization strategy.
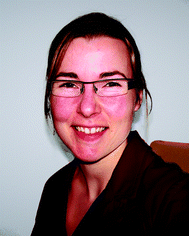 Florine Duval | Florine Duval received an MSc in Chemical Engineering and an MSc in Biomolecular Chemistry at Ecole Nationale Supérieure de Chimie de Montpellier in 2009. She is currently a PhD candidate under the supervision of Dr Teris A. van Beek and Prof. H. Zuilhof, developing novel applications of boronic acid-diol complexation. |
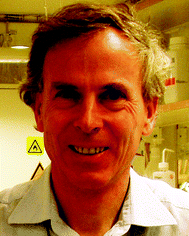 Teris A. van Beek | Teris A. van Beek performs research and teaches analytical chemistry at the Laboratory of Organic Chemistry of Wageningen University, Wageningen, The Netherlands since 1985. As such he has worked on the isolation, purification, identification, analysis and sensing of bioactive or economically relevant metabolites from plants, microbes, animals or humans. |
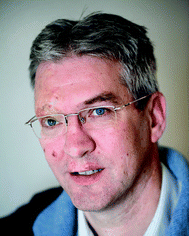 Han Zuilhof | Han Zuilhof is Chair of Organic Chemistry at Wageningen University, the Netherlands, and Distinguished Adjunct Professor of Chemical Engineering at King Abdulaziz University, Jeddah, Saudi Arabia. His interest in biospecific, so-called ‘romantic’ surfaces prompted his focus on the oriented immobilization of, e.g., antibodies on surfaces. |
1 Introduction
Antibody (Ab) immobilization is an important step in the preparation of devices for the detection of various analytes (= antigens) such as proteins, bacteria, viruses, drugs and toxins. Antibodies (Abs) are proteins, and protein immobilization strategies, which have been reviewed in detail,1,2 can be divided into two categories: random and oriented (or site-specific). Oriented immobilization is especially important for Abs in immunosensing applications, as their binding sites need to be kept available for antigen binding. Using oriented immobilization, signal enhancement factors of over 200 have been obtained,3 showing the significance of Ab orientation. Strategies for oriented Ab immobilization, which have recently been reviewed,4,5 aim at attaching the Ab to the surface via its Fc region (see the Ab structure in Fig. 1), far away from the binding sites. Abs are N-glycosylated (i.e., carbohydrate chains are attached to the amide nitrogen of Asn residues of Abs and thus defined as N-glycans) in their Fc regions, thus a proper orientation of the antibody can be achieved by immobilization via its N-glycans. This has mainly been done in two ways, both taking advantage of available vicinal diols in the N-glycans: (1) by oxidation of diols to aldehydes, which then react with nucleophiles attached to the surface, and (2) by reaction of diols with boronic acids attached to the surface. The present review focuses on the second approach.
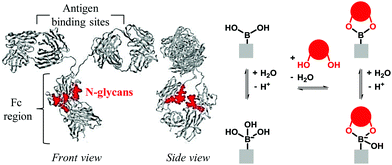 |
| Fig. 1 Principle of oriented antibody immobilization using boronic acids. Left: crystal structure of immunoglobulin with highlighted N-glycans (3D model from NCBI, recolored); right: scheme of the boronic acid–diol complexation.19 | |
Boronic acids react with 1,2-diols to form boronate esters (Fig. 1). This reaction has been used for many applications, including the separation and immobilization of glycoproteins (including antibodies).6 Oriented Ab immobilization using boronic acids (through the same reaction) was reported for the first time in 2009 by Lin et al.,7 and has appeared in another eleven papers since then.8–18 An overview of these articles is given in Table 1. This immobilization method appears very attractive, as it presents several advantages over more common ones: it leaves the binding sites available for antigen binding (oriented vs. random immobilization), it can be done in one step without prior antibody modification (vs. oxidation with periodate, use of antibody fragments or enzymatic modification of the N-glycans), and it is relatively economical (vs. Fc-chain binding proteins such as protein A/G). Moreover, several comparative studies (in Ab-BA 1, 2, 7 and 11) suggested that the highest antigen detection sensitivity was achieved when Abs were immobilized using boronic acids.
Table 1 Overview of the publications that report antibody immobilization via boronic acids (BAs). The “paper codes” are used in this review to quickly refer to these papers
Paper code |
Main feature |
Ref. |
Ab-BA 1 |
First Ab immobilization via BA |
7
|
Ab-BA 2 |
Novel immunosensing application |
8
|
Ab-BA 3 |
Novel immunosensing application |
9
|
Ab-BA 4 |
Novel immunosensing application |
10
|
Ab-BA 5 |
Novel immunosensing application |
11
|
Ab-BA 6 |
BA on zwitterionic polymer brushes |
12
|
Ab-BA 7 |
Probing of the available binding sites |
13
|
Ab-BA 8 |
Direct assessment of Ab orientation |
14
|
Ab-BA 9 |
Microarray of 71 Abs |
15
|
Ab-BA 10 |
First irreversible Ab immob. via BA |
16
|
Ab-BA 11 |
Novel immunosensing application |
17
|
Ab-BA 12 |
Novel immunosensing application |
18
|
The number of papers reporting this immobilization strategy, however, remains limited (12 papers, four of them having been reviewed by Wang et al.6). So the question arises: where does its lack of popularity come from? We believe that many researchers may have tested Ab immobilization via boronic acids, that likely problems were encountered in most cases, and that therefore few successful studies were published. Although promising, this immobilization strategy is not as simple as it seems. In this tutorial review, the reader is guided through several points that need to be considered to allow the development of the full potential of this still very appealing approach.
2 Step-wise guide
2.1 Preparation of boronic acid-functionalized surface
The first step towards a successful Ab immobilization via boronic acids is a surface modification with the right molecules.
Boronic acid structure.
The interaction between a boronic acid and a diol strongly depends on the structure of both partners.19 This interaction, hence the Ab immobilization efficiency, may be enhanced by a boronic acid with a low pKa value, which can be obtained by electron-withdrawing groups or intramolecular interactions (e.g., in Wulff-type boronic acids and benzoboroxoles).19,20 To achieve a correct Ab orientation, secondary interactions between the boronic acid and Ab should be minimized: a hydrophilic boronic acid would help to minimize hydrophobic interactions, and ionic interactions may be prevented by using a boronic acid that is not negatively charged at high pH (e.g., Wulff-type boronic acid or benzoboroxole).20 3-Aminophenylboronic acid was used in ten out of the twelve Ab-BA papers, but this choice was never justified and, in view of the above, may provide room for improvement.
Spacer length and structure.
The N-glycans are nested between the Ab Fc chains, and relatively far from the C-terminus/“bottom” (Fig. 1). If the boronic acid is directly attached to the surface without a spacer or with a too short one, then the Ab may not be immobilized or it may be forced to “lie” on the surface, which is not optimal for antigen binding. In contrast, if a long and flexible spacer separates the boronic acid from the surface, then the Ab has more freedom and it can “stand”. Thus we expect that the antigen binding capacity was relatively high when Abs were immobilized on zwitterionic polymer brushes (Ab-BA 6, Fig. 2) or poly(ethylene glycol) (PEG) chains (Ab-BA 10) that had been functionalized with boronic acids.
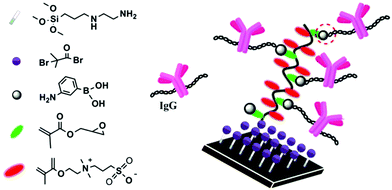 |
| Fig. 2 Antibody immobilization via boronic acids on zwitterionic polymer brushes.12 Adapted with permission from ref. 12. Copyright 2015 American Chemical Society. | |
Antifouling surface.
In Ab-BA 1, 6, 8, 10 and 11, which report the reaction of an amine-containing boronic acid molecule with an active ester (e.g., N-hydroxysuccinimide, NHS) or epoxide on the surface, the active esters or epoxides remaining after the reaction were deactivated with ethanolamine. This is required to prevent Ab nucleophilic groups (such as the –NH2 of lysine residues) from reacting with surface-bound esters or epoxides, hence yielding a random orientation again.
Furthermore, an antifouling coating helps to minimize other types of unwanted (e.g., electrostatic or hydrophobic) interactions between Ab and the surface. PEG is widely used for its antifouling properties, and zwitterionic polymer brushes prevent the nonspecific adsorption of biomolecules even more effectively.21,22 Thus, it may be a good idea to prepare boronic acid-containing zwitterionic polymer brushes, as was done in Ab-BA 6 (Fig. 2). Alternatively, commercially available substrates with antifouling coatings decorated with reactive groups such as NHS or epoxide may be modified with a boronic acid that contains an appropriate reactive group (such as 3-aminophenylboronic acid). This has for example been done in Ab-BA 10, in which commercial PEG–NHS slides were used. One has to keep in mind, however, that some antifouling polymers bind with boronic acid groups, possibly resulting in a decreased availability of the boronic acid groups for subsequent Ab binding. This problem may be encountered with dextran, which has been used as a boronic acid blocking agent in Ab-BA 1, 6 and 10, and with polymers of unknown structure, based on our own experience with proprietary coatings of some commercial antifouling slides.
Characterization of the boronic acid-functionalized surface.
In order to pinpoint causes of possibly unsuccessful Ab immobilization, it is useful to know whether the surface actually is modified with boronic acids. In Ab-BA 3, 4, 8 and 12, changes in surface properties before and after modification with 3-aminophenylboronic acid suggested that a chemical change indeed occurred, but did not prove the boronic acid integrity. In Ab-BA 2 and 6, however, X-ray photoelectron spectroscopy (XPS) revealed the presence of boron, and in Ab-BA 6, narrow-scan XPS data more accurately indicated the presence of boronic acids on the surface. XPS, though, is not available to every researcher and we would like to suggest a much simpler and more widely applicable method. Alizarin and its more water-soluble derivative alizarin red S (ARS) strongly bind with boronic acids to form fluorescent complexes.23 Outside the field of Ab immobilization, boronic acid-functionalized surfaces have been incubated with ARS, and characterization by fluorescence imaging then allowed for an even quantitative indicator of the presence of surface-bound boronic acid moieties.24–28
2.2 Choice of antibody with suitable glycosylation
Many different Abs were used in the Ab-BA papers. Few of them were fully described and the rationale behind their selection was never explained. Considering the intrinsic properties of an Ab, a key factor for its successful immobilization is the composition of the N-glycans at its Fc chain. These N-glycans must present diols that have a suitable conformation for binding with boronic acids. Many monosaccharides bind with boronic acids, but when they are attached to each other, relatively weak interactions between boronic acids and the formed glycans are enabled. Sialic acid, however, has a side chain with a free and flexible glycol function that may bind more strongly to boronic acids.19 Based on this, the presence of sialic acids in the Ab N-glycans is highly favorable, and possibly even required for a successful immobilization. The Ab N-glycans vary with the cell line, animal species, culture conditions and even from batch to batch, resulting in the presence or absence of sialic acids at the glycan chain ends.29
Analysis of Ab candidates for immobilization is therefore advisable. Ab glycosylation may be analyzed using mass spectrometric and chromatographic techniques,30 or more simply, Abs may be screened for sialic acids using the periodic acid-Schiff method31 (we used a commercial glycoprotein carbohydrate estimation kit). The affinity of Abs for boronic acids may also directly be assessed by boronate affinity chromatography using a commercially available boronate column,32,33 although the results will also depend on the properties of the column and the binding conditions (see section 2.3).
2.3 Antibody immobilization on the boronic acid-functionalized surface
Binding conditions.
Depending on the binding conditions, (glyco)proteins can bind to boronic acids not only via the boronic acid–diol complexation, but also via secondary interactions, such as hydrophobic, ionic20 and charge-transfer interactions.33 As these interactions would surely result in a partly random Ab immobilization, optimization of the binding conditions is essential. For example, the ionic strength should be low enough to minimize hydrophobic interactions and high enough to minimize ionic interactions.20 The pH should be low enough to preserve the integrity of the biomolecules and high enough to minimize charge transfer interactions between boronic acids on the surface and amines in the antibody.33 These effects are illustrated in the article by Azevedo et al., who studied the binding of pure human IgG, human recombinant insulin (non-glycosylated) and human serum albumin (HSA, poorly glycosylated) by boronate affinity chromatography under various conditions (Fig. 3).33 When PBS with pH 7 was used, almost all the IgG was bound, as well as a significant amount of HSA and insulin. PBS with pH ≈ 7 was used in Ab-BA 3, 4, 5, 9, 10, 11 and 12, in which no proof of the Ab orientation is given. Thus, it might be that some of the Abs were actually physically adsorbed in a random way on the boronic acid-functionalized surfaces. In contrast, Fig. 3 shows a very good selectivity for IgG when HEPES at pH 8.5 was used. HEPES was used in Ab-BA 8, although the pH was unfortunately not mentioned. This paper happens to be the only one in which the actual Ab orientation was studied (see below).
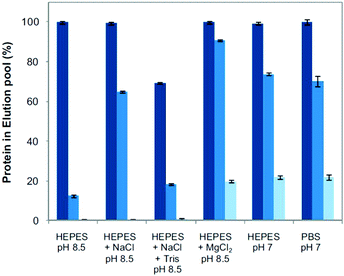 |
| Fig. 3 Binding of proteins to boronic acids as displayed by percentage of bound and then eluted IgG (dark), albumin (middle), and insulin (light) in different adsorption buffers: (i) 20 mM HEPES at pH 8.5; (ii) 20 mM HEPES, 150 mM NaCl at pH 8.5; (iii) 20 mM HEPES, 150 mM NaCl, 15 mM Tris at pH 8.5; (iv) 20 mM HEPES, 150 mM MgCl2 at pH 8.5; (v) 20 mM HEPES at pH 7; and (vi) 10 mM phosphate, 150 mM NaCl at pH 7. Reproduced with permission.33 | |
Reaction time.
Although the incubation in Ab-BA 1, 2, 4, 6, 7, 10, 11 and 12 was done for 12 h or more at 4 °C, it was shown in Ab-BA 8 that the Ab immobilization was complete within 20 min.
Blocking.
After Ab immobilization, the remaining surface binding sites (any sites that may attract unwanted proteins in subsequent experiments, e.g., by boronic acid–carbohydrate, hydrophobic or electrostatic interactions) were blocked with dextran (Ab-BA 1, 6, 10 and 11), bovine serum albumin (Ab-BA 3, 4, 5, 6, 8, 11 and 12), casein (Ab-BA 7), milk (Ab-BA 9) or left unblocked (Ab-BA 2). In Ab-BA 1, five different compounds (glucose, glycerol, dextran, ethylene glycol and ethanolamine) were tested for blocking. Dextran was selected as it resulted in the most effective suppression of non-specific binding, although we expect that it may expel the Ab from the surface upon binding with the boronic acid.
Characterization of the antibody-functionalized surface.
In order to pinpoint causes of possibly unsuccessful antigen binding, it is useful to figure out whether the Ab is bound to the surface in the expected way. Although Ab-BA 1, 4, 5, 6, 7 and 12 reported experiments that indicated that the Abs were successfully immobilized on the surfaces, the orientation of the Abs was directly assessed in Ab-BA 8 only: dual polarization interferometry showed upon binding of the Ab a thickness increase of 8.44 ± 0.86 nm and a mass increment of 3.33 ± 0.40 ng mm−2. These values indicated that the Abs were attached to the surface via their Fc chain (“end-on” conformation), based on Ab dimensions of 14.2 × 8.5 × 4.0 nm and a previously reported mass increment of 3.70 ng mm−2 for an end-on oriented Ab. More information about the techniques to study Ab orientation can be found in a previous review by our group.4
2.4 Stability
The reaction between a boronic acid and a diol to form a boronate ester is reversible, which may strongly disturb Ab immobilization. The diol content and pH of the solution both have a strong influence on the equilibrium.
Effect of competing diols on the stability of the immobilized antibody.
In Ab-BA 5 and 6, it was shown that sorbitol solutions were able to release the Ab from the surface. In Ab-BA 1, a western blot (Fig. S4 in Ab-BA 1) suggests a poor stability of the Ab-functionalized material in serum. In order to prevent the competition of soluble diols with the immobilized Ab, 3-aminophenylboronic acid was added to serum before incubation of the Ab-functionalized material with this serum. Subsequent antigen binding was apparently improved, but we are doubtful about the validity of this treatment: 3-aminophenylboronic acid may compete with the surface-bound boronic acid and expel the Ab from the surface, resulting in a loss of antigen binding capacity and consequently a wrong measurement of the antigen concentration in the sample.
If the antigen is a protein, then chances are high that it is glycosylated.34 Therefore, it may expel the Ab from the surface, resulting in erroneous measurements. Blocking antigen diols with 3-aminophenylboronic acid may not only cause the problem described in the previous paragraph, but also cause blocking of the antigen epitopes or nonspecific interactions between the antigen and the surface. However, if the antigen is big enough and the Ab layer is dense enough, then steric hindrance may prevent the antigen from accessing the boronic acid.35 If steric hindrance is not sufficient, then we recommend a synthetically involved but elegant solution: make the boronic acid-mediated immobilization irreversible (see below).
Effect of pH on the stability of the immobilized antibody.
Treatment with acidic solutions, as reported in Ab-BA 6 (pH 3) and 8 (glycine pH 2.5), resulted in dissociation of the Ab from the surface. In Ab-BA 1, a western blot (Fig. S2 in Ab-BA 1) suggests an increasing dissociation of the Ab from the surface when the pH was increased from 7 to 12. If the immunoassay conditions stay in a “safe” pH range and the sensor surface is not intended for reuse, then this should not cause a problem. If regeneration of the sensor surface with an acidic or basic solution is required, then one must keep in mind that not only the antigen, but also part or all of the Ab will be washed away, depending on the regeneration conditions.
Making the binding irreversible.
Reversibility of the binding between boronic acid and diol remains an obstacle of Ab immobilization that relies only on this binding. However, Adak et al. reported a strategy to make this immobilization irreversible (Fig. 4).16 First, a molecule that contains a primary amine, a boronic acid and a diazirine was synthesized, and attached on a surface via its primary amine. Second, the Ab was immobilized via the binding between N-glycan in the Fc chain and boronic acid on the surface. Third, the surface was irradiated with UV light: the diazirine group, inactive until then, was in this way converted into a carbene. This highly reactive carbene formed covalent bonds in its vicinity and hence in the Ab Fc chain, resulting in oriented and irreversible Ab immobilization, with a concomitantly increased sensitivity for antigen detection.
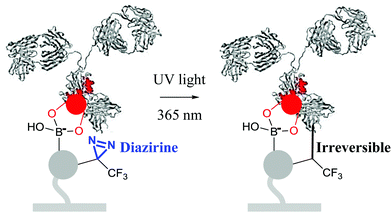 |
| Fig. 4 Strategy for oriented and irreversible antibody immobilization.16 | |
3 Conclusion and outlook
Ab immobilization using boronic acids requires substantial preliminary work: preparing a boronic acid-containing antifouling surface (most likely via several chemical steps), finding an Ab with suitable N-glycans, finding binding conditions that result in effective and oriented Ab immobilization, and making sure that the immobilized Ab is not expelled from the surface by competing diols or harsh regeneration conditions.
However, once the basic requirements are met and initial optimizations are done, Ab immobilization via boronic acids may result in a much higher sensitivity for antigen detection than what can be achieved by random immobilization, and an improvement of cost and simplicity compared to other strategies for oriented Ab immobilization.
Future research in this field may give precious information about trends in the glycosylation of Abs and their affinity for boronic acids, and explore the effect of boronic acid structure, surface architecture and binding conditions on Ab immobilization and orientation. This could lead to further strategies for oriented and irreversible Ab immobilization, making Ab immobilization via boronic acids a well-established method within the fabrication of high-performance biosensors. Moreover, further knowledge about the interactions between boronic acids and antibodies may lead to improvements in other related fields, such as antibody, or more generally, glycoprotein purification and site-specific antibody labelling.
Notes and references
- F. Rusmini, Z. Zhong and J. Feijen, Biomacromolecules, 2007, 8, 1775–1789 CrossRef CAS PubMed.
- P. Jonkheijm, D. Weinrich, H. Schroder, C. M. Niemeyer and H. Waldmann, Angew. Chem., Int. Ed., 2008, 47, 9618–9647 CrossRef CAS PubMed.
- A. K. Trilling, M. M. Harmsen, V. J. B. Ruigrok, H. Zuilhof and J. Beekwilder, Biosens. Bioelectron., 2013, 40, 219–226 CrossRef CAS PubMed.
- A. K. Trilling, J. Beekwilder and H. Zuilhof, Analyst, 2013, 138, 1619–1627 RSC.
- A. Makaraviciute and A. Ramanaviciene, Biosens. Bioelectron., 2013, 50, 460–471 CrossRef CAS PubMed.
- X. Wang, N. Xia and L. Liu, Int. J. Mol. Sci., 2013, 14, 20890–20912 CrossRef PubMed.
- P. C. Lin, S. H. Chen, K. Y. Wang, M. L. Chen, A. K. Adak, J. R. R. Hwu, Y. J. Chen and C. C. Lin, Anal. Chem., 2009, 81, 8774–8782 CrossRef CAS PubMed.
- J. A. A. Ho, W. L. Hsu, W. C. Liao, J. K. Chiu, M. L. Chen, H. C. Chang and C. C. Li, Biosens. Bioelectron., 2010, 26, 1021–1027 CrossRef CAS PubMed.
- M. Moreno-Guzmán, I. Ojeda, R. Villalonga, A. González-Cortés, P. Yáñez-Sedeño and J. M. Pingarrón, Biosens. Bioelectron., 2012, 35, 82–86 CrossRef PubMed.
- M. Moreno-Guzmán, A. González-Cortés, P. Yáñez-Sedeño and J. M. Pingarrón, Electroanalysis, 2012, 24, 1100–1108 CrossRef PubMed.
- Z. Wang, K. Shang, J. Dong, Z. Cheng and S. Ai, Microchim. Acta, 2012, 179, 227–234 CrossRef CAS.
- L. Song, J. Zhao, S. Luan, J. Ma, J. Liu, X. Xu and J. Yin, ACS Appl. Mater. Interfaces, 2013, 5, 13207–13215 CAS.
- W. C. Liao and J. A. Annie Ho, Biosens. Bioelectron., 2014, 55, 32–38 CrossRef CAS PubMed.
- H. Y. Song, J. Hobley, X. Su and X. Zhou, Plasmonics, 2014, 9, 851–858 CrossRef CAS.
- M. González-González, R. Bartolome, R. Jara-Acevedo, J. Casado-Vela, N. Dasilva, S. Matarraz, J. García, J. A. Alcazar, J. M. Sayagues, A. Orfao and M. Fuentes, Anal. Biochem., 2014, 450, 37–45 CrossRef PubMed.
- A. K. Adak, B.-Y. Li, L.-D. Huang, T.-W. Lin, T.-C. Chang, K. C. Hwang and C.-C. Lin, ACS Appl. Mater. Interfaces, 2014, 6, 10452–10460 CAS.
- L.-D. Huang, A. K. Adak, C.-C. Yu, W.-C. Hsiao, H.-J. Lin, M.-L. Chen and C.-C. Lin, Chem. – Eur. J., 2015, 21, 3956–3967 CrossRef CAS PubMed.
- S. A. Lim and M. U. Ahmed, Biosens. Bioelectron., 2015, 70, 48–53 CrossRef CAS PubMed.
- J. A. Peters, Coord. Chem. Rev., 2014, 268, 1–22 CrossRef CAS PubMed.
- H. Li and Z. Liu, TrAC, Trends Anal. Chem., 2012, 37, 148–161 CrossRef CAS PubMed.
- C. Rodriguez Emmenegger, E. Brynda, T. Riedel, Z. Sedlakova, M. Houska and A. B. Alles, Langmuir, 2009, 25, 6328–6333 CrossRef CAS PubMed.
- S. Joshi, P. Pellacani, T. A. van Beek, H. Zuilhof and M. W. F. Nielen, Sens. Actuators, B, 2015, 209, 505–514 CrossRef CAS PubMed.
- F. Duval, T. A. Van Beek and H. Zuilhof, Synlett, 2012, 1751–1754 CAS.
- D. Zhang, K. L. Thompson, R. Pelton and S. P. Armes, Langmuir, 2010, 26, 17237–17241 CrossRef CAS PubMed.
- A. E. Ivanov, A. Kumar, S. Nilsang, M. R. Aguilar, L. I. Mikhalovska, I. N. Savina, L. Nilsson, I. G. Scheblykin, M. V. Kuzimenkova and I. Y. Galaev, Colloids Surf., B, 2010, 75, 510–519 CrossRef CAS PubMed.
- P.-C. Chen, L.-S. Wan, B.-B. Ke and Z.-K. Xu, Langmuir, 2011, 27, 12597–12605 CrossRef CAS PubMed.
- R. Pelton, Y. Cui, D. Zhang, Y. Chen, K. L. Thompson, S. P. Armes and M. A. Brook, Langmuir, 2012, 29, 594–598 CrossRef PubMed.
- Y.-W. Lu, C.-W. Chien, P.-C. Lin, L.-D. Huang, C.-Y. Chen, S.-W. Wu, C.-L. Han, K.-H. Khoo, C.-C. Lin and Y.-J. Chen, Anal. Chem., 2013, 85, 8268–8276 CrossRef CAS PubMed.
- S. Raju, BioProcess Int., 2003, 1, 44–53 Search PubMed.
- C. Huhn, M. H. J. Selman, L. R. Ruhaak, A. M. Deelder and M. Wuhrer, Proteomics, 2009, 9, 882–913 CrossRef CAS PubMed.
- G. P. Roberts, Histochem. J., 1977, 9, 97–102 CrossRef CAS.
- B. Zhang, S. Mathewson and H. Chen, J. Chromatogr., A, 2009, 1216, 5676–5686 CrossRef CAS PubMed.
- A. M. Azevedo, A. G. Gomes, L. Borlido, I. F. S. Santos, D. M. F. Prazeres and M. R. Aires-Barros, J. Mol. Recognit., 2010, 23, 569–576 CrossRef CAS PubMed.
- G. A. Khoury, R. C. Baliban and C. A. Floudas, Sci. Rep., 2014, 1, 90 Search PubMed.
- S. Liu, X. Zhang, Y. Wu, Y. Tu and L. He, Clin. Chim. Acta, 2008, 395, 51–56 CrossRef CAS PubMed.
|
This journal is © The Royal Society of Chemistry 2015 |
Click here to see how this site uses Cookies. View our privacy policy here.