DOI:
10.1039/C3SC53076K
(Edge Article)
Chem. Sci., 2014,
5, 1312-1316
Sandmeyer trifluoromethylthiolation of arenediazonium salts with sodium thiocyanate and Ruppert–Prakash reagent†
Received
6th November 2013
, Accepted 2nd January 2014
First published on 6th January 2014
Abstract
In the presence of copper thiocyanate, sodium thiocyanate and the inexpensive, easy-to-use trifluoromethylating reagent Me3Si–CF3, diazonium salts are smoothly converted into the corresponding aryl trifluoromethyl thioethers. Combined with diazotisation, this convenient and inexpensive method allows the straightforward synthesis of aryl or heteroaryl trifluoromethyl thioethers from the corresponding anilines.
Introduction
In recent years, methods for the introduction of fluorine-containing groups into organic molecules have attracted great attention within organic synthesis, as they can impart desirable properties to bioactive compounds.1 Substantial progress has recently been achieved in the field of late-stage trifluoromethylations,2 whereas the corresponding trifluoromethylthiolations are less developed.3 In general, trifluoromethylthio groups induce even higher lipophilicity than trifluoromethyl substituents (Hansch constant π = 1.44 versus 0.88),4 and are more bulky. This allows a more effective transport of drug molecules through lipid membranes, thereby increasing their bioavailability. Thus, SCF3 groups are often seen as key functionalities of many pharmaceutical and agrochemical products, such as tiflorex, toltrazuril (Baycox®) or vaniliprole.5
Traditional strategies
As shown in Scheme 1, several access routes exist for the formation of trifluoromethyl thioethers. Traditional strategies for the introduction of SCF3 groups include halogen–fluorine exchange reactions of trihalogenomethyl thioethers (A),6 as well as trifluoromethylations of sulfur-containing compounds such as thiols,7 thiocyanates8 and disulfides,9 all of which have to be synthesised in additional steps (B). More modern, one-step trifluoromethylthiolation methods can be divided into four main categories: electrophilic (C and D), nucleophilic (E) and radical (F), as well as oxidative cross-couplings (G). Examples of electrophilic processes are the reactions of trifluoromethanesulfonamides with aryl-magnesium or lithium reagents reported by Billard et al. (Scheme 1, C)10 and the copper-mediated reaction of arylboronic acids with hypervalent iodine–SCF3 reagents by Lu and Shen (D).11 Nucleophilic trifluoromethylthiolations include, for example, the palladium-catalysed trifluoromethylthiolation of aryl halides with sensitive AgSCF3 by Buchwald et al.,12 and the nickel-catalysed coupling of aryl halides either with the similarly unstable Me4NSCF3 by Zhang and Vicic,13 or with stable, but laborious-to-prepare copper–trifluoromethylthiolate complexes by Huang (E).14 C–H functionalizations are exemplified by the copper-mediated ortho-trifluoromethylthiolation of benzamides with CF3S–SCF3 by Daugulis et al. (F).15 Oxidative trifluoromethylthiolations have been reported by Qing et al.,16,17 who treated boronic acids with the Ruppert–Prakash reagent (TMSCF3) and sulfur in the presence of CuSCN and silver carbonate (G). Very recently, Zhang and Vicic developed a similar oxidative trifluoromethylthiolation using Me4NSCF3 as the source of SCF3.18
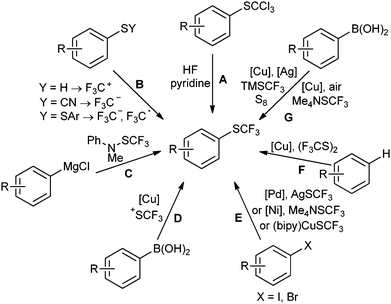 |
| Scheme 1 Strategies for the introduction of trifluoromethylthio groups. | |
Although the above approaches provide viable routes for the formation of trifluoromethyl thioethers, they all entail shortcomings, such as the laborious multi-step preparation of starting materials or the use of expensive, air-sensitive or poorly available reagents. As an alternative, we present a cheap and straightforward synthesis of trifluoromethyl thioethers via a Sandmeyer-type reaction.
Sandmeyer trifluoromethylthiolation
In the context of our work on new trifluoromethylation reactions,19,20 we have developed an effective synthesis of benzotrifluorides via a Sandmeyer reaction.21 The key advantage of this reaction over related processes22 is that the Cu–CF3 reagents are generated in situ from simple trifluoromethyl silanes or borates. An analogous reaction concept, in which easily accessible diazonium salts are converted into the corresponding trifluoromethyl thioethers via a redox-neutral reaction involving a nucleophilic CF3 reagent in combination with a sulfur source, appeared to be a plausible and attractive way of introducing trifluoromethylthio groups (Scheme 2, bottom). Clark et al. have shown the principal feasibility of Sandmeyer trifluoromethylthiolations starting from preformed CuSCF3. However, they found that their laboriously prepared CuSCF3 complex transfers its SCF3 group only reluctantly to diazonium salts, so that only a few electron-poor aryl trifluoromethyl thioethers could be accessed in reasonable yields.23
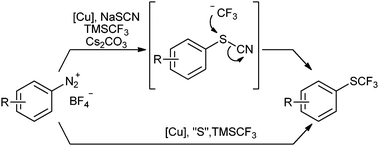 |
| Scheme 2 Approaches to Sandmeyer trifluoromethylthiolations. | |
Simply combining Clark's process with the in situ formation of the Cu–SCF3 reagents from a trifluoromethylating and a sulfurising agent thus did not appear to be a promising strategy towards a one-step trifluoromethylthiolation process. This assumption was supported by a series of test experiments (Table 1, entries 1–3).
Table 1 Optimisation of the reaction conditionsa
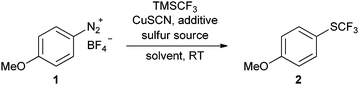
|
Entry |
Sulfur source |
Additive |
Solvent |
Yield of 2b [%] |
Reaction conditions: 0.5 mmol CuSCN, 2 equiv. additive, 1.5 equiv. sulfur source, 2 mL solvent, RT, dropwise addition of 0.5 mmol 1 in 2 mL of solvent, then 2 equiv. TMSCF3, 12 h.
Yields were determined by 19F NMR using 1,3-difluorobenzene as an internal standard.
TMSCF3 added before 1.
Without CuSCN.
1 equiv. Cs2CO3.
0.5 equiv. CuSCN.
0.1 equiv. CuSCN. Lawesson's reagent = 2,4-bis(4-methoxyphenyl)-1,3,2,4-dithiadiphosphetane-2,4-dithione.
|
1c |
S8 |
CsF |
MeCN |
5 |
2c |
Lawesson's reagent |
CsF |
MeCN |
Traces |
3c |
Na2S |
CsF |
MeCN |
0 |
4 |
NaSCN |
CsF |
MeCN |
30 |
5 |
KSCN |
CsF |
MeCN |
Traces |
6 |
NH4SCN |
CsF |
MeCN |
Traces |
7 |
NaSCN |
Cs2CO3 |
MeCN |
98 |
8 |
NaSCN |
Cs2CO3 |
DMF |
81 |
9 |
NaSCN |
Cs2CO3 |
Acetone |
18 |
10 |
NaSCN |
— |
MeCN |
0 |
11 |
— |
Cs2CO3 |
MeCN |
0 |
12d |
NaSCN |
Cs2CO3 |
MeCN |
0 |
13e |
NaSCN |
Cs2CO3 |
MeCN |
34 |
14f |
NaSCN |
Cs2CO3 |
MeCN |
98 |
15g |
NaSCN |
Cs2CO3 |
MeCN |
67 |
While searching for another straightforward strategy to introduce trifluoromethylthio groups, we reasoned that it should be advantageous to first connect the aryl-C–S and then the S–CF3 bonds. In search for a viable reaction pathway, we struck upon a report by Langlois et al. in which they demonstrated that aryl thiocyanates can be trifluoromethylated with Ruppert–Prakash's reagent.8 We reasoned that if we performed a Sandmeyer thiocyanation24 in the presence of a nucleophilic trifluoromethylation reagent, the arenediazonium salts might directly be converted into the corresponding aryl trifluoromethyl thioethers (Scheme 2, top). However, this appeared to be merely a theoretical possibility, since nucleophilic CF3 sources are known to react smoothly with copper salts.2e,19–21 Thus, one would expect that any trifluoromethylating reagent capable of substituting a cyano group in an aryl thiocyanate would also react with CuSCN intermediates to give unwanted Cu–CF3 or Cu–SCF3 species. Nevertheless, we were intrigued by the prospects offered by a one-pot trifluoromethylthiolation process and decided to evaluate its feasibility.
Results and discussion
Development of a Sandmeyer trifluoromethylthiolation
We systematically investigated the reaction of 4-methoxybenzenediazonium tetrafluoroborate with sodium thiocyanate and TMS–CF3 as a model system in the presence of various copper catalysts (see ESI†). As expected, anisole and 4-methoxybenzotrifluoride were formed in most cases, while the desired trifluoromethyl thioether 2 was only a minor product. However, when slowly adding the diazonium salt 1 and TMSCF3 to a mixture of sodium thiocyanate, CuSCN and CsF in acetonitrile, the desired trifluoromethyl thioether 2 was obtained in an encouraging 30% yield, along with a residual aryl thiocyanate intermediate (Table 1, entry 4). Under these conditions, the formation of 4-(trifluoromethyl)anisole was no longer observed.
Further experiments revealed that sodium thiocyanate is the most effective reagent, whereas many other thiocyanate salts suppressed the subsequent trifluoromethylation step (entries 5 and 6). A decisive step-up in the yields was achieved when replacing CsF, which is commonly used to activate TMSCF3, with Cs2CO3 (entry 7). Acetonitrile was confirmed to be the most effective solvent, which corresponds well with the findings for other Sandmeyer reactions (entries 8 and 9).25
Control experiments revealed that the reaction does not proceed if the copper mediator, the basic additive or sodium thiocyanate are omitted (entries 10–12). Reducing the amount of base to one equivalent led to decreased yields (entry 13). Even when the amount of copper was reduced to 50 mol%, the aryl trifluoromethyl thioether 2 was formed in a near-quantitative yield, with traces of the aryl thiocyanate as the only detectable by-product (entry 14). Reasonable yields were obtained even with only 10 mol% of copper (entry 15), which suggests that future catalyst generations will allow the metal loading to be lowered to truly catalytic amounts.
Scope of the new transformation
Having thus found an effective protocol for the trifluoromethylthiolation of arenediazonium salts, we next investigated its scope. As can be seen from the examples in Table 2, various arenediazonium tetrafluoroborates were smoothly converted into the corresponding aryl trifluoromethyl thioethers in moderate to excellent yields. In contrast to the reaction of diazonium salts with preformed CuSCF3, the new process is in no way limited to strongly electron-deficient derivatives.
Table 2 Scope of the trifluoromethylthiolation of arenediazonium saltsab
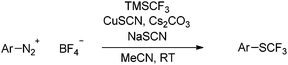
|
Reaction conditions: 1 mmol of arenediazonium tetrafluoroborate in 4 mL MeCN and 2 equiv. of TMSCF3 were slowly added to 0.5 equiv. CuSCN and 2 equiv. Cs2CO3 in 4 mL MeCN and stirred for 12 h at RT. Isolated yields are noted.
Yields determined by 19F NMR using 1,3-difluorobenzene as an internal standard.
|
|
Common functionalities including ester, ether, amino, keto, carboxylate and cyano groups were tolerated. Substrates containing chloro, bromo or even iodo substituents were trifluoromethylthiolated selectively at the position of the diazonium group. Various heterocycles such as quinoline, thiophene, benzothiazole and carbazole were also smoothly converted. Most products were directly obtained in a sufficiently pure form to allow their straightforward isolation. Only in a few cases did traces of the protodediazotisation products complicate the purification of the crude products. These results demonstrate the utility of the new reaction for the late-stage trifluoromethylthiolation of highly functionalised intermediates.
Mechanistic investigations
In order to gain a deeper mechanistic understanding, the reaction was investigated by 19F NMR. Mixtures of CuSCN, TMS–CF3 and Cs2CO3 in MeCN were found to contain CuCF3 (−28.1 ppm) and [Cu(CF3)2]− (−31.2 ppm), but no CuSCF3 species.26 This indicates that the SCN− anion does not react with TMS–CF3 under the reaction conditions. When NaSCN was added to the above mixture, the formation of CF3–copper species was no longer observed. This explains why no trifluoromethylation products are obtained under the optimised conditions. Further experiments confirmed that 4-methoxybenzenediazonium tetrafluoroborate (1) is smoothly converted into the aryl thiocyanate by treatment with CuSCN, NaSCN and Cs2CO3 in MeCN in the absence of a nucleophilic CF3 source. Moreover, preformed aryl thiocyanate quickly reacted to the corresponding aryl trifluoromethyl thioether 2 in the presence of a mixture of TMSCF3 and Cs2CO3, a process that does not require copper. Thus, a Sandmeyer trifluoromethylthiolation pathway involving CuSCF3 species was ruled out. Based on these results, we propose a mechanistic cycle, as depicted in Scheme 3.
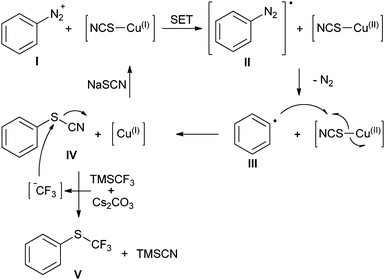 |
| Scheme 3 Proposed mechanism. | |
The diazonium salt is initially converted into the thiocyanate via a Sandmeyer process, in which the CuISCN species first transfers a single electron to the diazonium salt (I). The resulting diazo radical II releases nitrogen gas with the formation of an aryl radical III, which takes up the thiocyano group from the copper(II) intermediate to form the aryl thiocyanate IV. The presence of intermediate radicals was confirmed by the finding that the addition of TEMPO resulted in strongly decreased yields (20%). In the presence of Cs2CO3, the nucleophilic trifluoromethylation reagent TMSCF3 does not interfere with the above reaction steps, but efficiently converts the newly formed aryl thiocyanate to the trifluoromethyl thioether. This nucleophilic displacement of a cyanide leaving group by CF3 is promoted by Cs2CO3, probably by coordinating to the silicon atom in TMSCF3.
Conclusion
In conclusion, we have developed a straightforward, inexpensive and expedient method for the regiospecific conversion of arenediazonium salts into the corresponding aryl trifluoromethyl thioethers. The reaction is broadly applicable to electron-rich and electron-poor arene- and heteroarenediazonium salts and tolerates various functional groups. The availability of the substrates from the large pool of aromatic amines, the use of inexpensive reagents and the mild reaction conditions make this reaction particularly attractive for various applications from drug discovery to industrial-scale syntheses.5
Experimental section
The standard procedure for the synthesis of trifluoromethyl thioethers from the corresponding arenediazonium salts is as follows. Under a nitrogen atmosphere, an oven-dried 20 mL crimp cap vessel with a Teflon-coated stirrer bar was charged with copper thiocyanate (61.4 mg, 0.50 mmol), caesium carbonate (652 mg, 2.00 mmol) and sodium thiocyanate (122 mg, 1.50 mmol). Acetonitrile (4 mL) was added via syringe and the resulting suspension was stirred at room temperature for 10 minutes. A solution of the arenediazonium tetrafluoroborate (1.00 mmol) in acetonitrile (4 mL) was added dropwise via syringe and the reaction mixture was stirred for another 10 minutes. Trifluoromethyl-trimethylsilane (321 μL, 2.00 mmol) was then added via syringe and the mixture was stirred at ambient temperature for 16 h. The resulting mixture was filtered through a short pad of Celite (5 g) and rinsed with diethyl ether (20 mL). The resulting organic solution was washed with water (10 mL) and brine (10 mL). The organic layer was dried over MgSO4, filtered and concentrated (700 mbar, 40 °C). The residue was further purified by flash chromatography (SiO2, diethyl ether–hexane gradient), yielding the corresponding trifluoromethyl thioethers.
Acknowledgements
We thank the Landesgraduiertenförderung Rheinland Pfalz and Nanokat for financial support, and Umicore for donating metal catalysts.
Notes and references
-
(a)
T. Yamazaki, T. Taguchi and I. Ojima, Fluorine in Medicinal Chemistry and Chemical Biology, ed. I. Ojima, Wiley-Blackwell, Chichester, 2009 Search PubMed;
(b) P. Jeschke, ChemBioChem, 2004, 5, 570 CrossRef CAS PubMed;
(c) K. Muller, C. Faeh and F. Diederich, Science, 2007, 317, 1881 CrossRef PubMed;
(d) S. Purser, P. R. Moore, S. Swallow and V. Gouverneur, Chem. Soc. Rev., 2008, 37, 320 RSC;
(e) W. K. Hagmann, J. Med. Chem., 2008, 51, 4359 CrossRef CAS PubMed;
(f) T. Liang, C. N. Neumann and T. Ritter, Angew. Chem., Int. Ed., 2013, 52, 8214 CrossRef CAS PubMed;
(g) L. M. Yagupol'skii, A. Y. Il'chenko and N. V. Kondratenko, Russ. Chem. Rev., 1974, 43, 32 CrossRef PubMed.
- Reviews on trifluoromethylations:
(a) O. A. Tomashenko and V. V. Grushin, Chem. Rev., 2011, 111, 4475 CrossRef CAS PubMed;
(b) T. Furuya, A. S. Kamlet and T. Ritter, Nature, 2011, 473, 470 CrossRef CAS PubMed;
(c) X.-F. Wu, H. Neumann and M. Beller, Chem.–Asian J., 2012, 7, 1744 CrossRef CAS PubMed;
(d) Z. Jin, G. B. Hammond and B. Xu, Aldrichimica Acta, 2012, 45, 67 CAS; copper-mediated trifluoromethylations:
(e) T. Liu and Q. Shen, Eur. J. Org. Chem., 2012, 2012, 6679 CrossRef CAS; radical trifluoromethylations:
(f) A. Studer, Angew. Chem., Int. Ed., 2012, 51, 8950 CrossRef CAS PubMed; trifluoromethylations via C–H activation:
(g) H. Liu, Z. Gu and X. Jiang, Adv. Synth. Catal., 2013, 355, 617 CrossRef CAS.
- For reviews, see:
(a) V. N. Boiko, Beilstein J. Org. Chem., 2010, 6, 880 CrossRef PubMed;
(b) B. Manteau, S. Pazenok, J.-P. Vors and F. R. Leroux, J. Fluorine Chem., 2010, 131, 140 CrossRef CAS PubMed;
(c) A. Tlili and T. Billard, Angew. Chem., Int. Ed., 2013, 52, 6818 CrossRef CAS PubMed;
(d) F. Leroux, P. Jeschke and M. Schlosser, Chem. Rev., 2005, 105, 827 CrossRef CAS PubMed.
- C. Hansch, A. Leo, S. H. Unger, K. H. Kim, D. Nikaitani and E. J. Lien, J. Med. Chem., 1973, 16, 1207 CrossRef CAS.
-
(a) J. F. Giudicelli, C. Richer and A. Berdeaux, Br. J. Clin. Pharmacol., 1976, 3, 113 CrossRef CAS;
(b) A. Harder and A. Haberkorn, Parasitol. Res., 1989, 76, 8 CrossRef CAS;
(c) J. Stetter and F. Lieb, Angew. Chem., Int. Ed., 2000, 39, 1724 CrossRef.
-
(a) N. N. Yarovenko and A. S. Vasileva, J. Gen. Chem. USSR, 1958, 28, 2537 Search PubMed;
(b) E. A. Nodiff, S. Lipschutz, P. N. Craig and M. Gordon, J. Org. Chem., 1960, 25, 60 CrossRef CAS;
(c) A. E. Feiring, J. Org. Chem., 1979, 44, 2907 CrossRef CAS.
-
(a) C. Wakselman and M. Tordeux, J. Org. Chem., 1985, 50, 4047 CrossRef CAS;
(b) I. Kieltsch, P. Eisenberger and A. Togni, Angew. Chem., Int. Ed., 2007, 46, 754 CrossRef CAS PubMed;
(c) A. Harsányi, É. Dorkó, Á. Csapó, T. Bakó, C. Peltz and J. Rábai, J. Fluorine Chem., 2011, 132, 1241 CrossRef PubMed.
- T. Billard, S. Large and B. R. Langlois, Tetrahedron Lett., 1997, 38, 65 CrossRef CAS.
-
(a) C. Wakselman, M. Tordeux, J.-L. Clavel and B. Langlois, J. Chem. Soc., Chem. Commun., 1991, 993 RSC;
(b) B. Quiclet-Sire, R. N. Saicic and S. Z. Zard, Tetrahedron Lett., 1996, 37, 9057 CrossRef CAS;
(c) N. Roques, J. Fluorine Chem., 2001, 107, 311 CrossRef CAS;
(d) G. Blond, T. Billard and B. R. Langlois, Tetrahedron Lett., 2001, 42, 2473 CrossRef CAS;
(e) C. Pooput, M. Médebielle and W. R. Dolbier, Org. Lett., 2004, 6, 301 CrossRef CAS PubMed;
(f) C. Pooput, W. R. Dolbier and M. Médebielle, J. Org. Chem., 2006, 71, 3564 CrossRef CAS PubMed.
- F. Baert, J. Colomb and T. Billard, Angew. Chem., Int. Ed., 2012, 51, 10382 CrossRef CAS PubMed.
- X. Shao, X. Wang, T. Yang, L. Lu and Q. Shen, Angew. Chem., Int. Ed., 2013, 52, 3457 CrossRef CAS PubMed.
- G. Teverovskiy, D. S. Surry and S. L. Buchwald, Angew. Chem., Int. Ed., 2011, 50, 7312 CrossRef CAS PubMed.
- C.-P. Zhang and D. A. Vicic, J. Am. Chem. Soc., 2012, 134, 183 CrossRef CAS PubMed.
- Z. Weng, W. He, C. Chen, R. Lee, D. Tan, Z. Lai, D. Kong, Y. Yuan and K.-W. Huang, Angew. Chem., Int. Ed., 2013, 52, 1548 CrossRef CAS PubMed.
- L. D. Tran, I. Popov and O. Daugulis, J. Am. Chem. Soc., 2012, 134, 18237 CrossRef CAS PubMed.
- C. Chen, Y. Xie, L. Chu, R.-W. Wang, X. Zhang and F.-L. Qing, Angew. Chem., Int. Ed., 2012, 51, 2492 CrossRef CAS PubMed.
- C. Chen, L. Chu and F.-L. Qing, J. Am. Chem. Soc., 2012, 134, 12454 CrossRef CAS PubMed.
- C.-P. Zhang and D. A. Vicic, Chem.–Asian J., 2012, 7, 1756 CrossRef CAS PubMed.
- T. Knauber, F. Arikan, G.-V. Röschenthaler and L. J. Gooßen, Chem.–Eur. J., 2011, 17, 2689 CrossRef CAS PubMed.
- B. A. Khan, A. E. Buba and L. J. Gooßen, Chem.–Eur. J., 2012, 18, 1577 CrossRef CAS PubMed.
- G. Danoun, B. Bayarmagnai, M. F. Grünberg and L. J. Gooßen, Angew. Chem., Int. Ed., 2013, 52, 7972 CrossRef CAS PubMed.
-
(a) J.-J. Dai, C. Fang, B. Xiao, J. Yi, J. Xu, Z.-J. Liu, X. Liu, L. Liu and Y. Fu, J. Am. Chem. Soc., 2013, 135, 8436 CrossRef CAS PubMed;
(b) X. Wang, Y. Xu, F. Mo, G. Ji, D. Qiu, J. Feng, Y. Ye, S. Zhang, Y. Zhang and J. Wang, J. Am. Chem. Soc., 2013, 135, 10330 CrossRef CAS PubMed.
- D. J. Adams, A. Goddard, J. H. Clark and D. J. Macquarrie, Chem. Commun., 2000, 987 RSC.
-
(a) I. P. Beletskaya, A. S. Sigeev, A. S. Peregudov and P. V. Petrovskii, Mendeleev Commun., 2006, 16, 250 CrossRef PubMed;
(b) M. Barbero, I. Degani, N. Duilgheroff, S. Dughera and R. Fochi, Synthesis, 2001, 4, 585 CrossRef PubMed.
- A. Roglans, A. Pla-Quintana and M. Moreno-Mañas, Chem. Rev., 2006, 106, 4622 CrossRef CAS PubMed.
-
(a) H. Morimoto, T. Tsubogo, N. D. Litvinas and J. F. Hartwig, Angew. Chem., Int. Ed., 2011, 50, 3793 CrossRef CAS PubMed;
(b) O. A. Tomashenko, E. C. Escudero-Adán, M. Martínez Belmonte and V. V. Grushin, Angew. Chem., Int. Ed., 2011, 50, 7655 CrossRef CAS PubMed;
(c) J. H. Clark, C. W. Jones, A. P. Kybett, M. A. McClinton, J. M. Miller, D. Bishop and R. J. Blade, J. Fluorine Chem., 1990, 48, 249 CrossRef CAS.
Footnote |
† Electronic supplementary information (ESI) available: procedural and spectral data. See DOI: 10.1039/c3sc53076k |
|
This journal is © The Royal Society of Chemistry 2014 |
Click here to see how this site uses Cookies. View our privacy policy here.