DOI:
10.1039/C4RA09640A
(Paper)
RSC Adv., 2014,
4, 47056-47065
pH- and electro-response characteristics of bacterial cellulose nanofiber/sodium alginate hybrid hydrogels for dual controlled drug delivery
Received
2nd September 2014
, Accepted 16th September 2014
First published on 16th September 2014
Abstract
In this work, hybrid hydrogels composed of bacterial cellulose nanofibers (nf-BC) and sodium alginate (SA) were prepared as a dual-stimuli responsive release system. The pH and electric field stimulus-responsive swelling properties and the stimulus-responsive drug release behaviors of the nf-BC/SA hybrid hydrogels using Ibuprofen as a model drug, were investigated in vitro. As the pH changed from 1.5 to 11.8, the swelling ratio increased from less than 8 times compared with its dry weight at acidic conditions to more than 13 times when the pH value was 11.8. When the electric field changed from 0 to 0.5 V, the hybrid hydrogels also showed an increasing swelling ratio from 8 times to 14 times their dry weight. The release of Ibuprofen (IBU) could be controlled by the action of deprotonation or protonation of calcium alginate in the hydrogels under different pH conditions, faster in alkaline conditions and slower in acidic conditions. Furthermore, the drug release from the hybrid hydrogels could be enhanced with an applied electric stimulus. The drug release mechanism under pH and applied electric field could be interpreted by the superposition of both Fickian diffusion and case-II transport based on Peppas' semi-empirical equation. The nf-BC/SA hybrid hydrogels with dual pH- and electro-response were new promising candidates as controlled drug delivery systems.
1. Introduction
A drug delivery system (DDS) is a program of releasing a drug at a given release rate, at suitable time intervals or in a specific amount of drug as needed by the treatment.1–3 A drug delivery system with response-induced features represents several potential advantages. For example, the drug release controlled by a real-time stimulus-responsive, with on-demand dosage capability has achieved more and more interests with the ongoing enhancements. Therefore, the drug delivery systems have been studied with particular emphasis on their reversible volume changes by applying an external stimulus including electrical field, magnetic field, ultrasonic waves, light, pressure, pH, upon which the drug releases with a pre-designed pattern from the system to the diseased host to minimize life-threatening occurrences.4 The “intelligent” or “smart” hydrogels can exert obvious changes in swelling behavior, network structure, even in mechanical strength as responses to different applied stimuli which are being developed as responsive drug delivery systems. These drug carriers can release drug with a required quantity not only in a proper time but also in appropriate place in the body with the application of stimulus.5–8
Two important systems among them, from an applied point of view, are that sensitive to pH and electrical field. Because of variations in physiological pH in vitro such as gastrointestinal tract or pathological areas, pH-sensitive drug delivery system has a tremendous potential in the application for modulating drug release.9–12 On the other hand, hydrogels sensitive to electric field are usually prepared from pH-sensitive hydrogels, such as polyelectrolytes. Under the stimulus of an electric field, electro-responsive hydrogels may particularly offer unique advantages for providing on-demand release of drug molecules from implants or transdermal drug delivery systems.13–19
Alginate extracted from brown algae is an anionic polysaccharide. It exists in numerous applications such as wound healing, drug delivery, and tissue engineering owing to its favorable properties, including biocompatibility, biodegradability, non-toxicity, transparency, and ease of gelation.20–22 As the hydrogel possesses large number of ionizable –COO groups, alginate is prepared as components of stimuli-responsive delivery system.23,24 The pH-responsive SA and its derivative network has been exploited to prepare enteric dosage forms using the network as coating or as a matrix, showed slow release in low pH condition due to limited swelling of the particles and drug diffusion, but rapid release at neutral condition due to erosion of the network and enhanced diffusion rate. However, the low mechanical performances of SA hydrogels and relatively short effective drug release time limit its practical applications. It is also reported that pure SA hydrogels is nearly absent in electrical responsiveness under electric field.25 Therefore, an interpenetrating polymer network (IPN) of alginate was developed to overcome the main disadvantage of the relatively low mechanical strength and enhance the sensitivity of intelligent hydrogels.26–28
Another biopolymer of great potential materials was bacterial cellulose (BC) which was synthesized in abundance by Acetobacter xylinum, showed many unique characters including an ultrafine nanofibrous network structure, bioadaptibility, chemical stability, and non-toxicity.29–32 BC has been attracting attention as a raw material for preparation of advanced materials due to its advantageous properties that are imparted by the fine network structure.33–36 These advantageous rendered BC an attractive material for use in devising intelligent drug-delivery systems with efficient in vivo performance.37,38 A novel porous sponge which gained the beneficial properties of both BC and alginate was developed for the use in oral tissue regeneration.39 Recently, we have reported the synthesis and characterization of ionic crosslinking sodium alginate (SA)/carboxymethylated bacterial cellulose (CM-BC) composites with a semi-IPN structure and discussed its biomedical application. The swelling and mechanical properties of the composite were dramatically improved, at the same time, the pH-sensitivity was well maintained.40
In this study, we focused on further exploring the possibilities of developing nf-BC/SA hybrid hydrogels as an dual intelligent responsive drug release system. Ibuprofen is a kind of non-steroidal drugs which is used in musculoskeletal and joint disorders but causes a certain irritation in the gastrointestinal mucosa and possesses a bitter taste. The short half-life (2–3 h) and associated gastric side effects make Ibuprofen a good candidate for colonic targeting and transdermal delivery.41 Therefore, Ibuprofen was used in this investigation as the model drug. We hope that the introduction of BC nanofibers into the polymer matrix with semi-IPN structure may enhance some properties of SA hydrogels, such as prolonging the drug release time, improving the poor mechanical performances and electric response sensitivity. Thermal and microstructure of the nf-BC/SA hydrogels were performed to characterized the structure, stability and the porosity of the hydrogels. The swelling ratios of the nf-BC/SA hybrid hydrogels were studied at different pH conditions and electric field respectively. Furthermore, in vitro release behaviors were investigated at different pH conditions and electric field. Both swelling ratios and in vitro drug release behaviors of the drug-loaded hydrogels were studied to evaluate the electro- and pH-responsiveness of the hydrogels and their overall potential as a drug-delivery system.
2. Materials and methods
2.1 Material
Bacterial cellulose, white gel-like pellicles with thickness of 0.5 cm, were purchased from Yida Food Industry Co., Ltd. The sodium alginate was purchased from Tianjin Guangfu fine chemical research institute. Ibuprofen (IBU) was supplied by Juhua Group Co., Ltd. The rest of regents used in the experiments were all analytical reagents.
2.2 Preparation of nf-BC/SA hydrogels
To prepare the nf-BC/SA nanohydrogels, the preparation procedure is separated into two stages. First, the gel-like cellulose pellicles were immersed in 0.1 mol L−1 sodium hydroxide solution for 60 min at 90 °C water bath to remove the bacterial cell debris, then thoroughly washed by de-ionized water until the value of pH reach 7.0 and stored them in distilled water at 4 °C before used. The BC pellicles were crushed to form BC slurry at room temperature by homogenizer. Second sodium alginate was dissolved in distilled water. Sodium alginate of 2.0% (w/v) was dissolved in distilled water at room temperature to form a gel-like solution. To prepare nf-BC/SA hybrid hydrogels with varying contents of BC, the BC slurry and sodium alginate solution, at the weight ratios of BC/alginate at 100/0, 80/20, 67/33, 50/50, and 0/100, were mixed on a magnetic stirrer to obtain homogenous nf-BC/SA dispersions. The hybrid hydrogels were named as nf-BC/SA20, nf-BC/SA33, and nf-BC/SA50, on the basis of SA ratio in the hydrogels. Next, the mixtures were placed in Petri dish, cross-linked by an aqueous solution of 1.5 (w/v) CaCl2 and rinsed by de-ionized water to remove the excess cross-linking agents. Third the hybrid hydrogels were prefrozen at −40 °C for 12 h prior to experience a freezing-dry process for 24 h.
2.3 Characterization of nf-BC/SA hybrid hydrogels
XRD, FT-IR and mechanical testing data of the prepared hydrogels were determined by Chiaoprakobkij.39 The morphologies of the structure of the nf-BC/SA hydrogels were examined by scanning electron microscopy (SEM; Apollo 300, 10 kV). The samples were freeze-dried and coated with gold in sputter coater under nitrogen atmosphere.
Shimadzu TGA 50 analyzer was used to perform thermogravimetric analysis (TGA) and differential scanning calorimetry of the BC, AA, and hydrogels. In preparation for TGA, the hydrogels were ground to powder form. BC, SA, and hydrogel powder was placed in the sample pan for analysis over a temperature range of 50–650 °C at a heating rate of 10 °C min−1 under a nitrogen purge.
2.4 Responsive properties in swelling behavior
The swelling behaviors of the hydrogels which analysed in the condition of different pH and electric voltages applied were determined through a gravimetric method. Briefly, hydrogels were weighed (Wo) first. The swelling behaviors of dry samples were investigated by their swelling ratios (Sw) in PBS solutions with pH 1.5–12.0 in 37 °C. In addition the dried samples were immersed into the PBS (pH 7.4) for a certain period under different voltage of 0–0.5 V which was reported as an acceptable application range producing minimal damage and irritation.42 At a predetermined time point, the swollen samples were taken out to remove excess of buffer solution on the surface by wiping with tissue paper. They were weighed (Wt) immediately. After that, the samples were returned to the containers with refreshed buffer solution. All testing was proceed in triplicate; the equilibrium swelling ratio (Sw) of the hydrogels was calculated as follows:10 |
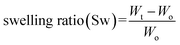 | (1) |
2.5 Responsive release behaviours in vitro
Ibuprofen was selected as a model drug for release study. 1% (w/v) Ibuprofen was added to the nf-BC/SA suspensions (prepared for forming hydrogels in the second stage). Drug loaded hydrogel samples were fabricated in a template (15 mm in diameter) by drug loaded nf-BC/SA suspensions as described before and dried by freeze-drying for 24 h.
2.5.1 Drug release under different pH conditions. The drug loaded hydrogels were added into 100 mL solution and vibrated at 37 °C in pH 1.5–12.0, respectively. 5 mL solution was taken out for test and 5 mL of fresh solution was replenished to supplement the release solution. The concentrations of released drugs were quantified every 2 h using a UV spectrophotometer at 266 nm to measure the drug release profile and their established standard curves obtained in various pH values solutions. The amount of released drug was determined by a cumulative curve. The drug release experiments were performed three times. The results were presented in terms of cumulative percentage release as a function of time using the following formula: |
 | (2) |
where Wi is the amount of Ibuprofen released from the conjugates at time t and Wd is the total amount of Ibuprofen loaded onto the hydrogels.
2.5.2 Drug release under applied voltage. Two circular platinum electrodes were kept in contact with the opposite surface of the swollen drug-loaded hydrogel in the 100 mL PBS (pH = 7.4), and different electric voltage of 0–0.5 V was applied. At different time intervals, 5 ml of solution was extracted from the solution and analyzed using a UV spectrophotometer at 266 nm, according to the standard calibration curve of Ibuprofen. The results were presented in terms of cumulative percentage release as a function of time using the following formula as above.To study the effect of electrode polarity on the release of the drug, the hydrogels were placed under the negatively charged electrode (anode in donor), and the positively charged electrode (cathode in donor), and under no current system delivery over a period of 48 h.
3. Results and discussion
3.1 Preparation of the drug loaded nf-BC/SA hybrid hydrogels
Ibuprofen was chosen as a model drug for exploring the drug release characteristics. The BC slurry was mixed with the sol of alginate by using strong stirring, then ethanol solution of Ibuprofen was incorporated. The aqueous solution of CaCl2 fog drops rained on the surface of BC caused inverse-phrase crosslinked though Ca2+ by the alginate adhered to the BC and formed a corrugated egg-box-like structure of calcium alginate dispersed in the BC network. In this way, the drug was encapsulated into the nf-BC/SA hybrid hydrogels with this special semi-IPN structure prepared as shown in Fig. 1.
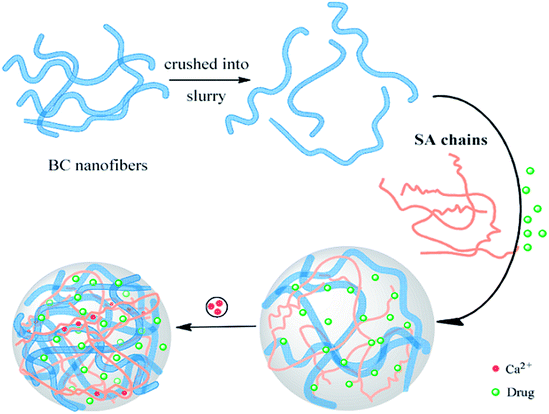 |
| Fig. 1 Formation process for the preparation of the drug loaded nf-BC/SA hybrid hydrogels with a semi-IPN structure. | |
3.2 Characterization of nf-BC/SA hybrid hydrogels
Thermogravimetric analysis (TGA) was conducted to examine the effect of composition and on the thermal decomposition of the nf-BC/SA hydrogels. The TG curves of BC, SA, and nf-BC/SA hydrogels were shown in Fig. 2a and b. BC underwent a single-step decomposition which was good corresponded to the previous report.29,31,43 The TG curves of nf-BC/SA hybrid hydrogels showed that underwent three weight loss steps. During the initial stage from room temperature to 100 °C, the moisture presented in the hybrid hydrogels vaporized. From 189 °C to 250 °C, the samples experienced a sharp weight loss, which might be ascribed to the destruction of SA backbone. When the temperature raised to 300 °C, the TG curves of nf-BC/SA showed a weight loss steps that were related to the thermal degradation of BC. As shown in Fig. 2b (the differential thermal gravity curves), the onset degradation temperatures of nf-BC/SA25, nf-BC/SA33, and nf-BC/SA50 hybrid hydrogels were 189 °C, 200 °C, and 212 °C, respectively. The decomposition temperatures of hybrid hydrogels were in the middle because of the molecular interactions enhanced on calcium alginate molecular chain by the presence of BC. Meanwhile, the decomposition temperatures were increased with the increasing content of SA. The phenomenon could be explained that the molecular interactions between BC and SA was enhanced by the increasing content of SA. All these above could be explained by a simple reason that the addition of BC afforded the hybrid hydrogels matrix a higher stability, particularly, by the excellent compatibility between the two carbohydrate components of the hybrid hydrogels.
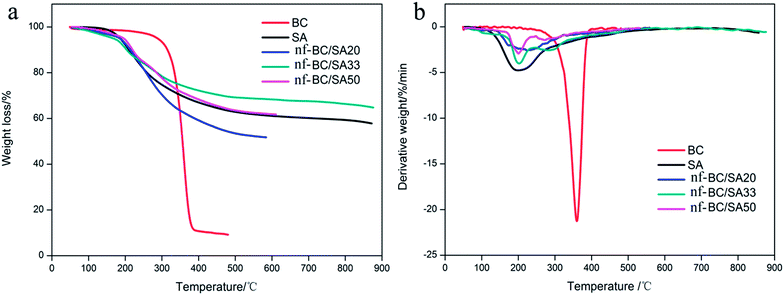 |
| Fig. 2 TG (a) and DTG (b) curves for the SA, BC, nf-BC/SA hybrid hydrogels. | |
Representative SEM micrographs of pure SA and nf-BC/SA hybrid hydrogels were presented in Fig. 3a–h, illustrating interconnected, asymmetric and highly porous structures with the three-dimensional interconnection throughout the hybrid hydrogels. Fig. 3a–d showed the surface of the samples presented a cellular-like structure. From the quantitative analysis of the micrographs (Fig. 3b–d), the pore size of the hybrid hydrogels was in the range of 100–328 μm. The surface microstructure of the nf-BC/SA was similar with that of bacterial cellulose/alginate as reported by Chiaoprakobkij.39 As shown in Fig. 3a–d, with increasing content of BC, a more favourable cellular semi-IPN structure in the hybrid hydrogels was formed, pore size became more homogeneous It was obvious that the extent of three-dimensional networks porous structure of hydrogel was significantly affected by the amount of BC. The nf-BC/SA33 had the most ideal structure with uniform pore diameters ranging from 100–200 μm. Fig. 3e–h showed the cross-sectional SEM images of the SA, nf-BC/SA50, nf-BC/SA33, and nf-BC/SA20 hybrid hydrogels. The calcium alginate microspheres were found interpenetrate on the BC network and formed semi-interpenetrating matrix. Compared with pure SA hydrogels, nf-BC/SA hybrid hydrogels presented a more stable and precise the microstructure. The porous structural properties were inclined to offer a larger specific surface area, which could provide a better interaction between solution and hydrogels, allowing easier solution uptake.44 The porous structural hydrogels might have larger bulk for drugs loading and release. Therefore, it could be important for controlling microstructure with the purpose of affecting the drug loading and releasing processes.
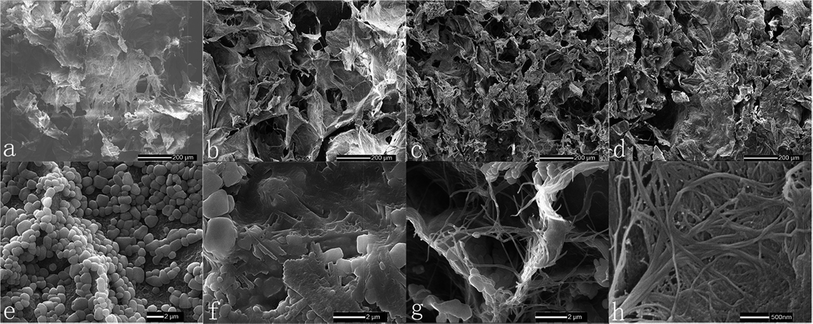 |
| Fig. 3 SEM micrographs of surface (top) and cross-section (bottom) of (a and e) SA, (b and f) nf-BC/SA50, (c and g) nf-BC/SA33, (d and h) nf-BC/SA20 hybrid hydrogels. | |
3.3 pH-responsive properties in swelling behaviours
We investigated the swelling behaviours of nf-BC/SA hybrid hydrogels in the conditions of different pH value. Fig. 4a showed the dynamic swelling profiles of nf-BC/SA with different SA weight fractions in PBS solutions (pH 7.4). Pure SA swelled rapidly and achieved a quick equilibrium within 20 min. Compared with about 7.8 times of the finally weight of pure SA, the supplement of BC which had an excellent water absorption capacity (14 times its dry weight) resulted in an increase in swelling behaviors but a delay in the process of swelling. As shown in Fig. 4b, the maximum weights of nf-BC/SA20, nf-BC/SA33, and nf-BC/SA50 hybrid hydrogels were 12.0, 11.6, and 10.5 times of their dry weight, respectively.
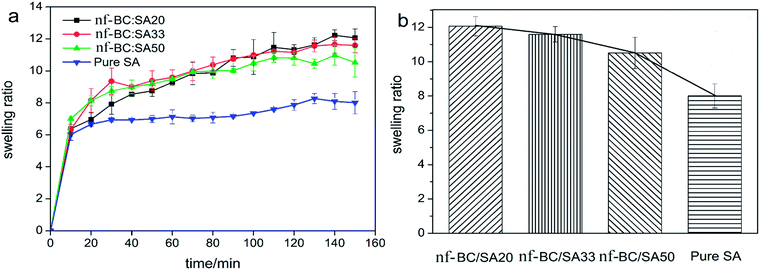 |
| Fig. 4 Swelling behaviors of the SA and nf-BC/SA hybrid hydrogels in neutral solution: (a) equilibrium swelling behaviors, (b) maximum swelling ratios in 150 min. | |
To further study, the nf-BC/SA33 was chosen to study the swelling behaviors in PBS with pH ranging from 1.5 to 12.0 shown in Fig. 5a and b. The swelling ratio was lowest in pH 1.5 which never surpassed the 8 times its dry weight. In neutral and alkaline environment the swelling ratio was considerably highly. As evidenced from the swelling data, the swelling ratio reached to about 11 times as the pH value increased to 6.5, which was in consistency with the data at pH 8.2. At pH 11.8 the sample constantly reached to more than 13 times when the process achieved equilibrium. From the swelling ratio it is obviously that the alkaline condition led to swelling more, while the acidic condition lowered the swelling degree.
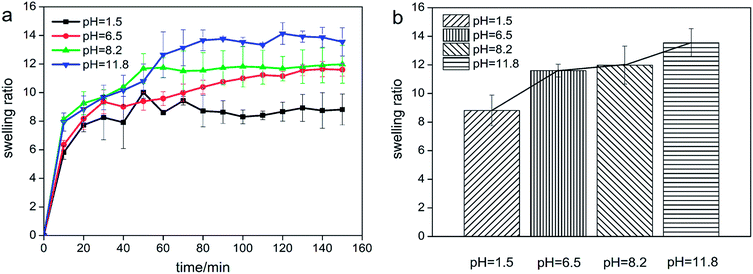 |
| Fig. 5 Swelling behaviors of the nf-BC/SA33 hybrid hydrogels in different pHs: (a) equilibrium swelling behaviors, (b) maximum swelling ratios in 150 min. | |
3.4 Electro-responsive properties in swelling behaviours
The investigation of the nf-BC/SA33 hybrid hydrogels swelling behavior under voltage applied was also performed in phosphate buffer at pH 7.4 shown in Fig. 6a and b. According to the previous report, pure SA hydrogels was nearly absent in electrical responsiveness under electric field.25 The enhanced swelling behaviours of nf-BC/SA33 hybrid hydrogels was observed when an electric stimulus was applied shown in Fig. 6. The swelling ratio was increased, which might be due to the presence of more number of –COO− groups in nf-BC/SA33 matrix. As mentioned in 3.2, the semi-IPN structure of nf-BC/SA33 offered a larger specific surface area which resulted in more number of active COO– groups, thus improving the electric response sensitivity. The swelling ratio under 0.15 V voltage applied was never surpassed the 11.6 times its dry weight, which was in consistency with the data at 0 V voltage applied. As evidenced from Fig. 6b, the swelling ratio reached about 11 times when the voltage increased to 0.3 V. The sample reached to 14 times under 0.5 V when the process achieved equilibrium. As can be observed from swelling data, swelling ratio of hybrid hydrogels increased when electric field was supplied. This could be contributed to electric field which accelerated the ionization of functional groups in the hydrophilic polymers. The ionization of functional groups caused the electrostatic repulsion and hydrophilicity of the polymer chains to increase significantly. These demonstrated characteristics of pH- and electro-responsive swelling behaviors suggested that the nf-BC/SA hybrid hydrogels was a kind of candidates for controlled release systems, as it could perceive the changes in environment.
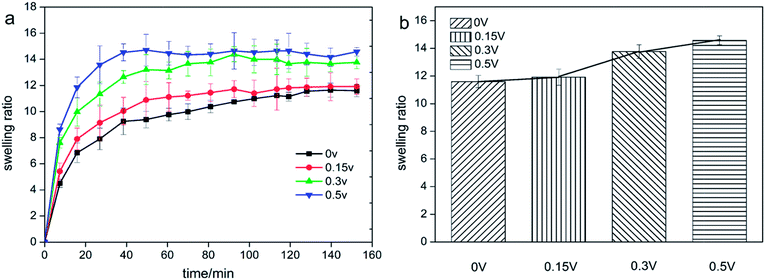 |
| Fig. 6 Swelling behaviors of the nf-BC/SA33 hybrid hydrogels in different voltages: (a) equilibrium swelling behaviors, (b) maximum swelling ratios in 150 min. | |
3.5 pH-controlled release behaviours of drug-loaded nf-BC/SA hybrid hydrogels
As previous reported about pH-sensitive hydrogels drug delivery systems, the swelling of hydrogel matrices will increase the drug release due to the enlarged mesh and the looser network.12,23,45 Taking the result of nf-BC/SA hybrid hydrogels had pH- and electro-responsive swelling behavior into consideration, we investigated whether they could achieve pH- and electro-responsive in drug release. Firstly we studied the drug release behaviors under several given pH conditions.
The schematic of drug release behaviors of Ibuprofen loaded nf-BC/SA hydrogels at pH 1.5–12.0 was shown in Fig. 7. It was noticed that Ibuprofen was released fast at alkaline conditions but slowly at acidic, which showed a similar trend to swelling behaviors of hydrogels. In low pH values, alginate was protonated into insoluble form of alginic acid which resulted in low swelling ratio. In higher pH conditions, an increase of the swelling behaviors occurred when the alginate converted to sodium salt. The deprotonation of alginic acid caused a break of hydrogen bands and the generation of the electrostatic repulsion among polymer chains, which led to the increase of swelling ratio.
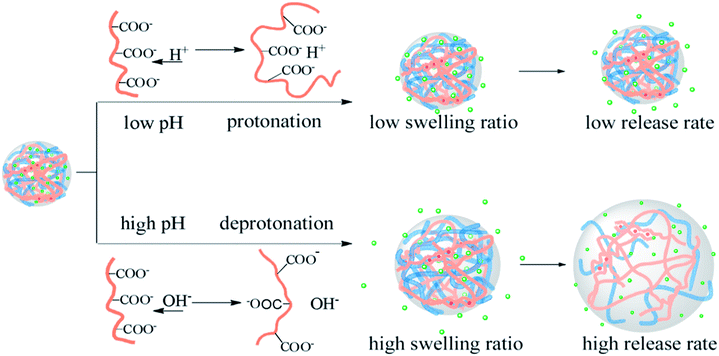 |
| Fig. 7 Releasing process for the drug loaded nf-BC/SA hybrid hydrogels under different pH values. | |
As can be seen from Fig. 8, during the first 2 h, the amount of Ibuprofen released was less than 10% in acidic conditions (pH = 1.5) while it reached 15% and 31% in pH = 7.0 and 11.8, respectively. After 6 h the amount of drug released in pH = 11.8 was twice as much as that in pH = 7.0. At 16 h, in pH 11.8, more than 90% of the drug was released from the hydrogels, while it took about 30 h to reach in pH 7.0. At the end of the release processes, the drug released amount reached more than 90% in pH = 11.8 and 7.0, but the amount was only about 60% in pH = 1.5. The drug release system showed a typical pH-sensitive release behavior, faster in neutral and alkaline medium but slower in acid medium. The hydrogels could protect the drug in the acidic stomach environment (pH 1.5) before releasing it in the small intestine (pH 7.0) for absorption into the systemic circulation. According to Fig. 8, the nf-BC/SA hybrid hydrogels could provide a sustainable and stable releasing for the model drug more than 20 h. Compared with pure SA hydrogels, the nf-BC/SA hydrogels effectively prolonged the drug release time.27 The drug release tendency observed here for the nf-BC/SA hybrid hydrogels, was in line with previous report described by Işıklan et al.27 The release behaviors had a pH-responsive characters which suggested that the nf-BC/SA hybrid hydrogels was a good candidate for colonic targeting delivery.
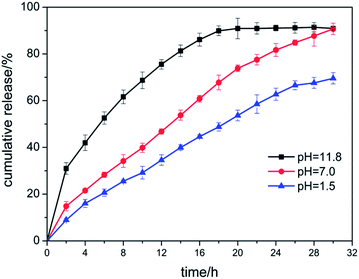 |
| Fig. 8 Amounts of Ibuprofen released from nf-BC/SA33 hydrogels at time t (h) under different pH values at 37 °C. | |
The Korsmeyer–Peppas mechanism is a semi-empiric model, in order to understand the release kinetics of the drug from the hydrogels to the elapsed time t:
|
 | (3) |
Mt/
Mn is the fractional release of drug in time
t,
k is a kinetic constant of the drug delivery system and
n is the diffusion exponent that characterizes the drug release mechanism.
46,47 This equation was used to the linearization of release data from several formulations. The
n and
k values were calculated from the slope and intercept of the plot of ln(
Mt/
Mn) against ln
t and represented in
Table 1 along with the regression coefficients. For cylindrical hydrogels, if the exponent
n < 0.45, then the drug release mechanism is a Fickian diffusion and if 0.45 <
n < 0.89, then it is a non-Fickian or anomalous diffusion. When
n > 0.89, the release mechanism is diffusion and zero-order (case II) transport.
Table 1 Release parameters of Ibuprofen sustained release from nf-BC/SA hybrid hydrogels in vary pH conditions
|
n |
k |
R2 |
pH = 11.8 |
0.498 |
0.2163 |
0.999 |
pH = 7.0 |
0.702 |
0.0825 |
0.996 |
pH = 1.5 |
0.772 |
0.0522 |
0.988 |
The release of drugs in pH 11.8–1.5 was fitted with the Korsmeyer–Peppas model. The exponent “n” revealed that the drug release mechanism of drug in pH = 11.8 was more diffusion-based (n = 0.498) than the one in pH = 7.0 (n = 0.702) or the one in pH = 1.5 (n = 0.77) as shown in Fig. 9. As the value of pH increased, the “n” exponent decreased. Therefore, the results showed that the samples obtained by loading the drug in porous matrices were controlled by a non-Fickian diffusion mechanism. Similar to this phenomenon, previous works reported that some pH-responsive hydrogels demonstrated a same releasing mechanism.10,38
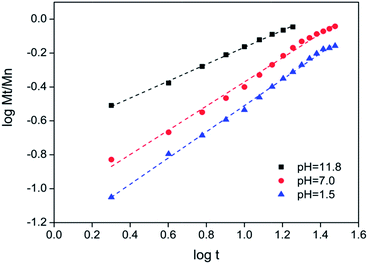 |
| Fig. 9 Korsmeyer–Peppas model for mechanism of drug release under different pH values at 37 °C. | |
3.6 Electro-responsive release behaviours of drug-loaded nf-BC/SA hybrid hydrogels
The release behaviors of Ibuprofen from nf-BC/SA hydrogels under various voltage applied in PBS solution (pH = 7.4) were shown in Fig. 10. Upon application of an electrical stimulus, the H+ ion, located in the nf-BC/SA33 matrix, preferred to escape and move to the cathode surface. The absence of H+ ion resulted in the presence of more number of ionizable –COO groups, thus enhancing swelling behaviours of nf-BC/SA33 hydrogels under electric stimulus and improving the electric response sensitivity.48 The drug could release into solution more rapidly with the increasing of electric voltage strength due to the higher swelling ratio of hydrogels. As shown in Fig. 11, during the first 2 h, the drug released about 10% at 0 V due to the lower swelling of hydrogels, as it reached 23% and 38% at 0.3 V and 0.5 V, respectively. After 8 h, the amount of drug released at 0.5 V was twice higher than that of 0 V. It took about 12 h and released more than 90% of the drug from the hydrogels at 0.5 V. A higher electric field strength induced a higher electrostatic force which drove the drug through the polymer matrix. Hence, the electro-responsive swelling behavior of hydrogels, was the main factor to effect the electro-sensitive control release of drug.13,14,49,50
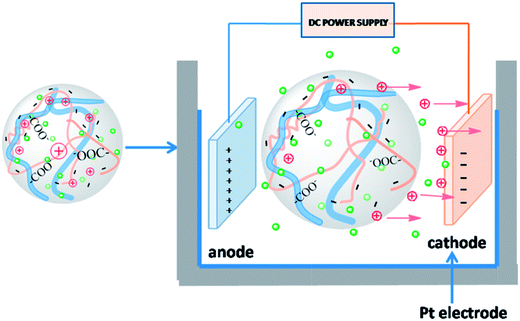 |
| Fig. 10 Releasing process for the drug loaded nf-BC/SA33 hybrid hydrogels under electric field. | |
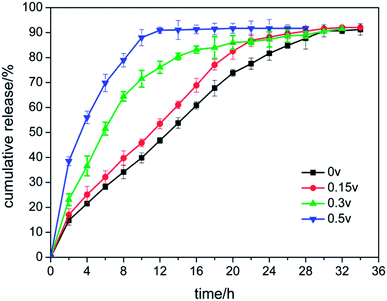 |
| Fig. 11 Amounts of Ibuprofen released from nf-BC/SA33 hydrogels at time t (h) under different electric field at 37 °C. | |
As the drug release from the swelling matrices under applied electric voltages was primarily controlled by diffusion process, hence, Peppas' semi-empirical model as shown in eqn (3) was applied in order to understand the release kinetics of the releasing process. The exponent “n” revealed that the drug release mechanism of drug under 0.5 V was more diffusion-based (n = 0.4919) than the others as shown in Fig. 11.
As shown in Fig. 12, the values of applied electric voltage were increased, the “n” exponent decreased. As shown in Tables 1 and 2 where the plots showed a high linearity. With high correlation coefficient (R2) values, the release profiles nf-BC/SA hybrid hydrogels could be explained by Korsmeyer–Peppas model.
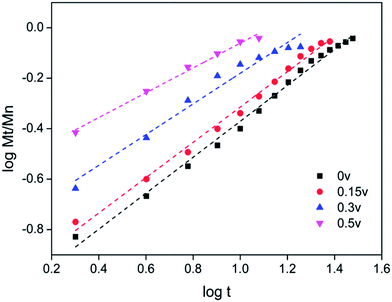 |
| Fig. 12 Korsmeyer–Peppas model for mechanism of drug release under different electric field at 37 °C. | |
Table 2 Release parameters of Ibuprofen sustained release from nf-BC/SA hydrogels in various electric field strengths
|
n |
k |
R2 |
0 V |
0.712 |
0.0825 |
0.996 |
0.15 V |
0.7004 |
0.0967 |
0.996 |
0.3 V |
0.60798 |
0.1628 |
0.985 |
0.5 V |
0.4919 |
0.2801 |
0.996 |
4. Conclusions
Hybrid hydrogels of bacterial cellulose nanofiber and sodium alginate (nf-BC/SA) was synthesized for application in drug delivery systems. The stimulus-responsive swelling properties and its stimulus-responsive drug release behaviors of the nf-BC/SA hydrogels, using Ibuprofen as a model drug, were investigated in vitro. With addition of BC nanofiber, nf-BC/SA hybrid hydrogels presented a more stable and precise the microstructure. Compared with pure SA hydrogels, the swelling properties of nf-BC/SA hybrid hydrogels not only maintained pH-responsive property but also effectively enhanced electro-responsive property. The swelling ratio was less than 8 times compared with its dry weight at acidic conditions (pH = 1.5) and it increased to more than 13 times when the pH value increased to 11.8, which might be related to the ionization of SA in higher pH conditions. The hybrid hydrogels also showed an increasing swelling ratio from 8 times to 14 times its dry weight under an increasing applied voltage (0–0.5 V). Effected by pH-responsive swelling behaviours, the drug release rate of nf-BC/SA hybrid hydrogels in vitro was quite depended on pH value, i.e. fast in neutral or alkaline media but slow in acidic media. The drug release could also be enhanced in the presence of electric stimulus when compared with passive release. The releasing rate was dependent on the applied electric strength. Furthermore, the drug release profiles was interpreted based on Peppas' semi-empirical equation that showed under pH and applied electric field the mechanism of drug release was characteristic for an anomalous transport, which can be regarded as a superposition of both Fickian diffusion and case-II transport (swelling and erosion controlled drug release).
Acknowledgements
This study was financially supported by the National Natural Science Foundation of China Project (Grant no. 51273021 and 51473019) and the National Science and Technology Support Project of China (Grant no. 2011BAK15B04).
References
- K. E. Uhrich, S. M. Cannizzaro, R. S. Langer and K. M. Shakesheff, Chem. Rev., 1999, 99, 3181–3198 CrossRef CAS PubMed.
- J. M. Dang and K. W. Leong, Adv. Drug Delivery Rev., 2006, 58, 487–499 CrossRef CAS PubMed.
- P. Gupta, K. Vermani and S. Garg, Drug Discovery Today, 2002, 7, 569–579 CrossRef CAS.
- S. Maiti, S. Ranjit and B. Sa, Int. J. PharmTech Res., 2010, 2, 1350–1358 CAS.
- L. Klouda and A. G. Mikos, Eur. J. Pharm. Biopharm., 2008, 68, 34–45 CrossRef CAS PubMed.
- W. C. Huang, T. J. Lee, C. S. Hsiao, S. Y. Chen and D. M. Liu, J. Mater. Chem., 2011, 21, 16077 RSC.
- A. V. Kabanov and S. V. Vinogradov, Angew. Chem., 2009, 48, 5418–5429 CrossRef CAS PubMed.
- J. Y. Sun, X. Zhao, W. R. Illeperuma, O. Chaudhuri, K. H. Oh, D. J. Mooney, J. J. Vlassak and Z. Suo, Nature, 2012, 489, 133–136 CrossRef CAS PubMed.
- H. Zhang, Y. Dong, L. Wang, G. Wang, J. Wu, Y. Zheng, H. Yang and S. Zhu, J. Mater. Chem., 2011, 21, 13530 RSC.
- C. Mura, A. Nacher, V. Merino, M. Merino-Sanjuan, M. Manconi, G. Loy, A. M. Fadda and O. Diez-Sales, Colloids Surf., B, 2012, 94, 199–205 CrossRef CAS PubMed.
- W. Wu, J. Liu, S. Cao, H. Tan, J. Li, F. Xu and X. Zhang, Int. J. Pharm., 2011, 416, 104–109 CrossRef CAS PubMed.
- O. Malay, A. Batigun and O. Bayraktar, Int. J. Pharm., 2009, 380, 120–126 CrossRef CAS PubMed.
- K. Juntanon, S. Niamlang, R. Rujiravanit and A. Sirivat, Int. J. Pharm., 2008, 356, 1–11 CrossRef CAS PubMed.
- Y. Y. Kim, J. Yun, Y. S. Lee and H. I. Kim, Carbon Lett., 2010, 11, 211–215 CrossRef.
- S. J. Kim, S. G. Yoon, S. M. Lee, J. H. Lee and S. I. Kim, Sens. Actuators, B, 2003, 96, 1–5 CrossRef CAS.
- Z. Jin, G. Guven, V. Bocharova, J. Halamek, I. Tokarev, S. Minko, A. Melman, D. Mandler and E. Katz, ACS Appl. Mater. Interfaces, 2012, 4, 466–475 CAS.
- K. H. Liu, T. Y. Liu, S. Y. Chen and D. M. Liu, Acta Biomater., 2008, 4, 1038–1045 CrossRef CAS PubMed.
- S. Niamlang and A. Sirivat, Int. J. Pharm., 2009, 371, 126–133 CrossRef CAS PubMed.
- T. S. Tsai, V. Pillay, Y. E. Choonara, L. C. Du Toit, G. Modi, D. Naidoo and P. Kumar, Polymers, 2011, 3, 150–172 CrossRef CAS PubMed.
- C. H. Goh, P. W. S. Heng and L. W. Chan, Carbohydr. Polym., 2012, 88, 1–12 CrossRef CAS PubMed.
- C. J. Knill, J. F. Kennedy, J. Mistry, M. Miraftab, G. Smart, M. R. Groocock and H. J. Williams, Carbohydr. Polym., 2004, 55, 65–76 CrossRef CAS PubMed.
- K. Y. Lee and D. J. Mooney, Prog. Polym. Sci., 2012, 37, 106–126 CrossRef CAS PubMed.
- C. Alvarez-Lorenzo, B. Blanco-Fernandez, A. M. Puga and A. Concheiro, Adv. Drug Delivery Rev., 2013, 65, 1148–1171 CrossRef CAS PubMed.
- N. Paradee, A. Sirivat, S. Niamlang and W. Prissanaroon-Ouajai, J. Mater. Sci.: Mater. Med., 2012, 23, 999–1010 CrossRef CAS PubMed.
- R. V. Kulkarni, C. M. Setty and B. Sa, J. Appl. Polym. Sci., 2010, 115, 1180–1188 CrossRef CAS.
- N. Işiklan, J. Appl. Polym. Sci., 2006, 99, 1310–1319 CrossRef.
- N. Işıklan, M. İnal, F. Kurşun and G. Ercan, Carbohydr. Polym., 2011, 84, 933–943 CrossRef PubMed.
- X. Y. Wu, S. W. Huang, J. T. Zhang and R. X. Zhuo, Macromol. Biosci., 2004, 4, 71–75 CrossRef CAS PubMed.
- J. George, K. V. Ramana, A. S. Bawa and Siddaramaiah, Int. J. Biol. Macromol., 2011, 48, 50–57 CrossRef CAS PubMed.
- P. Krishnamachari, R. Hashaikeh and M. Tiner, Micron, 2011, 42, 751–761 CrossRef CAS PubMed.
- C. J. Grande, F. G. Torres, C. M. Gomez and M. C. Bano, Acta Biomater., 2009, 5, 1605–1615 CrossRef CAS PubMed.
- J. Wu, Y. Zheng, X. Wen, Q. Lin, X. Chen and Z. Wu, Biomed. Mater., 2014, 9, 35005–35014 CrossRef PubMed.
- S. Liu, S. Jeannes and B. Chen, J. Biomater. Tissue Eng., 2011, 1, 60–67 CrossRef PubMed.
- Q. Cui, Y. Zheng, Q. Lin, W. Song, K. Qiao and S. Liu, RSC Adv., 2014, 4, 1630–1639 RSC.
- J. Wu, Y. Zheng, Z. Yang, Q. Lin, K. Qiao, X. Chen and Y. Peng, RSC Adv., 2014, 4, 3998–4009 RSC.
- J. Wu, Y. Zheng, W. Song, J. Luan, X. Wen, Z. Wu, X. Chen, Q. Wang and S. Guo, Carbohydr. Polym., 2014, 102, 762–771 CrossRef CAS PubMed.
- A. Stoica-Guzun, M. Stroescu, F. Tache, T. Zaharescu and E. Grosu, Nucl. Instrum. Methods Phys. Res., Sect. B, 2007, 265, 434–438 CrossRef CAS PubMed.
- M. C. I. Mohd Amin, N. Ahmad, N. Halib and I. Ahmad, Carbohydr. Polym., 2012, 88, 465–473 CrossRef CAS PubMed.
- N. Chiaoprakobkij, N. Sanchavanakit, K. Subbalekha, P. Pavasant and M. Phisalaphong, Carbohydr. Polym., 2011, 85, 548–553 CrossRef CAS PubMed.
- Q. Lin, Y. Zheng, L. Ren, J. Wu, H. Wang, J. An and W. Fan, J. Appl. Polym. Sci., 2014, 131, 39848–39856 Search PubMed.
- R. Xing, H. Lin, P. Jiang and F. Qu, Colloids Surf., A, 2012, 403, 7–14 CrossRef CAS PubMed.
- Ö. Malay, A. Batıgün and O. Bayraktar, Int. J. Pharm., 2009, 380, 120–126 CrossRef PubMed.
- L. C. Tomé, L. Brandão, A. M. Mendes, A. J. D. Silvestre, C. P. Neto, A. Gandini, C. S. R. Freire and I. M. Marrucho, Cellulose, 2010, 17, 1203–1211 CrossRef.
- W. Wu, J. Liu, S. Cao, H. Tan, J. Li, F. Xu and X. Zhang, Int. J. Pharm., 2011, 416, 104–109 CrossRef CAS PubMed.
- P. Gupta, K. Vermani and S. Garg, Drug Discovery Today, 2002, 7, 569–579 CrossRef CAS.
- S. Dash, P. N. Murthy, L. Nath and P. Chowdhury, Acta Pol. Pharm., 2010, 67, 217–223 CAS.
- I. F. Alexa, M. Ignat, R. F. Popovici, D. Timpu and E. Popovici, Int. J. Pharm., 2012, 436, 111–119 CrossRef CAS PubMed.
- W. C. Huang, T. J. Lee, C. S. Hsiao, S. Y. Chen and D. M. Liu, J. Mater. Chem., 2011, 21, 16077–16086 RSC.
- J. Yun, J. S. Im, Y.-S. Lee, T.-S. Bae, Y.-M. Lim and H.-I. Kim, Colloids Surf., A, 2010, 368, 23–30 CrossRef CAS PubMed.
- J. Yun, J. S. Im, Y.-S. Lee and H.-I. Kim, Eur. Polym. J., 2011, 47, 1893–1902 CrossRef CAS PubMed.
|
This journal is © The Royal Society of Chemistry 2014 |