Open Access Article
Free-standing poly (N-isopropylacrylamide) microgel-based etalons
Received
29th April 2014
, Accepted 8th June 2014
First published on 20th June 2014
Abstract
Poly (N-isopropylacrylamide-co-acrylic acid) (pNIPAm-co-AAc) microgel-based free-standing etalons have been developed by fabricating etalons on a solid support that has been coated with a sacrificial polymer layer. Therefore, the etalon could be easily removed from the solid substrate upon dissolution of the sacrificial layer. We show that the free-standing etalons retain the optical properties, and the temperature/pH responsivity, of the etalon before its removal from the solid substrate. Furthermore, we show that the free-standing etalons could be transferred to a variety of different substrates, with different chemistries and shapes. We go on to show that the free-standing etalons could be loaded with a small molecule model drug Crystal Violet (CV), transferred to a hydrogel, and triggered to release the CV in a pH-dependent fashion. In the future, these novel free-standing films can be used for sensing, as transferable optical materials, and will be further developed into drug delivery platforms.
Introduction
Stimuli responsive materials1–3 or smart materials are able to “sense” changes in their environment by undergoing a chemical and/or physical transition. Ideally, the response is reversible, i.e., once the stimulus is removed, the responsive material returns to its initial state. A number of responsivities have been introduced into responsive materials including responsivity to: temperature, pH, ionic strength, light, electric and magnetic field.1,4–6 These materials have found a number of applications as electronic materials, for controlled/triggered drug delivery, as antibacterial coatings and for tissue engineering.7 Considering all of the responsive polymer-based materials discovered to date, thermoresponsive poly (N-isopropylacrylamide) (pNIPAm) is by far the most extensively studied and used. PNIPAm is well known to be fully water soluble in water (and swollen) at temperatures below 32° C, while it transitions to an “insoluble” (deswollen) state when the water temperature is increased to above 32° C – this transition is known as a coil to globule transition.8
NIPAm can also be polymerized in the presence of a crosslinker to yield a network polymer. Like linear pNIPAm, these polymer networks (hydrogels) are swollen with water at T < 32 °C and deswollen at T > 32° C. Additionally, colloidal pNIPAm-based hydrogels (microgels and nanogels depending on their diameter) can also be synthesized.9,10 Regardless of their dimensions, hydrogels can easily be modified with additional functional comonomers by simple copolymerization.11 For example, ionizable comonomers, such as methacrylic acid and acrylic acid (AAc), render the microgels pH responsive, in addition to thermoresponsive. Specifically, AAc has a pKa of ∼4.25; therefore at pH > 4.25 the microgels are deprotonated (negatively charged) and relatively swollen compared to the microgels at pH < 4.25, which are protonated (neutral). The acid groups also allow the microgels to be easily modified by further reaction with other functional molecules.
A wide variety of responsive polymer-based thin films have been fabricated using both polymerization (e.g., atom transfer radical polymerization12–14) and deposition techniques (e.g., layer-by-layer assembly15–19). While substrate-supported thin films are of utmost importance for a number of applications, there are instances where free-standing thin films are beneficial, and have recently received a lot of attention. Compared to substrate-bound thin films, free-standing materials are: flexible; able to change size, shape, and/or conformation; easily accessible from all dimensions, and oftentimes more sensitive to environmental stimuli than substrate-supported thin films. A variety of approaches have been developed to fabricate free-standing responsive films,20–22 one of the most facile ways to accomplish this is utilizing layer-by-layer (LbL) assembly. In one case (with charged components) a charged substrate is exposed alternately to solutions containing positively or negatively charged polymers (polyelectrolytes). The interactions between the substrate charges and the polyelectrolyte allow a substrate-attached thin film to be formed. Since these films are held together by electrostatics, they can be destabilized in solutions of extreme pHs and ionic strength.18,23
Our group has recently fabricated optical materials composed of a pNIPAm-based microgel layer sandwiched between two thin metal layers.24–27 A schematic depiction of the device, also known as an etalon, can be seen in Fig. 1a. As can be seen in Fig. 1b, these devices exhibit unique multipeak reflectance spectra, which we have exploited for sensing applications.28,29 The position of the peaks in the reflectance spectrum depends on the distance between the device's two Au layers and the refractive index of microgels as shown in eqn (1).26
| λm = 2nd cos θ | (1) |
where
λ is the wavelength maximum of the peak (s),
m is the peak order,
n is the refractive index of the dielectric,
d is the spacing between the mirrors, and
θ is the angle of incidence.
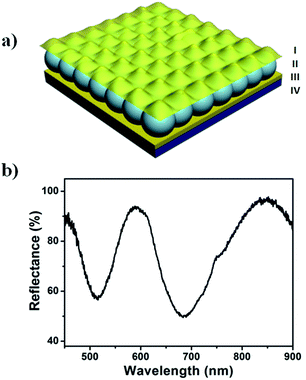 |
| Fig. 1 (a) Basic construct of a microgel-based etalon. (I) and (III) are 15 nm Au layers (a 2 nm Cr adhesion layer below the Au layer is not shown); (II) monolithic pNIPAm-co-AAc microgel layer; and (IV) glass substrate. (b) Characteristic reflectance spectrum for a pNIPAm-co-AAc microgel-based etalon in water. | |
While we have shown that microgel-based etalons can be fabricated on various planar substrates, there are some substrates that are difficult to coat with etalons, e.g., curved surfaces, rods, and tubes. Furthermore, for our sensing and monitoring efforts, it is advantageous to fabricate devices that can be adhered to skin.30–32 To achieve this, it would be beneficial to fabricate microgel-based etalons on planar substrates, followed by their desorption. The desorbed etalon can then be adhered to any other substrate as needed. In this submission, we describe the fabrication of free-standing pNIPAm-co-AAc microgel-based etalons that exhibit high quality optical properties, which are capable of being transferred to multiple substrates. The desorbed etalons exhibit similar optical properties to the substrate adhered etalons, and retain their pH and temperature responsivity.
Experimental section
Materials
N-Isopropylacrylamide was purchased from TCI (Portland, Oregon) and purified by recrystallization from hexanes (ACS reagent grade, EMD, Gibbstown, NJ) prior to use. N,N′-Methylenebisacrylamide (99%), acrylic acid (99%), H2O2 (30 wt%) and ammonium persufate (98+%) were obtained from Aldrich (St. Louis, MO) and were used as received. Poly (diallyldimethylammonium chloride) (Mw = 100
000–200
000) (PDADMAC, 20 wt% in water) and poly(allylamine hydrochloride) (Mw = 55
000–65
000) (PAH) were obtained from Aldrich as solutions. Poly (sodium 4-styrenesulfonate) (Mw = 70
000) (PSS, Aldrich) and poly(acrylic acid) (Mw = 90
000) (PAA, Polysciences) were used as an anionic polyelectrolyte. H2SO4 (98%) was purchased from Caledon Chemicals (Alberta) and was used as received. Anhydrous ethanol was obtained from Commercial Alcohols (Brampton, Ontario). Hydrochloric acid and sodium hydroxide were purchased from Caledon Chemicals (Alberta). All deionized water (DI) was 18.2 M Ω cm and obtained from a Milli-Q Plus system from Millipore (Billerica, MA). Au annealing was performed in an Isotemp muffle furnace from Fisher Scientific (Ontario, Canada). Microscope glass slides were 25 mm × 75 mm × 1.0 mm and obtained from Fisher Scientific. Cr (99.999%) was obtained from ESPI (Ashland, OR), while Au (99.99%) was obtained from MRCS Canada (Edmonton, AB). Photographs of the films were taken with a Canon Power shot SD20 Digital ELPH SD.
Microgel synthesis
Microgels composed of poly (N-isopropylacrylamide-co-acrylic acid) (pNIPAm-co-AAc) were synthesized via surfactant-free, free radical precipitation polymerization as described previously.24,26 The monomer mixture was comprised of 85% N-isopropylacrylamide (NIPAm) and 10% acrylic acid (AAc) with 5% N,N′-methylenebisacrylamide (BIS) as the crosslinker. The monomer, NIPAm (11.9 mmol), and the crosslinker, BIS (0.703 mmol), were dissolved in DI water (99 mL) with stirring in a small beaker. The mixture was filtered through a 0.2 μm filter affixed to a 20 mL syringe into a 250 mL, 3-necked round bottom flask. The flask was then fitted with a temperature probe, a condenser/N2 outlet, stir bar, and a N2 inlet. The monomer solution was purged with N2 gas for ≈1 h with stirring, while the temperature was allowed to reach 70 °C. AAc (1.406 mmol) was added to the heated mixture with a micropipette. An aqueous solution of ammonium persulfate (APS, 0.046 g in 1.0 mL) was delivered to the monomer solution with a transfer pipette to initiate the reaction, and reaction was continued for 4 h. Following completion of the reaction, the reaction mixture was filtered through glass wool to remove any large aggregates. The coagulum was rinsed with deionized water and the reaction solution was diluted to ≈120 mL. Aliquots of these particles (13 mL) in centrifuge tubes were centrifuged at a speed of ≈8500 relative centrifugal force (rcf) at 20 °C to produce a pellet. The supernatant was removed from the pellet of particles, which were then re-suspended to their original volume (13 mL) using deionized water. This process was completed a total of six times to remove the unreacted monomer and linear polymer from the microgels.
Sacrificial layer fabrication
25 mm × 25 mm glass microscope slides were cleaned and hydroxylated in Piranha solution (mixture of 7
:
3(v/v) 98% H2SO4 and 30% H2O2) (Caution: piranha solutions react violently with organic materials and should not be stored in closed containers). They were then rinsed copiously with deionized water and dried under a stream of nitrogen before use. Layer-by-layer (LbL) self-assembly was used to form the sacrificial layer on the glass substrate. Specifically, the freshly cleaned glass substrate was immersed in a PDADMAC aqueous solution (1 mg mL−1, pH 6.5) for 15 min to obtain a cation-terminated surface and the substrate was then rinsed by immersion in deionized water for 15 min before the next layer was deposited. Next, the substrate was immersed into an aqueous PSS solution (1 mg mL−1, pH 6.5) for 15 min to obtain an anion-terminated surface. The rinsing and adsorption steps were repeated until the desired number of bi-layers was obtained. Finally, the prepared substrate was dried with nitrogen and used. PAH/PAA multilayers were assembled in the same way, although the pH of the PAH solution was adjusted to 7.5, while the pH of the PAA solution was 3.5.23
Etalon fabrication
Etalons were prepared following a previously published method.27 To fabricate etalons on the modified coverslips, 2 nm Cr and 15 nm of Au was thermally evaporated onto the substrate at a rate of 0.2 Å s−1, and 0.1 Å s−1, respectively (Torr International Inc., thermal evaporation system, Model THEUPG, New Windsor, NY). A 40 μL aliquot of concentrated microgels (from viscous pellet formed via centrifugation of a microgel solution) was added to the substrate and then spread toward each edge using the side of a micropipette tip. The film was rotated 90°, and the microgel solution was spread again. The spreading and rotation continued until the microgel solution became too viscous to spread due to drying. This process was done with extreme care as to not damage the sacrificial polymer layer on the glass. The microgel solution was allowed to dry completely on the substrate for 2 h with the hot plate temperature set to 35 °C. After that, the dry film was rinsed copiously with DI water to remove any excess microgels not bound directly to the Au. The film was then placed into a DI water bath and allowed to incubate overnight on a hot plate set to ∼30 °C. Following this step, the substrate was again rinsed with DI water to further remove any microgels not bound directly to the Au substrate surface. The samples were then dried with N2 gas and another Au overlayer (2 nm Cr for adhesion layer) was evaporated. To yield a free-standing etalon, the whole substrate was immersed in an aqueous sodium hydroxide aqueous solution (1 M) to remove the sacrificial layer. The free-standing etalons were transferred to a variety of substrates with no previous treatment.
Reflectance spectroscopy
Reflectance measurements were conducted using a Red Tide USB650 spectrometer, a LS-1 tungsten light source, and a reflectance probe from Ocean Optics (Dunedin, FL). The spectra were recorded using Ocean Optics Spectra Suite Spectroscopy Software at room temperature over a wavelength range of 400–1000 nm. Measurements were conducted by placing the film in a Petri dish with water at room temperature. The probe tip was immersed in the water and its distance from the etalon surface adjusted for optimal signal. The probe remained undisturbed between measurements to ensure that all of the spectra were taken in the same manner. The film was moved under the probe and at least three measurements were taken for each film in different areas.
Scanning electron microscopy
SEM images were obtained with a JSM-6010LA JEOL (Peabody, MA) SEM. Samples were tilted 30° (unless otherwise indicated) relative to the source. Etalons were imaged by transferring the free-standing etalon onto a clean silica substrate and then dried under nitrogen gas flow.
Results and discussion
To achieve free-standing etalons, microgel-based etalons were fabricated on LBL-deposited sacrificial thin polymer films, all on a glass substrate. A schematic of the fabrication protocol is shown in Fig. 2. Specifically, a glass substrate was cleaned and hydroxylated by immersion in piranha solution for 4 h (Caution: piranha solution is extremely dangerous – handle with care). Then the LBL multilayer film was constructed on the glass surface. Here, two polyelectrolyte systems were used, poly (diallyldimethylammonium chloride) (PDADMAC)/poly (sodium 4-styrenesulfonate) (PSS) and poly(allylamine hydrochloride) (PAH)/poly(acrylic acid) (PAA). Briefly, the glass was subsequently immersed into 1 mg mL−1 aqueous solution of PDADMAC solution (pH 6.5) for 15 min, followed by immersing into DI water for 15 min for rinsing. The substrate was then immersed in a 1 mg mL−1 solution of PSS (pH 6.5), for another 15 min, and again in DI water for 15 min. The process was repeated until 10 bilayers (PDADMAC/PSS is a bilayer) were formed on the glass. The (PAH/PAA)10 layers were formed in the same way, using different solution pH's, as indicated in the experimental section.23 Following thin film formation, 15 nm of Au was deposited (via thermal evaporation) on top of the LbL assembled thin film, followed by microgel deposition on the Au layer following our previously published painting protocol.27 Another Au layer was subsequently deposited on top of the microgel layer to yield the etalon's Au–microgel–Au sandwich structure. A schematic of the device can be seen in Fig. 1, along with a representative reflectance spectrum for the etalon in water.
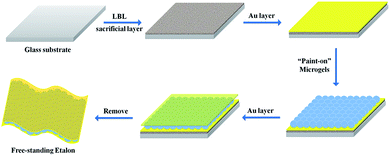 |
| Fig. 2 Schematic depiction of the etalon assembly and removal process. First, a sacrificial LbL-assembled film was deposited on the glass followed by the etalon fabrication on the sacrificial layer. Finally, the assembly was exposed to a 1 M NaOH aqueous solution, which dissolves the sacrificial layer, and releases the etalon. | |
To generate a free-standing etalon, the assembly was immersed into a 1 M aqueous solution of NaOH. This causes the sacrificial layer to dissolve, and the etalon to be released from the glass substrate. The disassembly and etalon release process is shown in Fig. 3. As can be seen, when the assembly was immersed into the high pH solution the corners began to delaminate from the glass after ∼2 minutes, while the whole etalon was removed from the glass substrate within 10 min, see Fig. 3(a, b) and (d, e). In both cases, the etalon was released from the substrate due to a combination of effects. Considering the assembly on glass, the etalon could be released from the substrate by breaking the polymer-glass, polymer–polymer, and/or polymer–Cr/Au interactions. In the case of the weak polyelectrolytes (PAH/PAA), the high pH most likely broke the polymer–polymer interactions, while a combination of these effects was most likely responsible for the disassembly of the PDADMAC/PSS layers. In fact, the etalon was removed from the substrate much faster with the PAH/PAA sacrificial layers. We point out here that the edges of the slide were scratched before immersion of the etalon into the high pH solution, which we found to assist with the peeling process. Fig. 3b shows a free-standing etalon film in the basic solution. As can be seen in Fig. 3c and f, the optical properties of the free-standing etalons were retained after peeling from the substrate surface. Additionally, the free-standing etalon can be dried and rehydrated with no apparent effect on the optical properties.
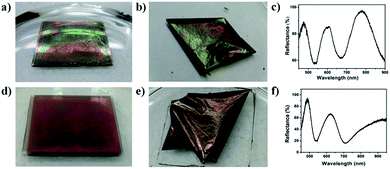 |
| Fig. 3 (a) Photograph of the substrate-bound etalon (PDADMAC/PSS sacrificial layer) immersed in 1 M NaOH solution and (b) after desorption. (c) After desorption, the etalon still exhibits the unique, multipeak reflectance spectrum. (d) Photograph of the substrate-bound etalon (PAH/PAA sacrificial layer) immersed in 1 M NaOH solution and (e) after ∼8 minutes NaOH exposure. (f) Again, after desorption, the etalon still exhibits the unique, multipeak reflectance spectrum. The size of the glass substrate above was 2.5 cm × 2.5 cm. | |
To investigate the structure of the free-standing etalon, and to verify the Au–microgel–Au structure was still intact after desorption from the glass substrate, scanning electron microscopy (SEM) was performed. To accomplish this, the free-standing etalon was transferred to a silicon substrate and imaged. As can be seen in Fig. 4a–c, the full etalon structure remains intact after desorption, indicating that the pH treatment doesn't affect the device's structure, and that the microgels are still sandwiched between the two Au layers. The free-standing etalon film can be completely broken into small pieces by ultrasonication, as shown in Fig. 4d. This result may provide us a way to prepare a single microgel-based etalon element in the future, which may have unique optical properties and potential applications for sensing and drug delivery.
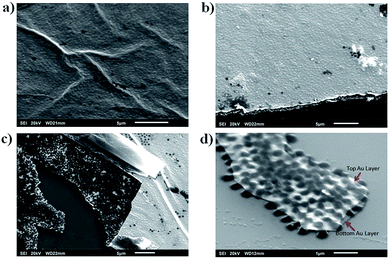 |
| Fig. 4 SEM images of a free standing etalon from: (a and c) the top, (b and d) tilted 30°. As can be seen in (a and b), the etalon structure remained intact after desorption from the solid substrate, and the microgels remained sandwiched between the two Au layers. As can be seen in (c), there are two Au layers present. (d) The structure of an etalon after sonication. Sonication breaks up the etalon into small pieces, which appear to retain the etalon structure. | |
Next, we wanted to confirm that the free-standing etalons could be transferred to different substrates and that their optical properties remained intact. To accomplish this, we desorbed etalons from the solid glass substrates and adhered them to different substrates, such as: silicon, polydimethylsiloxane (PDMS), plastic and paper. After the free-standing films were transferred onto the different substrates, the reflectance spectra were collected; the results are shown in Fig. 5. As can be seen in Fig. 5a, the reflectance spectrum of the etalon film on a silicon substrate shows multiple peaks similar to the original etalon device made on glass substrate (Fig. 1b). As can be seen in Fig. 5b–d, similar spectra could be observed for a free-standing etalon transferred to PDMS, plastic and paper, respectively. For the “plastic” substrate, we used a piece of a nitrile lab gloves as an example. When the etalon film was transferred onto the nitrile, the film was not flat based due to its deposition on the elastic substrate, but it still exhibited a reflectance spectrum that is characteristic to the etalon (Fig. 5c). Finally, filter paper was used as a substrate of the etalon film in Fig. 5d. While the optical properties of this film were comparable to the initial substrate-bound etalon, there were some differences due to the porosity of the paper. The porosity, combined with the capillary effects of the paper made the etalon difficult to hydrate.
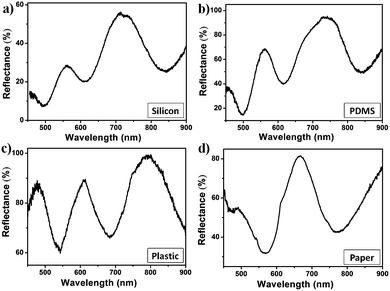 |
| Fig. 5 Reflectance spectra for etalons desorbed from the initial glass substrate and transferred to: (a) silicon; (b) PDMS; (c) plastic/nitrile and (d) paper. | |
Additionally, we wanted to show the utility of the approach for coating curved surfaces and even skin with an etalon. This could find applications in the biomedical area, and for real time health monitoring applications.31–35 To accomplish this, we desorbed an etalon from a glass substrate and (like above) and transferred it to the outside of a glass tube with a diameter of 1 cm. The resultant etalon can be seen in Fig. 6a. As can be seen, the etalon structure remains intact, and retains its optical properties as can be seen in Fig. 6b. Furthermore, a free-standing etalon was transferred to human skin, as shown in Fig. 6c. Similarly, the etalon remained intact and the optical properties were unaffected.
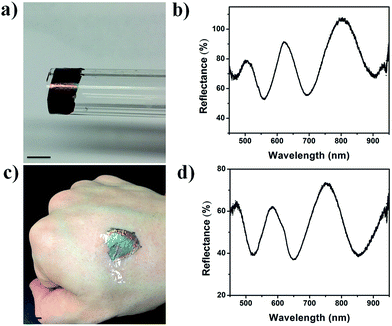 |
| Fig. 6 A free-standing etalon transferred to (a) a glass tube, and (c) skin. (b and d) The reflectance spectra obtained from the devices. The scale bar is 0.5 cm. | |
In our previous studies, we showed that etalon devices have unique responsivity to a variety of stimuli, specifically, they are very sensitive to temperature and pH changes (among other things).26,27,36–38 Therefore, we investigated the responsivity and sensitivity of the free-standing etalons to temperature and pH. To accomplish this, the free-standing etalon was desorbed from the initial glass substrate and added to a bare silicon substrate. The results are shown in Fig. 7. Fig. 7a shows that the etalon is extremely sensitive to solution pH, i.e., the reflectance peak red shifts a total of ∼330 nm upon increasing the solution pH from 3.17 to 9.32. This is due to the deprotonation of AAc at high pH, which leads to enhanced Coulombic repulsion in the microgel network and swelling. The temperature-dependent optical properties of the etalon are shown in Fig. 7b at different solution pH. We found that the etalon was fully responsive to temperature at pH 3.0, while it was minimally responsive to temperature at high pH. This behavior is similar to etalons that have not been desorbed from the glass substrate and is a result of the charges in the microgels at high pH preventing the microgel collapse at elevated temperature.26,39 At pH 3.0, the AAc groups are neutral and therefore there is no barrier for the microgel deswelling at elevated temperature.
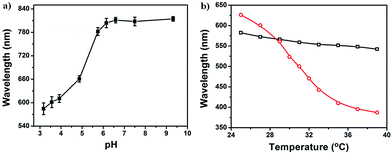 |
| Fig. 7 (a) Response of the free-standing etalon film to pH, and (b) temperature at different pH's. As can be seen in (b), the etalon responds to temperature at pH = 3.0 (red), while the thermal response is hindered at pH = 6.0 (black). This is due to the deprotonated AAc groups preventing the collapse of the microgels. | |
Finally, to further demonstrate the applicability of the free-standing etalons, we showed that they could be used to release a small molecule model drug once absorbed onto a polymer matrix. To accomplish this, the small molecule crystal violet (CV, model drug) was loaded into the etalon's microgel layer at a given pH, while it was released at another pH. Specifically, at pH > 4.25 (pKa for AAc) the microgels were negatively charged, while the CV is positively charged, thus the microgels were able to absorb the CV via electrostatic interactions. When the pH was lowered to <4.25 the AAc groups were neutralized, releasing the CV.40 To load the free-standing etalon with CV, it was immersed into 20 mL of an aqueous solution of CV (0.5 g L−1, pH 6.5) for 4 h. It was then transferred to a glass substrate (used as a support) and gently rinsed with pH 6.5 solution to remove the excess CV. Finally, the edges of etalon were sealed with epoxy and put in direct contact with a pNIPAm-based hydrogel (15 mm × 15 mm × 2.5 mm) synthesized following standard protocol.41 The hydrogels were swollen with different pH solutions, by soaking them for more than 5 h in solutions of pH 3.0 (release) and pH 6.5 (no release). We point out that the pNIPAm-based hydrogel was used purely out of convenience, and only served as a generic polymer matrix in this case. The results are shown in Fig. 8, which reveals that the hydrogel immersed in pH 3.0 solution was able to induce the etalon to release the loaded CV (by protonating the AAc groups), while the hydrogel immersed in pH 6.5 solution did not because the electrostatic interactions were still strong. This results show that the free-standing etalons could be adhered to “foreign” substrates, e.g., skin, and be triggered to release small molecules in a controlled fashion. This specifically demonstrates the utility of the devices for drug delivery applications.
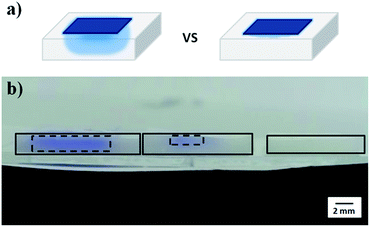 |
| Fig. 8 (a) Schematic showing that a “drug” loaded free-standing etalon can be transferred to a hydrogel that was swollen in solutions of (left) pH 3.0 and (right) 6.5. (b) As can be seen, the small molecule can be released from the etalon at (left) pH 3.0, while minimal CV was released at (middle) pH 6.5. (right) The hydrogel before contact with the CV loaded free-standing etalon. The black dashed lines indicate the region in the hydrogel where CV was located, while the black solid lines indicate the individual hydrogel boundaries. | |
Conclusions
In summary, a simple method for fabricating free-standing etalons was developed by introducing a sacrificial layer between the etalon and glass substrate. The sacrificial layer was prepared by LbL assembly, which could be destroyed in solutions at high pH; destroying the film releases the etalon from the substrate. The free-standing etalon film can be easily transferred to substrates with different chemistries and shapes. We found that the etalon's optical properties are retained after desorption from the solid substrate, which continue to exhibit very sensitive responses to solution pH and temperature. Finally, we demonstrate that the devices could be transferred to another surface, in this case a hydrogel, and be triggered to release a small molecule “drug”. In the future, the free-standing etalon will be used for light filtering applications, biosensing, we will further the drug delivery concept.
Acknowledgements
M.J.S. acknowledges funding from the University of Alberta (the Department of Chemistry and the Faculty of Science), the Natural Sciences and Engineering Research Council of Canada (NSERC), the Canada Foundation for Innovation (CFI), the Alberta Advanced Education & Technology Small Equipment Grants Program (AET/SEGP), and Grand Challenges Canada. M.J.S. also acknowledges Mark McDermott for the use of the thermal evaporator.
References
- M. A. C. Stuart, W. T. Huck, J. Genzer, M. Müller, C. Ober, M. Stamm, G. B. Sukhorukov, I. Szleifer, V. V. Tsukruk and M. Urban, Emerging applications of stimuli-responsive polymer materials, Nat. Mater., 2010, 9, 101–113 CrossRef PubMed.
- I. Tokarev and S. Minko, Stimuli-responsive hydrogel thin films, Soft Matter, 2009, 5, 511–524 RSC.
- I. Tokarev, M. Motornov and S. Minko, Molecular-engineered stimuli-responsive thin polymer film: a platform for the development of integrated multifunctional intelligent materials, J. Mater. Chem., 2009, 19, 6932–6948 RSC.
- T. Chen, R. Ferris, J. Zhang, R. Ducker and S. Zauscher, Stimulus-responsive polymer brushes on surfaces: transduction mechanisms and applications, Prog. Polym. Sci., 2010, 35, 94–112 CrossRef CAS PubMed.
- L. Zhai, Stimuli-responsive polymer films, Chem. Soc. Rev., 2013, 42, 7148–7160 RSC.
- G. Stoychev, S. Turcaud, J. W. Dunlop and L. Ionov, Hierarchical Multi-Step Folding of Polymer Bilayers, Adv. Funct. Mater., 2013, 23, 2295–2300 CrossRef CAS.
- T. Miyata, N. Asami and T. Uragami, A reversibly antigen-responsive hydrogel, Nature, 1999, 399, 766–769 CrossRef CAS PubMed.
- C. Wu and S. Zhou, Laser light scattering study of the phase transition of poly (N-isopropylacrylamide) in water. 1. single chain, Macromolecules, 1995, 28, 8381–8387 CrossRef CAS.
- R. Pelton and P. Chibante, Preparation of aqueous latices with N-isopropylacrylamide, Colloids Surf., 1986, 20, 247–256 CrossRef CAS.
- J. D. Debord, S. Eustis, S. Byul Debord, M. T. Lofye and L. A. Lyon, Color-Tunable Colloidal Crystals from Soft Hydrogel Nanoparticles, Adv. Mater., 2002, 14, 658–662 CrossRef CAS.
- J. D. Debord and L. A. Lyon, Synthesis and characterization of pH-responsive copolymer microgels with tunable volume phase transition temperatures, Langmuir, 2003, 19, 7662–7664 CrossRef CAS.
- J.-S. Wang and K. Matyjaszewski, Controlled/ “living” radical polymerization. Atom transfer radical polymerization in the presence of transition-metal complexes, J. Am. Chem. Soc., 1995, 117, 5614–5615 CrossRef CAS.
- J.-S. Wang and K. Matyjaszewski, Controlled/ “living” radical polymerization. Halogen atom transfer radical polymerization promoted by a Cu(I)/Cu(II) redox process, Macromolecules, 1995, 28, 7901–7910 CrossRef CAS.
- K. Matyjaszewski and J. Xia, Atom transfer radical polymerization, Chem. Rev., 2001, 101, 2921–2990 CrossRef CAS PubMed.
- G. Decher, Fuzzy nanoassemblies: toward layered polymeric multicomposites, Science, 1997, 277, 1232–1237 CrossRef CAS.
- Y. Lvov, K. Ariga, I. Ichinose and T. Kunitake, Assembly of multicomponent protein films by means of electrostatic layer-by-layer adsorption, J. Am. Chem. Soc., 1995, 117, 6117–6123 CrossRef CAS.
- F. Caruso, K. Niikura, D. N. Furlong and Y. Okahata, 1. Ultrathin multilayer polyelectrolyte films on gold: construction and thickness determination, Langmuir, 1997, 13, 3422–3426 CrossRef CAS.
- C. Jiang, S. Markutsya and V. V. Tsukruk, Compliant, robust, and truly nanoscale free-standing multilayer films fabricated using spin-assisted layer-by-layer assembly, Adv. Mater., 2004, 16, 157–161 CrossRef CAS.
- C. Jiang and V. V. Tsukruk, Freestanding Nanostructures via Layer-by-Layer Assembly, Adv. Mater., 2006, 18, 829–840 CrossRef CAS.
- K. E. Shopsowitz, H. Qi, W. Y. Hamad and M. J. MacLachlan, Free-standing mesoporous silica films with tunable chiral nematic structures, Nature, 2010, 468, 422–425 CrossRef CAS PubMed.
- D. Xu, Q. Xu, K. Wang, J. Chen and Z. Chen, Fabrication of Free-Standing Hierarchical Carbon Nanofiber/Graphene Oxide/Polyaniline Films for Supercapacitors, ACS Appl. Mater. Interfaces, 2013, 6, 200–209 Search PubMed.
- S. H. Wibowo, E. H. Wong, A. Sulistio, S. N. Guntari, A. Blencowe, F. Caruso and G. G. Qiao, Assembly of Free-Standing Polypeptide Films via the Synergistic Combination of Hyperbranched Macroinitiators, the Grafting-From Approach, and Cross-Chain Termination, Adv. Mater., 2013, 25, 4619–4624 CrossRef CAS PubMed.
- S. S. Shiratori and M. F. Rubner, pH-dependent thickness behavior of sequentially adsorbed layers of weak polyelectrolytes, Macromolecules, 2000, 33, 4213–4219 CrossRef CAS.
- C. D. Sorrell and M. J. Serpe, Reflection Order Selectivity of Color-Tunable Poly (N-Isopropylacrylamide) Microgel Based Etalons, Adv. Mater., 2011, 23, 4088–4092 CrossRef CAS PubMed.
- L. Hu and M. J. Serpe, Color-tunable etalons assembled from poly(N-isopropylacrylamide) based microgels, Polymers, 2012, 4, 134–149 CrossRef CAS PubMed.
- C. D. Sorrell, M. C. Carter and M. J. Serpe, Color Tunable Poly(N-Isopropylacrylamide)-co-Acrylic Acid Microgel–Au Hybrid Assemblies, Adv. Funct. Mater., 2011, 21, 425–433 CrossRef CAS.
- C. D. Sorrell, M. C. Carter and M. J. A. Serpe, “paint-on” protocol for the facile assembly of uniform microgel coatings for color tunable etalon fabrication, ACS Appl. Mater. Interfaces, 2011, 3, 1140–1147 CAS.
- C. D. Sorrell and M. J. Serpe, Glucose sensitive poly (N-isopropylacrylamide) microgel based etalons, Anal. Bioanal. Chem., 2012, 402, 2385–2393 CrossRef CAS PubMed.
- M. R. Islam and M. J. Serpe, Polyelectrolyte mediated intra and intermolecular crosslinking in microgel-based etalons for sensing protein concentration in solution, Chem. Commun., 2013, 49, 2646–2648 RSC.
- Z. Bian, J. Song, R. C. Webb, A. P. Bonifas, J. A. Rogers and Y. Huang, Thermal analysis of ultrathin, compliant sensors for characterization of the human skin, RSC Advances, 2014, 4, 5694–5697 RSC.
- W. H. Yeo, Y. S. Kim, J. Lee, A. Ameen, L. Shi, M. Li, S. Wang, R. Ma, S. H. Jin and Z. Kang, Multifunctional epidermal electronics printed directly onto the skin, Adv. Mater., 2013, 25, 2773–2778 CrossRef CAS PubMed.
- R. C. Webb, A. P. Bonifas, A. Behnaz, Y. Zhang, K. J. Yu, H. Cheng, M. Shi, Z. Bian, Z. Liu and Y.-S. Kim, Ultrathin conformal devices for precise and continuous thermal characterization of human skin, Nat. Mater., 2013, 12, 938–944 CrossRef CAS PubMed.
- L. Persano, C. Dagdeviren, Y. Su, Y. Zhang, S. Girardo, D. Pisignano, Y. Huang and J. A. Rogers, High performance piezoelectric devices based on aligned arrays of nanofibers of poly (vinylidenefluoride-co-trifluoroethylene), Nat. Commun., 2013, 4, 1633 CrossRef PubMed.
- D.-H. Kim, N. Lu, Y. Huang and J. A. Rogers, Materials for stretchable electronics in bioinspired and biointegrated devices, MRS bulletin, 2012, 37, 226–235 CrossRef CAS.
- X. Huang, W.-H. Yeo, Y. Liu and J. A. Rogers, Epidermal differential impedance sensor for conformal skin hydration monitoring, Biointerphases, 2012, 7, 1–9 CrossRef PubMed.
- M. R. Islam and M. J. Serpe, Penetration of Polyelectrolytes into Charged Poly (N-Isopropylacrylamide) Microgel Layers Confined Between Two Surfaces, Macromolecules, 2013, 46, 1599–1606 CrossRef CAS.
- I. N. Heppner and M. J. Serpe, Poly (N-isopropylacrylamide) microgel-based etalons constructed from various metal layers, Colloid Polym. Sci., 2013, 1–6 Search PubMed.
- M. R. Islam, K. C. Johnson and M. J. Serpe, Microgel-based etalon coated quartz crystal microbalances for detecting solution pH: The effect of Au overlayer thickness, Anal. Chim. Acta, 2013, 792, 110–114 CrossRef CAS PubMed.
- M. C. Carter, C. D. Sorrell and M. J. Serpe, Deswelling kinetics of color tunable poly (N-isopropylacrylamide) microgel-based etalons, J. Phys. Chem. B, 2011, 115, 14359–14368 CrossRef CAS PubMed.
- Y. Gao, G. P. Zago, Z. Jia and M. J. Serpe, Controlled and Triggered Small Molecule Release from a Confined Polymer Film, ACS Appl. Mater. Interfaces, 2013, 5, 9803–9808 CAS.
- A. Rotzetter, C. Schumacher, S. Bubenhofer, R. Grass, L. Gerber, M. Zeltner and W. Stark, Thermoresponsive polymer induced sweating surfaces as an efficient way to passively cool buildings, Adv. Mater., 2012, 24, 5352–5356 CrossRef CAS PubMed.
|
This journal is © The Royal Society of Chemistry 2014 |
Click here to see how this site uses Cookies. View our privacy policy here.