DOI:
10.1039/C4RA12061B
(Paper)
RSC Adv., 2014,
4, 60652-60657
Synthesis of β-hydroxy-1,4-disubstituted-1,2,3-triazoles catalyzed by copper ferrite nanoparticles in tap water using click chemistry†
Received
9th October 2014
, Accepted 6th November 2014
First published on 7th November 2014
Abstract
A novel one pot synthesis of β-hydroxy-1,4-disubstituted-1,2,3-triazoles has been developed by using CuFe2O4 magnetic nanoparticles. This methodology involves additive free, easily recyclable catalyst in water medium and avoids the handling of organic azides as they are generated in situ.
Introduction
Although homogeneous catalysts have several advantages like higher selectivity and strong interaction with the components in the reaction, the separation of the final product is difficult after completion of the reaction and trace amounts of catalyst may remain in the final product. Since metal contamination is highly regulated in the pharmaceutical industry, it is essential to remove the catalyst from the final compound even if it is at the ppm level. However, heterogeneous catalysts, in particular nano catalysts due to their particle size, having a very large surface area help to enhance the contact between reactants and catalyst, which can maximize the reaction rates and minimize the consumption of the catalyst to give excellent yields.1 However, the isolation of these nanoparticles is not easy and involves filtration or centrifugation steps. It is beneficial for these catalysts to be separated from the reaction mixture with the aid of an external magnet. In this context, magnetic nanoparticles (MNPs) have emerged as a useful group of heterogeneous catalysts, because of their insoluble and paramagnetic nature.2
1,2,3-Triazoles are important in heterocyclic chemistry because of their unique structure and chemical properties. Many compounds possessing 1,2,3-triazole structural scaffold exhibit interesting biological properties such as antibacterial, antiviral, antiepileptic, and anti-allergic activities.3,4 In particular, 1,4-disubstituted-1,2,3-triazoles can be used to selectively open calcium channels in cells,5 to regulate plant growth,6 and to inhibit enzymes,7 as well as exhibit significant antiproliferative action against a wide range of human cancer cell lines.8
Sharpless9 re-invigorated the Huisgen's 1,3-dipolar cycloaddition10 by using a Cu(I) metal source. These copper-accelerated 1,3-dipolar cycloadditions enabled efficient preparation of 1,4-disubstituted-1,2,3-triazoles from alkynes and azides with excellent selectivity. Recently a few procedures have been reported for the synthesis of β-hydroxy triazoles by the opening of epoxides in presence of homogeneous catalysts like CuSO4 (ref. 11) or CuI.12 Francisco Alonso13 and Patrick Pale14 have developed separately laboratory-made copper nano particles on activated carbon and Cu(I)-zeolite catalytic method to afford 1,4-disubstituted-β-hydroxy triazoles. Since the resulting products possess a 1-(hydroxyethyl)-1H-1,2,3-triazole moiety which is present in peptide surrogates of HIV-1 protease inhibitors AB2, AB3 (Fig. 1) and also useful in drugs and pharmaceuticals,15 there is a need to develop a simple and efficient method by using a readily available and reusable heterogeneous catalyst in green solvent.
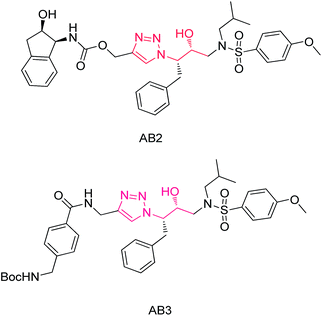 |
| Fig. 1 AB2 (pdb-1zp8) and AB3 (pdb-1zpA). | |
In continuation of our research interest in the synthesis of heterocyclic compounds using green synthetic protocols involving transition metal catalyst,16 a copper ferrite catalyzed one pot synthesis of 1,4-disubstituted-1,2,3-triazoles from benzyl halides, sodium azide, and alkynes17 is developed. Furthermore, in this context, we hereby report copper ferrite MNPs (purchased from Aldrich-CAS no. 12018-79-0) catalyzed one-pot synthesis of β-hydroxy triazoles from epoxides, sodium azide, and alkynes in aqueous medium. The present protocol satisfies most of the green chemistry principles such as (a) one pot multi component synthesis, (atom economy) (b) reactions in water medium, (green solvent) (c) use of easily separable and recyclable heterogeneous catalyst with the help of an external magnet, (d) simple workup procedure.
Results and discussion
Initially, CuFe2O4 catalyzed reaction between styrene oxide 1a (1 mmol) and phenyl acetylene 2a (1 mmol) was investigated to optimise the reaction conditions in various solvents (Scheme 1). Among different solvents examined (Table 1), water yielded the best results (entry 2), where as MeOH and MeOH
:
H2O (1
:
1) gave the products in moderate yields (entries 1 and 7). Solvents such as DMSO, DMF, THF and toluene were ineffective (entries 3, 4, 6 and 8). The influence of the amount of catalyst and sodium azide was also evaluated. It was observed that the best yield was obtained using 5 mol% of catalyst and 1.1 mmol of NaN3 (entries 9–13).
 |
| Scheme 1 | |
Table 1 Optimization studies for the synthesis of β-hydroxy-1,4-disubstituted-1,2,3-triazolesa
Entry |
Catalyst (mol%) |
NaN3 (mmol) |
Solvent |
Yieldb (%) |
Reaction conditions styrene oxide (1 mmol), phenyl acetylene (1 mmol), NaN3 (1.0–1.5 mmol), CuFe2O4 (1–10 mol%) in H2O (10 mL) at 60 °C for 6 h. Isolated yields. At 40 °C. at 80 °C. |
1 |
5 |
1.1 |
MeOH |
45 |
2 |
5 |
1.1 |
H2O |
87 |
3 |
5 |
1.1 |
DMSO |
0 |
4 |
5 |
1.1 |
DMF |
Trace |
5 |
5 |
1.1 |
EtOH |
30 |
6 |
5 |
1.1 |
THF |
Trace |
7 |
5 |
1.1 |
MeOH : H2O (1 : 1) |
68 |
8 |
5 |
1.1 |
Toluene |
0 |
9 |
1 |
1.1 |
H2O |
42 |
10 |
2 |
1.1 |
H2O |
49 |
11 |
10 |
1.1 |
H2O |
87 |
12 |
5 |
1.0 |
H2O |
71 |
13 |
5 |
1.5 |
H2O |
87 |
14 |
5 |
1.1 |
— |
0 |
15c |
5 |
1.1 |
H2O |
69 |
16d |
5 |
1.1 |
H2O |
86 |
With the optimal conditions in hand, styrene oxide (1a) was treated with sodium azide and phenyl acetylene (2a) in the presence of 5 mol% of CuFe2O4 in 10 mL of water at 60 °C (Scheme 2). The reaction proceeded smoothly to give the corresponding β-hydroxy-1,4-disubstituted-1,2,3-triazole (3aa) in high yield. Based on the literature reports,12,13 among the two possible regional isomers, it was observed that the present product was exclusively primary β-hydroxy triazole (3aa) which was obtained from the styrene oxide by benzylic position opening.
 |
| Scheme 2 | |
The scope of this copper ferrite catalyzed click reaction was further expanded with a variety of oxiranes and alkynes and these results were summarized in Table 2. The reactions proceeded well to obtain products in encouraging yields. Electron donating substituents like tertiary butyl, as well as methyl group at para position (entries 3 and 4) and electron withdrawing fluorine atom at meta position of phenyl acetylene (entry 5) did not induce appreciable changes in the reaction efficiency. The presence of heterocyclic moiety in the alkynes was equally effective towards the opening of epoxide, followed by 1,3-dipolar cycloaddition (entry 2).
Table 2 One pot synthesis of β-hydroxy triazoles using CuFe2O4 nano particles in water from various epoxides, sodium azide and alkynesa
Entry |
Epoxide 1 |
Alkyne 2 |
Triazole 3 |
t (h) |
Yieldb (%) |
Reaction conditions: alkyne (1 mmol), epoxide (1 mmol), NaN3 (1.1 mmol), CuFe2O4 (5 mol%) in H2O (10 mL) at 60 °C. Isolated yield. Phenyl acetylene (2 mmol). |
1 |
 |
 |
 |
6 |
87 |
2 |
1a |
 |
 |
6 |
84 |
3 |
1a |
 |
 |
8 |
80 |
4 |
1a |
 |
 |
7 |
77 |
5 |
1a |
 |
 |
6 |
79 |
6 |
1a |
 |
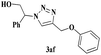 |
7 |
85 |
7 |
 |
 |
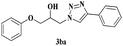 |
5 |
87 |
8 |
1b |
 |
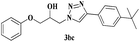 |
6 |
81 |
9 |
1b |
 |
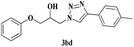 |
6 |
84 |
10 |
1b |
 |
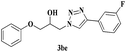 |
7 |
78 |
11 |
1b |
 |
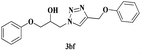 |
6 |
82 |
12 |
1b |
 |
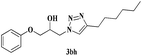 |
4 |
76 |
13 |
 |
 |
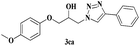 |
6 |
84 |
14 |
1c |
 |
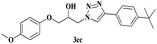 |
7 |
79 |
15 |
1c |
 |
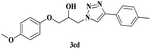 |
7 |
83 |
16 |
1c |
 |
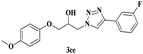 |
8 |
80 |
17 |
1c |
 |
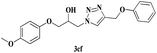 |
6 |
82 |
18 |
1c |
 |
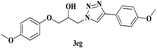 |
8 |
79 |
19 |
 |
 |
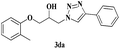 |
6 |
86 |
20 |
1d |
 |
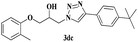 |
7 |
83 |
21 |
1d |
 |
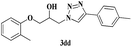 |
7 |
85 |
22 |
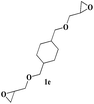 |
 |
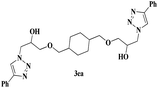 |
9 |
74c |
The reactivity of glycidyl phenyl ether (entries 7 to 12) and aryl substituted glycidyl phenyl ethers was further explored and examined (Scheme 3). These epoxides gave a single regioisomer (3ba) with preferential attack at the less hindered terminal carbon atom which was confirmed by the reported literature.12,13
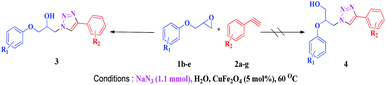 |
| Scheme 3 | |
Both para methoxy as well as ortho methyl substituted glycidyl phenyl ethers also reacted effectively (entries 13 to 22). The reaction between glycidyl phenyl ether and aliphatic 1-octyne (entry 12) resulted in the product in an encouraging yield. It was observed that 1,4-bis oxirane also reacted with two equivalents of phenyl acetylene providing bis triazole product (entry 22).
Recycling
CuFe2O4 catalyst was easily separated by using an external magnet from the reaction mixture (Fig. 2), washed with acetone and dried under vacuum. The air dried catalyst was used directly for the next cycles without further purification. As shown in Table 3, catalyst could be reused four times without significant changes.
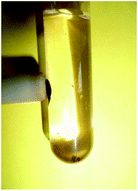 |
| Fig. 2 Separation of CuFe2O4 nano particles by using an external magnet. | |
Table 3 Recyclability of nano CuFe2O4 catalysta
Cycle |
Isolated product yield (%) |
Catalyst recovery (%) |
Reaction conditions: phenyl acetylene (1 mmol), styrene oxide (1 mmol), NaN3 (1.1 mmol), CuFe2O4 (5 mol%) in H2O (10 mL) at 60 °C. |
Native |
87 |
91 |
1 |
85 |
89 |
2 |
82 |
86 |
3 |
80 |
81 |
The SEM images of the catalyst exhibited identical shape and size (Fig. 3) and the XRD analysis also indicated similar peaks for both native and recycled catalyst (Fig. 4). These results clearly support nearly unaltered efficiency of the catalyst.
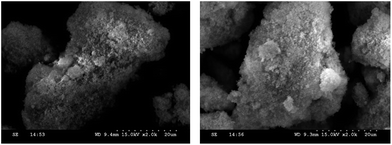 |
| Fig. 3 (a) SEM-analysis of native CuFe2O4 catalyst. (b) SEM-analysis of reused CuFe2O4 catalyst after 4th cycle. | |
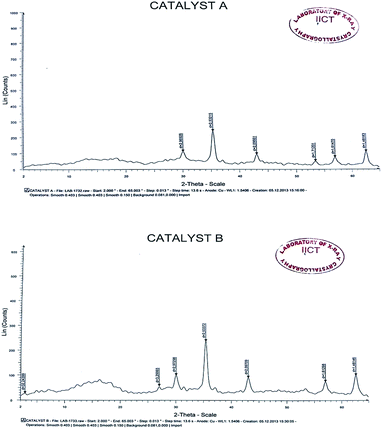 |
| Fig. 4 XRD spectra of CuFe2O4 catalyst (a) native and (b) after the 4th cycle. | |
Conclusions
In conclusion, an efficient green protocol is developed for the synthesis of β-hydroxy-1,2,3-triazoles from epoxides, sodium azide and alkynes in aqueous medium. The advantages of the present protocol are (a) one pot three component 1,3-dipolar alkyne-azide cyclo addition, which involves the in situ generation of organic azides by using low cost sodium azide in water medium as it avoids the isolation of hazardous organic azides, (b) reduction in the number of steps and waste generation, which improves the overall yield and reduces the reaction time and operational procedures, (c) ease of recyclability of CuFe2O4 magnetic nano particles, (d) use of universally acceptable green solvent–water medium without any additives at 60 °C. This method is simple, facile and applicable to a wide range of epoxides and alkynes with high functional group tolerance.
Experimental section
General experimental procedure
Styrene oxide (1 mmol), phenyl acetylene (1 mmol) and NaN3 (72 mg, 1.1 mmol) were placed in a 25 mL round-bottomed flask in H2O (10 mL), to which CuFe2O4 (5 mol%) was added. The reaction mixture was warmed to 60 °C and monitored by TLC until total conversion of the starting materials. After completion of the reaction, the catalyst was separated with the aid of a magnet. The separated catalyst was washed several times with acetone, dried under vacuum. The reaction mixture was extracted with EtOAc (4 × 10 mL), the collected organic phases were dried with Na2SO4 and the solvent was removed under vacuum to give the corresponding triazole derivative. Further, products were purified by column chromatography using hexane and ethyl acetate.
Acknowledgements
The authors thank the Council of Scientific and Industrial Research (CSIR), New Delhi for financial assistance.
References
- P. T. Anastas and J. C. Warner, Green Chemistry Theory and Practice, Oxford University Press, Oxford, 1998 Search PubMed.
-
(a) G. A. Somorjai, H. Frei and J. Y. Park, J. Am. Chem. Soc., 2009, 131, 16589l CrossRef PubMed;
(b) A. H. Lu, E. L. Salabas and F. Schuth, Angew. Chem., Int. Ed., 2007, 46, 1222 CrossRef CAS PubMed;
(c) V. Polshettiwar and R. S. Varma, Green Chem., 2010, 12, 743 RSC;
(d) Md. A. Zolfigol, V. Khakyzadeh, A. R. Moosavi-Zare, A. Rostami, A. Zare, N. Iranpoor, Md. H. Beyzavi and R. Luque, Green Chem., 2013, 15, 2132 RSC;
(e) Md. A. Zolfigol, V. Khakyzadeh, A. R. Moosavi-Zare, A. Zare, P. Arghavani-Hadi, Z. Mohammadi and Md. H. Beyzavi, S. Afr. J. Chem., 2012, 65, 280 CAS;
(f) Md. A. Zolfigol, A. R. Moosavi-Zare, P. Moosavi, V. Khakyzadeh and A. Zare, C. R. Chim., 2013, 16, 962 CrossRef CAS PubMed;
(g) A. Khazaei, A. R. Moosavi-Zare, H. Afshar-Hezarkhani and V. Khakyzadeh, RSC Adv., 2014, 4, 32142 RSC.
-
(a) H. Wamhoff, in Comprehensive Heterocyclic Chemistry, ed. A. R. Katritzky and C. W. Rees, Pergamon, Oxford, 1984, vol. 5, p. 669 Search PubMed;
(b) S. Velazquez, R. Alvarez, C. Perez, F. Gago, C. De, J. Balzarini and M. Camaraza, Antiviral Chem. Chemother., 1998, 9, 481 CAS;
(c) C. Im, S. N. Maiti, R. G. Micetich, M. Daneshtalab, K. Atchison and O. A. Phillips, J. Antibiot., 1994, 47, 1030 CrossRef CAS.
-
(a) M. J. Genin, D. A. Allwine, D. J. Anderson, M. R. Barbachyn, D. E. Emmert, S. A. Garmon, D. R. Graber, K. C. Grega, J. B. Hester, D. K. Hutchinson, J. Morris, R. J. Reischer, C. W. Ford, G. E. Zurenko, J. C. Hamel, R. D. Schaadt, D. Stapert and B. H. Yagi, J. Med. Chem., 2000, 43, 953 CrossRef CAS PubMed;
(b) W. Q. Fan and A. R. Katritzky, in Comprehensive Heterocyclic Chemistry II, ed. A. R. Katritzky, C. W. Rees and E. F. V. Scriven, Elsevier Science, Oxford, 1996, vol. 4, pp. 1–126 Search PubMed.
- V. Calderone, I. Giorgi, O. Livi, E. Martinotti, E. Mantuano, A. Martelli and A. Nardi, Eur. J. Med. Chem., 2005, 40, 521 CrossRef CAS PubMed.
- J. M. Beck, R. K. Mann and E. Lilly, US Pat. 4931083, June 1990.
- U. Nilsson, PCT Int. Appl., WO 2009139719 A1 20091119, 2009.
-
(a) S. A. Bakunov, S. M. Bakunova, T. Wenzler, M. Ghebru, K. A. Werbovetz, R. Brun and R. R. Tidwell, J. Med. Chem., 2010, 53, 254 CrossRef CAS PubMed;
(b) A. H. Banday, S. A. Shameem, B. D. Gupta and H. M. Sampath Kumar, Steroids, 2010, 801 CrossRef CAS PubMed.
- H. V. Rostovtsev, L. G. Green, V. V. Fokin and K. B. Sharpless, Angew. Chem., Int. Ed. Engl., 2002, 41, 2596 CrossRef.
-
(a) R. Huisgen, Angew. Chem., Int. Ed. Engl., 1963, 2, 565 CrossRef;
(b) 1,3-Dipolar Cycloaddition Chemistry, ed. A. Padwa, Wiley, New York, 1984, pp. 1–176 Search PubMed;
(c) Comprehensive Organic Synthesis, ed. B. M. Trost, Pergamon, Oxford, 1991, vol. 4, pp. 1069–1109 Search PubMed.
- L. S. Campbell-Verduyn, W. Szymanski, C. P. Postema, R. A. Dierckx, P. H. Elsinga, D. B. Janssen and B. L. Feringa, Chem. Commun., 2010, 46, 898 RSC.
- G. Kumaraswamy, K. Ankamma and A. Pitchaiah, J. Org. Chem., 2007, 72, 9822 CrossRef CAS PubMed.
- F. Alonso, Y. Moglie, G. Radivoy and M. Yus, J. Org. Chem., 2011, 76, 8394 CrossRef CAS PubMed.
- T. Boningari, A. Olmos, B. M. Reddy, J. Sommer and P. Pale, Eur. J. Org. Chem., 2010, 6338 CrossRef CAS.
- A. Brik, J. Alexandratos, Y.-C. Lin, J. H. Elder, A. J. Olson, A. Wlodawer, D. S. Goodsell and C.-H. Wong, ChemBioChem, 2005, 6, 1167 CrossRef CAS PubMed.
-
(a) K. Harsha Vardhan Reddy, V. Prakash Reddy, B. S. P. Anil Kumar, R. Uday Kumar and Y. V. D. Nageswar, RSC Adv., 2014, 4, 45579 RSC;
(b) K. Swapna, S. N. Murthy, M. T. Jyothi and Y. V. D. Nageswar, Org. Biomol. Chem., 2011, 9, 5989 RSC;
(c) K. H. V. Reddy, V. P. Reddy, J. Shankar, B. Madhav, B. S. P. A. Kumar and Y. V. D. Nageswar, Tetrahedron Lett., 2011, 52, 2679 CrossRef CAS PubMed;
(d) K. H. V. Reddy, V. P. Reddy, J. Shankar and Y. V. D. Nageswar, Synlett, 2011, 9, 1268 Search PubMed;
(e) K. H. V. Reddy, A. A. Kumar, G. Kranthi and Y. V. D. Nageswar, Beilstein J. Org. Chem., 2011, 7, 886 CrossRef CAS PubMed;
(f) G. Satish, K. H. V. Reddy, K. Ramesh and K. Karnakar, Tetrahedron Lett., 2012, 53, 2518 CrossRef CAS PubMed;
(g) K. H. V. Reddy, G. Satish, K. Ramesh, K. Karnakar and Y. V. D. Nageswar, Chem. Lett., 2012, 41, 585 CrossRef CAS;
(h) B. Madhav, S. Narayana Murthy, B. S. P. Anil Kumar, K. Ramesh and Y. V. D. Nageswar, Tetrahedron Lett., 2012, 53, 3835 CrossRef CAS PubMed;
(i) B. S. P. A. Kumar, B. Madhav, K. H. V. Reddy and Y. V. D. Nageswar, Tetrahedron Lett., 2011, 52, 2862 CrossRef CAS PubMed;
(j) K. Ramesh, K. Karnakar, G. Satish, B. S. P. Anil Kumar and Y. V. D. Nageswar, Tetrahedron Lett., 2012, 53, 6936 CrossRef CAS PubMed;
(k) K. Harsha Vardhan Reddy, G. Satish, V. Prakash Reddy, B. S. P. Anil Kumar and Y. V. D. Nageswar, RSC Adv., 2012, 2, 11084 RSC;
(l) G. Satish, K. H. V. Reddy, B. S. P. Anil kumar, J. Shankar, R. Uday kumar and Y. V. D. Nageswar, Tetrahedron Lett., 2014, 55, 5533 CrossRef CAS PubMed.
- B. S. P. Anil Kumar, K. Harsha Vardhan Reddy, B. Madhav, K. Ramesh and Y. V. D. Nageswar, Tetrahedron Lett., 2012, 53, 4595 CrossRef CAS PubMed.
Footnote |
† Electronic supplementary information (ESI) available. See DOI: 10.1039/c4ra12061b |
|
This journal is © The Royal Society of Chemistry 2014 |
Click here to see how this site uses Cookies. View our privacy policy here.