DOI:
10.1039/C5AN00112A
(Paper)
Analyst, 2015,
140, 6254-6259
Rapid detection of cocaine, benzoylecgonine and methylecgonine in fingerprints using surface mass spectrometry
Received
19th January 2015
, Accepted 1st May 2015
First published on 1st May 2015
Abstract
Latent fingerprints provide a potential route to the secure, high throughput and non-invasive detection of drugs of abuse. In this study we show for the first time that the excreted metabolites of drugs of abuse can be detected in fingerprints using ambient mass spectrometry. Fingerprints and oral fluid were taken from patients attending a drug and alcohol treatment service. Gas chromatography mass spectrometry (GC-MS) was used to test the oral fluid of patients for the presence of cocaine and benzoylecgonine. The corresponding fingerprints were analysed using Desorption Electrospray Ionization (DESI) which operates under ambient conditions and Ion Mobility Tandem Mass Spectrometry Matrix Assisted Laser Desorption Ionization (MALDI-IMS-MS/MS) and Secondary Ion Mass Spectrometry (SIMS). The detection of cocaine, benzoylecgonine (BZE) and methylecgonine (EME) in latent fingerprints using both DESI and MALDI showed good correlation with oral fluid testing. The sensitivity of SIMS was found to be insufficient for this application. These results provide exciting opportunities for the use of fingerprints as a new sampling medium for secure, non-invasive drug detection. The mass spectrometry techniques used here offer a high level of selectivity and consume only a small area of a single fingerprint, allowing repeat and high throughput analyses of a single sample.
Introduction
The drug testing industry is worth several billion dollars worldwide, and is routinely used by probation services, prisons, courts and other law enforcement agencies. Recently there has been a push towards workplace drug testing as well as new initiatives to test motorists for drug driving.1 Drug testing is usually carried out by taking a sample of blood or urine from a suspect and using either an antibody assay or chromatographic analysis to detect the relevant drug and its metabolites. However, these methods of sampling have limitations – blood testing can require trained staff, urine testing has associated privacy concerns and in both cases the samples must be treated as a biological hazard, which increases the complexity of sample handling in terms of storage and disposal. Oral fluid and sweat have been proposed as alternative non-invasive collection matrices. These matrices, as well as oral fluid and sweat, frequently require extraction steps from the collection devices or precipitation from the biological fluids prior to analysis and this contributes to the cost of analysis.2
In contrast, a latent fingerprint can be deposited quickly and transported easily. The identity of the donor is encapsulated within the fingerprint ridge detail, making the test impossible to falsify. It has recently been shown that drugs and their metabolites can be detected in latent fingerprints using antibody reagents.3 Whilst antibody reagents provide a rapid screening test, non-specific binding can lead to false positive results. Mass spectrometry techniques provide a higher level of specificity, providing confirmation of the identity of the substance detected.
Recent work by Goucher et al.4 has shown that liquid chromatography mass spectrometry (LC-MS) can be used to detect lorazepam, methadone and their metabolites in latent fingerprints. However, a positive detection was only achieved when ten fingerprints were used (and consumed) in combination, making it impractical for use in the field as this precludes repeat analysis. In contrast, recent developments in surface mass spectrometry allow in situ analyses of a small area (less than 100 × 100 μm) of a sample under atmospheric pressure conditions. This provides the potential for rapid sample throughput (often a few seconds per sample, compared with several hours for LC-MS when sample preparation is taken into account), a demonstration that the substance of abuse resides in the fingerprint and not the substrate and the opportunity to take repeat tests. These techniques have been applied previously to fingerprints that have been deliberately contaminated by drugs via contact5–7 but (to our knowledge) not to unambiguously confirm the presence of drug metabolites in the fingerprints of drug users. In order to confirm ingestion of a drug (as opposed to contact), detection of drug metabolites is essential. Rowell et al.8 used surface assisted laser desorption ionization (SALDI) under vacuum conditions to detect the major metabolite of methadone in natural fingerprints, and was unable to confirm its presence using tandem mass spectrometry.
Of the many surface mass spectrometry techniques available, matrix assisted laser desorption ionization (MALDI),9–11 desorption electrospray ionization (DESI)5 and secondary ion mass spectrometry (SIMS)12–14 have been previously shown to provide chemical images of endogenous and exogenous compounds in a latent fingerprint. In this context, the techniques offer complementary features, these being high sensitivity (MALDI), quantitative precision (SIMS) and high throughput (DESI). All three of the techniques can operate under atmospheric pressure conditions, allowing high throughput analysis. In this paper, we will show for the first time that cocaine and its metabolites benzoylecgonine (BZE) and methylecgonine (EME) can be detected by these surface mass spectrometry techniques in latent fingerprints, and in particular under atmospheric pressure conditions, offering an exciting new opportunity for drug testing.
Materials and methods
Cocaine, EME, acetone and acetonitrile (ACN) were obtained from Sigma Aldrich (St. Louis, USA). BZE was obtained either from Sigma Aldrich (St. Louis, USA) or from Sigma Aldrich (Poole, UK). Methanol was obtained either from Rathburn (Walkerburn, UK) or from Sigma Aldrich (Poole, UK). Trifluoroacetic acid (TFA), and α-cyano-4-hydroxycinnamic acid (α-CHCA) were purchased from Sigma Aldrich (Poole, UK). Double-side conductive carbon tape was obtained from TAAB (Aldermaston, UK).
Sample preparation
Latent fingerprints.
Latent fingerprints were obtained from five individuals who were attending a drug and alcohol treatment service to receive treatment for drug dependence. “Natural” fingerprints were deposited onto clean glass slides at a pressure between 400–1000 g and barcoded, before being shipped to the two analyzing laboratories. The fingerprints were then stored in a freezer at each lab at −80 °C. A corresponding oral fluid sample was collected from each patient using a Quantisal™ collection kit, Alere Toxicology, UK. The oral fluid samples were screened for cocaine and benzoylecgonine BZE using a standard drug screening procedure utilizing GC-MS/MS at LGC Forensics, Teddington, Middlesex. A favourable ethical opinion for sample collection and analysis was granted from the National Research Ethics Service. In line with the ethics protocol, the patients were not asked whether they had used drugs.
MALDI.
For MALDI analyses, the fingerprints were taken out of storage and a 0.5 μL BZE standard solution at a concentration of 100 μg mL−1 in 1
:
9 methanol
:
water mixed 1
:
1 with a solution of 5 mg mL−1 α-CHCA in 70
:
30 ACN: 0.5% TFA was then spotted onto an area of the glass slide away from the mark. Both the fingerprint and the standard on the glass slide were allowed to dry in a vacuum desiccator for 20 minutes prior to analysis. The entire fingermark was spotted at random points with the MALDI matrix before spectral acquisition. Fingerprints were analysed approximately 3 months after deposition.
DESI and SIMS.
Gels containing standards of cocaine, BZE and EME at concentrations of 5, 50, 500 and 5000 ng ml−1 were pipetted onto glass slides and smeared across the slides to create a uniform layer. The gels, which were a few mm in thickness, were made of synthetic gelatine (Sigma Aldrich, UK) and analysed using DESI and SIMS. No sample preparation was required for the fingerprint samples. Fingerprints were analysed approximately 1 month after deposition.
Instrumentation
MALDI ion mobility tandem mass spectrometry (MALDI-IMS-MS/MS) analysis was conducted using a Synapt™ G2 HDMS mass spectrometer (Waters Corporations, Manchester, UK) incorporating a Nd:YAG laser operating at 1 kHz. Spectra were acquired in positive and resolution (‘Res’) mode in the mass range between m/z 100–1000 with a laser power of 200 (a.u.). Transfer fragmentation was used, allowing for ions to be separated based on their mobility and then subjected to collision induced dissociation in the Transfer T-Wave™. This maintained that product ions retained the same ion drift time as their corresponding precursor ion. Collision energy was applied at 26 eV for the dissociation of BZE. Prior to analysis, calibration was performed in the specified mass range using Glu-1-fibrinopeptide. Data processing of all spectra was performed using MassLynx 4.1 (Waters Corporation, Manchester, UK).
DESI analyses were carried out at the National Physical Laboratory, Teddington using a DESI 2D Omni Spray Ion Source (Prosolia, Indianapolis, IN, USA) and an LTQ Orbitrap Velos mass spectrometer (Thermo Scientific, Bremen, Germany), optimised as outlined in ref. 15. Data were acquired using the Orbitrap mass analyser with the highest resolution setting of 100
000 (at m/z 400), except where stated when the linear ion trap analyser was used. The solvent composition was 90
:
10 MeOH
:
H2O supplied at a flow rate of 1.5 μL min−1 using a 5 kV bias. The spray was directed through a 50 micron capillary, giving a spot of approximately 300 × 300 μm on the sample.16 Approximately 1 mm2 of sample was consumed during the analysis. Spectra were collected in positive ion mode using both full scan mode and MS/MS mode.
SIMS analyses were carried out using an ION-TOF GmbH (Münster, Germany) TOF SIMS 5 instrument at the University of Surrey, UK. A 25 keV Bi3+ primary ion beam delivering 0.18 pA of current was employed. Images were acquired at 128 × 128 resolution in the MacroRaster mode of operation. Scans of 500 × 500 μm were acquired. Image data were acquired using 512 cycles per pixel point with 1 scan per pixel. A cycle time of 100 μs was employed. Mass calibration was performed by assigning peaks with a known mass using IonSpec software (version 4.1). Calibration was carried out using the following ions: CH3, Na, C2H3, CHO, C2H5, C3H5, C2H3O, C3H7, C2H5O, C4H5, C3H3O, C4H7, C3H5O, C4H9, C6H5, C7H7, and Si2C5H15O.
Results
MALDI IMS-MS/MS
The ion mobility function can provide superior separation of isobaric ions and sensitivity, as ions are separated, in addition to their m/z, also on the basis of a specific ‘drift time’ (DT) that is unique to that particular ion as it is linked to their collisional cross section.17 This allows for the reliable detection of biologically relevant concentrations. In this instance, MALDI-IMS-MS/MS has been employed to confirm the presence of BZE based on the matching of the product ions and their DT's in the fingerprint and in the BZE standard. MALDI IMS-MS/MS analysis of both the standard and the mark allowed for Drift Scope Plots (a graphical representation of the ion mobility separation) to be generated and select DT regions of interest containing both the parent and the corresponding product ions (Fig. 1A). Spectra from these acquisitions were interrogated for a product ion derived from the collision induced dissociation of BZE which directly corresponded to those observed in the standard (m/z 168.1033) (Fig. 1B). Drift time chromatograms could then be produced based on the DT of this product ion, showing that the BZE product ion in the standard and the mark directly coincided (Fig. 1C). These data prove, unequivocally, that it is possible to detect excreted BZE contained within the fingerprint residue with corresponding oral fluid above the 16 ng ml−1 cut-off determined by the service provider's GC-MS protocol18 (although it is unknown at the present what is the lowest limit of detection and limit of quantification for fingerprint residue) and highlight the selectivity and sensitivity of modern analytical techniques such as ion mobility mass spectrometry.
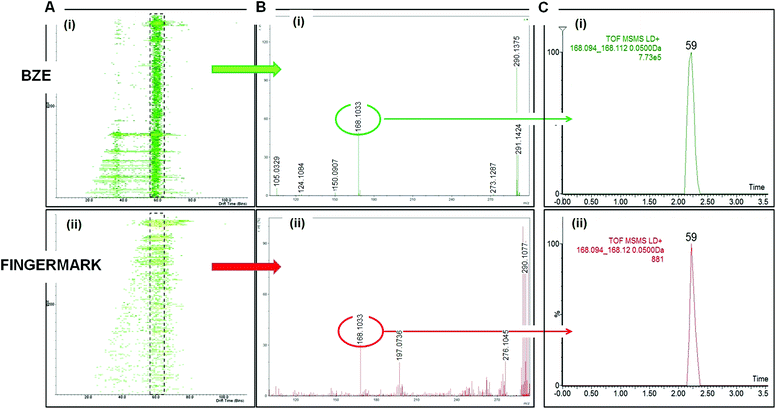 |
| Fig. 1 Detection of BZE from a fingerprint from a drug user in a rehabilitation centre by MALDI IMS-MS/MS. (Fig. 1A) Drift scope plots of BZE standard and BZE in the fingerprint (generated through transfer fragmentation experiment). (Fig. 1B) MS/MS spectra of BZE standard and BZE in the fingerprint after the selection of the BZE parent ion and products ion within the same drift time plume in the fingerprint and in the standard. Selection of the ion product at m/z 168.1033 in common to the BZE standard and BZE in fingerprint showing superimposable drift time chromatograms. | |
DESI
Analysis of the standards showed that detectable signals for cocaine and BZE were present above 5 ng ml−1, and above 50 ng ml−1 for EME. In Fig. 2, spectra are presented which show positive identification of cocaine, BZE and EME in a fingerprint sample. Fig. 2A shows the MS/MS spectrum from a fingerprint for ions with m/z 304, with the (M + H)+ peak for cocaine detected at a mass accuracy of 4.8 ppm (in full scan mode this was improved to 0.807 ppm) and the detection of the fragment ion at m/z 182. Similarly, Fig. 2B shows the MS/MS spectrum from a fingerprint for ions with m/z 290, with the (M + H)+ peak for BZE detected at a mass accuracy of 5.0 ppm (0.016 ppm in full scan mode), and detection of the fragment ion at m/z 168, confirming the presence of BZE in the fingerprint. No signal was detected when the spray was moved away from the fingerprint. Finally, Fig. 2C shows the MS/MS spectrum, using the linear ion trap analyser, from a fingerprint for ions with m/z 200, with the (M + H)+ peak for EME and detection of the fragment ion at m/z 182, confirming the presence of EME in the fingerprint. Using the Orbitrap mass analyser in full scan mode, EME was detected with a mass accuracy of 0.200 ppm. Table 1 compares the results of oral fluid testing and fingerprint testing from DESI of four donors’ fingerprints. Donors 1a and 1b represent different fingerprints from the same donor, and there is direct correspondence between the oral fluid test and the two fingerprints analysed by DESI. A similar result is seen for Donor 3. Donor 2's oral fluid tested negative, and the absence of a detectable signal in the DESI spectra from Donor 2's fingerprints are in direct agreement with this. The oral fluid test for Donor 4 tested positive for cocaine and this is also detected in Donor 4's fingerprint. However, EME and BZE were not detected in the fingerprints from this donor.
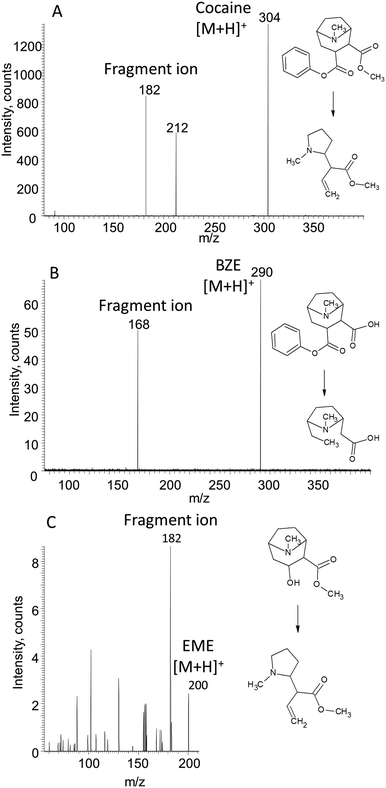 |
| Fig. 2 Detection of cocaine, BZE and EME in a fingerprint from a drug user attending a rehabilitation centre using DESI MS/MS. Spectra from a fingerprint with ions of m/z (Fig. 1A) 304 (cocaine) (Fig. 1B) 290 (BZE) (Fig. 1C) 200 (EME). | |
Table 1 Oral fluid test results and corresponding DESI and MALDI signals in the fingerprints of five donors
Donor |
Oral fluid screening result |
DESI fingerprint analysis |
Cocaine |
BZE |
EME |
DESI |
|
|
|
|
1a |
Positive |
y |
y |
y |
1b |
Positive |
y |
y |
y |
2 |
Negative |
n |
n |
n |
3 |
Positive |
y |
y |
y |
4 |
Positive |
y |
n |
n |
MALDI |
|
|
|
|
5 |
Positive |
y |
y |
N/A |
Table 2 presents data relating to the qualitative repeatability of the DESI method. Three fingerprints were taken from each of a further two donors who tested positive for cocaine in their oral fluid. Three separate points were analysed on each fingerprint. Table 2 shows that cocaine and EME were detected at every point analysed. BZE was detected in 17 out of 18 of the analysis points.
Table 2 DESI signals for cocaine, BZE and EME across three positions on three different fingerprints of two donors
|
Position |
Cocaine |
BZE |
EME |
Donor 6 |
Lthumb |
1 |
✓ |
✓ |
✓ |
2 |
✓ |
✓ |
✓ |
3 |
✓ |
⊠ |
✓ |
Rindex |
1 |
✓ |
✓ |
✓ |
2 |
✓ |
✓ |
✓ |
3 |
✓ |
✓ |
✓ |
Rring |
1 |
✓ |
✓ |
✓ |
2 |
✓ |
✓ |
✓ |
3 |
✓ |
✓ |
✓ |
Donor 7 |
Rindex |
1 |
✓ |
✓ |
✓ |
2 |
✓ |
✓ |
✓ |
3 |
✓ |
✓ |
✓ |
Rmiddle |
1 |
✓ |
✓ |
✓ |
2 |
✓ |
✓ |
✓ |
3 |
✓ |
✓ |
✓ |
Rthumb |
1 |
✓ |
✓ |
✓ |
2 |
✓ |
✓ |
✓ |
3 |
✓ |
✓ |
✓ |
SIMS
SIMS analysis of the standards revealed peaks at m/z 168, 124, 105 and 82, representing fragments of cocaine, BZE and EME. Molecular ion peaks could be seen only with the highest concentration of the analytes. In the latent fingerprint samples, only the peak at m/z 82 was detected.
Discussion
MALDI IMS-MS/MS and DESI analysis of a “natural” latent fingerprint allowed for the successful detection of the excreted primary metabolite, BZE. DESI was also able to detect EME and, at the time of the MALDI analysis, researchers were specifically asked to look for BZE only, therefore no comparative conclusions can be made on the feasibility of MALDI to detect this secondary metabolite.
The ability to detect excreted substances in latent fingerprints proves that surface mass spectrometry techniques could provide the drug testing industry with an exciting new and complementary tool, potentially allowing differentiation between drug consumption and contact solely based on the presence of metabolites in the residue. The significance of detecting cocaine metabolites in a fingerprint is currently unknown, because it is not known whether cocaine in fingerprint residue from contact could metabolise on the skin's surface. Furthermore, the possibility of absorption and metabolism of contact residues and the potential for secondary transfer has never been investigated. Although an image of the distribution of these excreted illicit substances in the fingerprint samples has not yet been attempted by either technique, the use of these techniques in profiling mode enables a rapid confirmatory screening for drugs of interest in less than 2 minutes.
The correspondence between the DESI and MALDI results and the oral fluid measurements is promising, but also shows the importance of developing a quantitative test with a cut-off level. The DESI result for Donor 4 shows that whilst this donor's oral fluid was above the oral fluid screening test cut-off level, only cocaine (and not BZE or EME) was detected in the fingerprint. This could be due to a difference in the (currently unknown) detection window for these metabolites in fingerprints compared with oral fluid or it may be a consequence of insufficient sensitivity of the DESI technique.
No quantification of the DESI data was attempted at this stage since a matrix-matched standard does not exist. Additionally, since the fingerprints are not spatially uniform, an appropriate sampling regime should be developed. The difficulty with quantification of a substance within fingerprint residue is that there is currently no accepted method for determining how much material was deposited in the finger deposition. In contrast, in blood, urine or oral fluid, the sample volume is known. It has been shown previously using an LC-MS method to detect caffeine in fingerprints that different fingers gave different results, presumably due to different contact pressure and/or deposition area from different fingers.19 We are currently investigating the validity of using different endogenous compounds within a fingerprint for normalisation purposes. Further studies are necessary in this area to establish a quantitative procedure.
Whilst SIMS has been previously shown to be a useful technique for imaging endogenous compounds in latent fingerprints,13,14 its suitability for the detection of drug metabolites in fingerprints is questionable, under the analytical conditions used here. The high level of fragmentation results in only the detection of the fragment ion at m/z 82 in fingerprint samples and standards of concentrations below 5000 ng ml−1. This fragment is common to cocaine, BZE and EME as well as other compounds, and therefore the selectivity is insufficient for toxicological application. It should be noted however, that Ar cluster SIMS sources can provide significantly higher molecular ion yields than Bi SIMS for organic compounds, and this may yield the desired selectivity in fingerprint samples. If this were the case, SIMS may provide superior opportunities for quantification compared with DESI and MALDI.
Conclusion
We have demonstrated the use of established and emerging analytical techniques for the analysis of cocaine and its metabolites in natural fingerprints. DESI was carried out under ambient conditions, and atmospheric pressure MALDI systems are also available. This, combined with the ability to analyse the sample in situ and with minimal sample preparation allows for very rapid sample throughput compared with chromatographic methods. The high sensitivity of the methods and selectivity to the analytes described has enabled us to detect metabolites of cocaine drugs from those who use illicit substances. Secondly, although the fingerprints are not spatially uniform, we are optimistic that these approaches can be developed for quantitative analysis of fingerprint residues.
Abbreviations
GC-MS | Gas chromatography mass spectrometry |
BZE | Benzoylecgonine |
EME | Methylecgonine |
DESI | Desorption electrospray ionisation mass spectrometry |
MALDI-IMS-MS/MS | Matrix assisted laser desorption ion mobility tandem mass spectrometry |
SIMS | Secondary ion mass spectrometry |
LC-MS | Liquid chromatography mass spectrometry |
SALDI | Surface assisted laser desorption ionisation |
Acknowledgements
The authors would like to thank the EPSRC Impact Acceleration Account for funding the DESI analysis in this work as well as Prof. J. F. Watts for access to the Surface Analysis Laboratory at the University of Surrey. The authors would also like to thank Dr Fiona Robinson, Sarah Hamilton and the service users of Surrey and Borders Partnership NHS Foundation Trust for their help with sample collection and the National Institute for Health Research for sponsoring this research.
References
- N. De Gionvanni and N. Fucci, The current status of sweat testing for drugs of abuse: a review, Curr. Med. Chem., 2013, 20, 545–561 Search PubMed.
- D. A. Kidwell, J. C. Holland and S. Athanaselis, Testing for drugs of abuse in saliva and sweat, J. Chromatogr., B: Anal. Technol. Biomed. Life Sci., 1998, 713, 111–135 CrossRef CAS.
- R. Leggett, E. L. Smith, S. M. Jickells and D. A. Rusell, “Intelligent” fingerprinting: simultaneous identification of drug metabolites and individuals by using antibody-functionalized nanoparticles, Angew. Chem., Int. Ed., 2007, 46, 4100–4103 CrossRef CAS PubMed.
- E. Goucher, A. Kicman, N. Smith and S. Jickells, The detection and quantification of lorazepam and its 3-O-glucuronide in fingerprint deposits by LC-MS/MS, J. Sep. Sci., 2009, 32, 2266–2272 CrossRef CAS PubMed.
- D. R. Ifa, N. E. Manicke, A. L. Dill and R. G. Cooks, Latent fingerprint chemical imaging by mass spectrometry, Science, 2008, 321, 805 CrossRef CAS PubMed.
- M. I. Szynkowska, K. Czerski, J. Rogowski, T. Paryjczak and A. Parczewski, ToF-SIMS application in the visualization and analysis of fingerprints after contact with amphetamine drugs, Forensic. Sci. Int., 2009, 184, e24–e26 CrossRef CAS PubMed.
- K. K. Sandquist, M. A. LeBeau and M. L. Miller, Chemical analysis of pharmaceuticals and explosives in fingermarks using matrix-assisted laser desorption ionization/time of flight mass spectrometry, Forensic. Sci. Int., 2014, 235, 68–77 CrossRef PubMed.
- F. Rowell, K. Hudson and K. Seviour, Detection of drugs and their metabolites in dusted latent fingerprints by mass spectrometry, Analyst, 2009, 134, 701–707 RSC.
- R. Wolstenholme, R. Bradshaw, M. R. Clench and S. Francese, Study of latent fingermarks by matrix-assisted laser desorption/ionisation mass spectrometry imaging of endogenous lipids, Rapid Commun. Mass Spectrom., 2009, 23, 3031–3039 CrossRef CAS PubMed.
- L. S. Ferguson, F. Wulfert, R. Wolstenholme, J. M. Fonville, M. R. Clench, V. A. Carolan and S. Francese, Direct detection of peptides and small proteins in fingermarks and determination of sex by MALDI mass spectrometry profiling, Analyst, 2012, 137, 4686–4692 RSC.
- R. Bradshaw, R. Wolstenholme, R. Blackledge, M. R. Clench, L. Ferguson and S. Francese, A novel MALDI MSI based methodology for the identification of sexual assault suspects, Rapid Commun. Mass Spectrom., 2011, 25, 415–422 CrossRef CAS PubMed.
- S. J. Hinder and J. F. Watts, SIMS fingerprint analysis on organic substrates, Surf. Interface Anal., 2010, 42, 826–829 CrossRef CAS PubMed.
- M. J. Bailey, N. J. Bright, R. S. Croxton, S. Francese, L. S. Ferguson and S. Hinder,
et al., Chemical characterization of latent fingerprints by matrix-assisted laser desorption ionization, time-of-flight secondary ion mass spectrometry, mega electron volt secondary mass spectrometry, gas chromatography/mass spectrometry, X-ray photoelectron spectroscopy, and attenuated total reflection Fourier transform infrared spectroscopic imaging: an intercomparison, Anal. Chem., 2012, 84, 8514–8523 CrossRef CAS PubMed.
- M. J. Bailey, M. Ismail, S. Bleay, N. Bright, M. Levin Elad and Y. Cohen,
et al., Enhanced imaging of developed fingerprints using mass spectrometry imaging, Analyst, 2013, 138(21), 6246–6250 RSC.
- F. M. Green, P. Stokes, C. Hopley, M. P. Seah, I. S. Gilmore and G. O'Connor, Developing repeatable measurements for reliable analysis of molecules at surfaces using DESI, Anal. Chem., 2009, 81, 2286–2293 CrossRef CAS PubMed.
- F. M. Green, T. L. Salter, I. S. Gilmore, P. Stokes and G. O'Connor, The effect of electrospray solvent composition on desorption electrospray ionisation (DESI) efficiency and spatial resolution, Analyst, 2010, 135, 731–737 RSC.
- S. Pringle, K. Giles, J. Wildgoose, J. Williams, S. Slade and K. Thalassinos,
et al., An investigation of the mobility separation
of some peptide and protein ions using a new hybrid quadrupole/travelling wave IMS/oa-ToF instrument, Int. J. Mass Spectrom., 2007, 261, 1–12 CrossRef CAS PubMed.
- LGC, Queens Road, Teddington, TW11 0LY, UK.
- S. Jacob, S. Jickells, K. Wolff and N. Smith, Drug testing by chemical analysis of fingerprint deposits from methadone-maintained opioid dependent patients using UPLC-MS/MS, Drug Metab.Lett., 2008, 2, 245 CrossRef CAS.
Footnote |
† Equally contributing first authors. |
|
This journal is © The Royal Society of Chemistry 2015 |
Click here to see how this site uses Cookies. View our privacy policy here.