DOI:
10.1039/C4RA09470K
(Paper)
RSC Adv., 2014,
4, 56992-56997
A comparative study of antioxidant-loaded carbon nanoparticles as drug delivery vehicles
Received
29th August 2014
, Accepted 28th October 2014
First published on 28th October 2014
Abstract
Chronic obstructive pulmonary disease (COPD) describes chronic lung diseases that cause limitations in lung airflow. Reactive oxygen species (ROS) play a central role in causing COPD and scavenging of ROS holds great promise to alleviate the symptoms of COPD. Antioxidant proteins such as catalase are good therapeutic drug candidates; however, they are easily degraded by proteases in vivo. Here, we investigated the characteristics of carbon nanoparticles as vehicles for delivering antioxidant proteins to macrophages. A direct comparison using catalase was conducted from zero to three dimensional carbon nanoparticles, namely: fullerene (zero dimensional (0D)), carbon nanotubes (CNTs, one dimensional (1D)), graphene oxide (GO, two dimensional (2D)), and graphene aerogel (GA, three dimensional (3D)). Over 90% of catalase was encapsulated in CNTs, GO, and GA. GA released less than 3% catalase in 24 hours, which is useful for long-term release. Fullerene delivered catalase inside macrophages with high efficiency although the encapsulation of catalase in the fullerene was 39.7%. Functionalization of carbon nanoparticles can modify the chemical properties that affect the characteristics of drug delivery vehicles. The release rate of catalase from functionalized carbon nanoparticles, CNTs with 2.0 wt% (percent by weight) of –COOH and GO, is 15–20% in 4 hours, which is much higher than GA. As drug delivery vehicles, carbon nanoparticles are very promising due to their high loading efficiency of therapeutic drugs and tunable surface properties. We also anticipate numerous applications of porous carbon nanoparticles for treating lung diseases, since low density nanoparticles can reach the deep lung efficiently.
Introduction
Chronic obstructive pulmonary disease (COPD), one of the many types of airway obstructions, is an irreversible chronic inflammatory condition in the lungs.1,2 COPD causes the respiratory passages to swell and become irritated which makes it hard to breathe.3,4 COPD is a major health problem worldwide and most COPD patients are smokers.1,2 Currently there is no cure for COPD; however, smoking cessation is beneficial for both development and prognosis of COPD.5,6 The major risk factor for COPD is the reactive oxygen species (ROS) from cigarette smoking and other lung irritants such as air pollution, chemical fumes, or dust.7,8
ROS are highly reactive chemical forms of oxygen such as superoxide (O2˙−), hydrogen peroxide (H2O2), singlet oxygen (1O2), and hydroxyl radical (HO˙).9 ROS are produced as by-products of normal cellular metabolism and they are very useful tools to destroy biological pathogens by damaging their proteins, lipids, and DNA.10 However, overproduction of ROS is toxic to even host cells.11 H2O2 is a relatively stable ROS and mild oxidant; however, H2O2 plays an important role in ROS-induced injury.12 H2O2 is uncharged so that it can freely diffuse through cell membranes and H2O2 acts as a signalling molecule or mediator of apoptotic cell death.11,13,14 H2O2 also stimulates the nicotinamide adenine dinucleotide phosphate (NADPH) oxidase and xanthine oxidase to convert oxygen into superoxide that enhances oxidative stress.15
Catalase (EC 1.11.1.6), one of antioxidant enzymes, can decompose H2O2 into water and oxygen, which are non-toxic compounds.16 Some antioxidants such as superoxide dismutase generate H2O2 as a by-product of enzymatic or non-enzymatic process and catalase can also scavenge H2O2 produced by other antioxidants.17 Catalase can protect cells from the apoptosis induced by H2O2 and catalase can be a beneficial therapeutic drug candidate for the treatment of COPD. However, the therapeutic proteins including catalase are not membrane-permeable and easily degraded in vivo; therefore, an effective drug delivery system for catalase is required.18–20 In the past decades, the rapid development of nanotechnology has brought many fascinating ideas and opportunities to disease diagnosis and treatment.21 In the realm of drug delivery, carbon nanomaterials, notably 0D fullerenes, 1D carbon nanotubes (CNTs), 2D graphene, have gained tremendous attention as promising nano-carriers, owing to their distinct characteristics, such as high surface area, enhanced cellular uptake and the possibility to be easily conjugated with many therapeutics, including both small molecules and biologics, displaying superior efficacy, enhanced specificity and diminished side effects.22–26 Fullerene is a nanoscaled carbonous material with unique photochemical, electrochemical, and physical properties. With its low systemic toxicity, fullerene shows tremendous promise for target-specific delivery of drugs in the body.22 CNTs are cylindrical tubes of sp2 carbon, which can be conjugated with drugs and biomolecules by covalent or noncovalent interaction methods and used as molecular carriers for in vitro and in vivo drug delivery.26 Recently, graphene, a 2D mono-layer of carbon atoms, has attracted much attention due to its fascinating properties such as high electrical conductivity, high thermal conductivity, extraordinary elasticity, large specific surface area, and biocompatibility, etc.27 In the area of nano-medicine, graphene and its composites have emerged as new biomaterials that provide exciting opportunities for the development of a broad range of applications, including a new generation of biosensors, nano-carriers for drug delivery and probes for cell and biological imaging. However, it is very difficult to compare and evaluate the drug delivery performance of the different carbon nanofillers based on the literature data because the drug delivery related data varies with many uncontrolled factors such as the drug type.
Due to the low density, inhalation of porous nanoparticles can deliver therapeutic drugs to the deep lung efficiently. Here, we propose nanoparticles based on carbon for treatment of inflammatory lung diseases including COPD. We encapsulated catalase, one of antioxidant proteins, in four carbon-based materials: 0D fullerene, 1D CNT, 2D graphene oxide (GO), and three-dimensional (3D) graphene aerogel (GA), and investigated the effects of these carbon nanoparticles on the loading efficiency, releasing rate of catalase, and the effectiveness of COPD treatment.
Materials and methods
Materials
Multi-walled CNTs of diameter 10–20 nm, length about 30 μm, 2.0 percent by weight (wt%) of –COOH, and purity > 95% were purchased from Chengdu Organic Chemicals Co. Ltd. Fullerene (C60), graphite powder with particle size <20 μm, hydrogen peroxide (H2O2, 30%), hydroiodic acid (HI, 30%), and catalase were obtained from Sigma-Aldrich Co. Ltd. All of the materials were directly used without further purification.
Preparation 2D GO and 3D GA
GO was synthesized from graphite powder with modified Hummer's method, a detailed description can be found in our previous paper.18 GA was subsequently fabricated by hydrothermal treatment of GO suspensions with the assistance of HI. First, 1 mL of HI (30%) was added into 60 mL homogeneous GO (2 mg mL−1) aqueous solution, and then the mixture was sealed in a 100 mL Teflon-lined stainless steel autoclave and maintained at 180 °C for 12 h. The as-prepared graphene hydrogels were taken out from reactor and dipped into distilled water for 24 h to remove the residual HI. Finally, the samples were freeze-dried to obtain GA.
Encapsulation of catalase in carbon nanoparticles
Each carbon nanoparticle (10 mg mL−1), fullerene, CNT, GO, and GA, was dispersed with a sonicator for 10 minutes to suspend the nanoparticles into short segments. Catalase (3.3 mg mL−1) was mixed with each carbon nanoparticle for 24 hours. In order to measure the loading efficiency of catalase in carbon nanoparticles, the catalase was labeled with fluorescein isothiocyanate (FITC). Briefly, catalase was dissolved in pH 9.0 buffer solution (100 mM, carbonate buffer) and 50 μL of 10 mg mL−1 FITC in DMSO was mixed with catalase solutions for 2 h at room temperature. The FITC labeled catalase were separated from unreacted FITC using a PD-10 desalting column equilibrated in PBS (pH 7.4). FITC-catalase in carbon nanoparticles were separated from the aqueous medium by ultracentrifugation at 8000 rpm for 5 min. The amount of catalase encapsulated in the nanoparticles was determined by the amount of catalase in supernatant, which is the difference between the total amount of drug used to prepare the nanoparticles and the amount of drug present in the aqueous medium.
Physical characterization using microscopy
The morphology of fullerene, CNT, and GA was examined by a JEOL JSM-7500 field-emission scanning electron microscopy (FESEM). The GO nano-sheets were characterized by a Veeco Nanoman atomic force microscopy (AFM) using tapping mode and operated in air.
Determination of catalase activity in vitro
Catalase activity was measured by the decomposition rate of H2O2. A solution containing either free catalase (0.1 mL of 16.6 μg mL−1) or equivalent amount of catalase in carbon-based nanoparticles was mixed with 2.9 mL of 10 μM H2O2 in 50 mM phosphate buffer (pH 7.4) and the absorbance of H2O2 was monitored at 240 nm.
The release of catalase from the carbon nanoparticles in vitro
The release of catalase from the carbon nanoparticles was evaluated using FITC-labeled catalase. FITC-catalase-loaded carbon nanoparticles (1.5 mg) were suspended in pH 7.4 buffer solutions (1.5 mL) and the suspensions were kept at 37 °C under gentle shaking. For statistical analysis, 3 independent samples per group were prepared. At specific time points, the suspensions were centrifuged at 8000 rpm for 2 min and the fluorescence of supernatant was then analyzed with a Cary Eclipse spectrofluorophotometer (Victoria, Australia) (λex/λem = 494/510 nm). The pellets were re-suspended with fresh buffer solutions (1.5 mL) and the procedure was repeated for each time point.
Cell culture
RAW264.7 macrophage (ATCC number: TIB-71) from the American Type Culture Collection (ATCC) (Manassas, VA) were maintained at 37 °C under a humidified atmosphere of 5% CO2 in Dulbecco's modified eagle's medium (DMEM) containing 10% (v/v) fetal bovine serum, supplemented with penicillin (100 units per mL) and streptomycin (100 μg mL−1).
Cellular uptake of catalase-loaded carbon nanoparticles
The cellular uptake of catalase-loaded carbon nanoparticles was detected by flow cytometry (BD Accuri™ C6, BD Biosciences) (San Jose, CA) using FITC-catalase. RAW264.7 macrophages were incubated with either 166.7 μg mL−1 FITC-catalase, or the equivalent amount of FITC-catalase-loaded carbon nanoparticles. At specific time points, the cells were washed 3 times and analyzed by flow cytometry.
Activity test of catalase in carbon-based nanoparticles – measurement of intracellular H2O2
RAW-264.7 macrophages were incubated with either 166.7 μg mL−1 catalase or an equivalent catalase concentration of catalase in carbon nanoparticles for 2 hours. Macrophages were washed with PBS and treated with 100 μM H2O2 for 4 hours. The cells were washed with PBS 3 times, trypsinized, and stained by 5 μM5-(and-6)-chloromethyl-2′,7'-dichlorodihydrofluorescein diacetate, acetyl ester (CM-H2DCFDA) (Invitrogen, Carlsbad, CA) for measuring H2O2. After 20 min, cells were washed 3 times with ice cold PBS. Fluorescence was measured by flow cytometry (BD Accuri™ C6, BD Biosciences) (San Jose, CA) using a laser for CM-H2DCFDA (λex/λem = 488/530 nm).
Cytotoxicity of the carbon nanoparticles (MTT reduction assay)
An MTT (3-(4,5-dimethylthiazol-2-yl)-2,5-diphenyl tetrazolium bromide) reduction assay was performed to measure the toxicity of the carbon nanoparticles. The macrophages (1 × 106 cells per well, 12 well plate) in DMEM with 10% FBS were incubated with the carbon nanoparticles for 0.5, 1, 2, and 4 h. Cells were treated with the carbon nanoparticles at various particle concentrations (0.01 mg mL−1–10 mg mL−1). Next, 20 μL of MTT solution (5 mg mL−1 in PBS) was added to each well, and the cells were incubated for 2 hours. Then, 200 μL of dimethyl sulfoxide (DMSO) was added to dissolve the resulting formazan crystals. After 10 min of incubation, the absorbance at 585 nm was measured using an Infinite M200 PROmicroplate reader (Tecan, Männedorf, Switzerland). Percentage cell viability was calculated by comparing the absorbance of the control cells to that of carbon nanoparticle-treated cells.
Results and discussion
As shown schematically in Fig. 1, we demonstrate the characteristics of carbon nanoparticles as vehicles for delivering a therapeutic protein, catalase, to macrophages. A direct comparison between carbon nanoparticles was conducted from zero to three dimension carbon nanoparticles, namely: fullerene, CNT, GO, and GA.
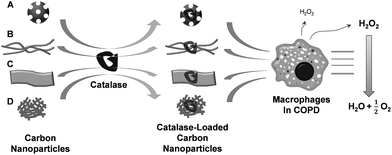 |
| Fig. 1 Schematic diagram of catalase-loaded carbon nanoparticles. Catalase can be loaded in carbon nanoparticles by non-specific adsorption. (Carbon nanoparticles – (A): zero-dimensional (0D) fullerene, (B): one-dimensional (1D) carbon nanotubes (CNTs), (C): two-dimensional (2D) graphene oxide, and (D): three-dimensional (3D) graphene aerogel) Carbon nanoparticles enhance the delivery of catalase to macrophages in the presence of 10% serum and the catalase-loaded carbon nanoparticles can scavenge hydrogen peroxide (H2O2)produced by macrophages. | |
Representative SEM images of fullerene, CNT, and GA, which were dispersed in aqueous solution with ultrasonication for 1 h, are shown in Fig. 2. As shown in Fig. 2a, the typical fullerene powers are irregular shape particles and rods with diameter from several hundred nanometer to several microns. As shown in Fig. 2b, it is clear that the CNTs are in the form of wires that are several tens of microns long. In addition, some agglomerated particles are also observed. The GA obtained is ultra-light with a density of 0.022 g cm−3. The typical morphology of GA is shown in Fig. 2c. It is shown from the SEM images that the GA has a 3D porous structure, with pore size in the range of a few to several microns.
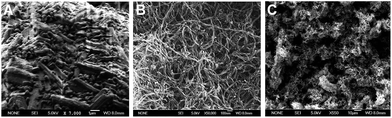 |
| Fig. 2 SEM images of carbon nanoparticles. A: fullerene with magnification ×7000, B: CNT with magnification ×50 000, C: graphene aerogel with magnification ×550. SEM images were taken on a JEOL JSM-7500 field-emission scanning electron microscopy (FESEM). | |
Interconnected porous with many wrinkles can be seen in the high-magnification SEM image, indicating the formation of 3D network structure via aggregation of reduced GO sheets.27 The morphology of GO nanosheets prepared has been characterized by AFM and shown in Fig. 3. The GO sheets show irregular shapes with uniform thickness, and their sizes are in the order of several hundred nanometers. As shown in Fig. 3b, the thickness of the GO sheets is typically around 1.0 nm, indicating that a single layer of GO is achieved.28
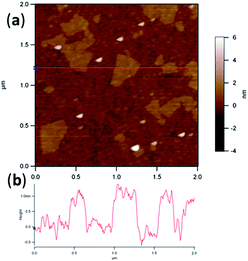 |
| Fig. 3 A. Typical tapping-mode AFM image of GO sheets; B. line profile for the sheet marked by the red line in image (A). | |
Therapeutic proteins such as catalase can be loaded in carbon nanoparticles by non-specific adsorption that depends on hydrophobic interaction. Fullerene, CNT, and graphene are considered to be hydrophobic materials that enhance the encapsulation of catalase in carbon nanoparticles. In Fig. 4A, it is seen that the loading efficiency of catalase into GA is 97.6 ± 0.1% (mean ± standard deviation) in 24 h of incubation, which is higher than the loading efficiency of catalase into fullerenes (39.7 ± 4.8%). Fullerenes are similar to GA in chemical structure; however, GA is a single layer of densely packed carbon atoms, which have higher surface area. A fullerene composed entirely of carbon is in the form of a sphere and the size of fullerenes is about 0.7–1.0 nm in diameter. Fullerenes particles mainly contained micropores (<2 nm diameter) and the pore size is smaller than the radius of gyration (RG) of catalase, 39.8 Å (3.98 nm), which reduces the accessibility of catalase inside fullerene. Hydrophobic interaction enhances the encapsulation of therapeutic drugs in carbon nanoparticles and therapeutic drugs are released from carbon nanoparticles very slowly. Only less than 3% of catalase is released from GA in 4 hours (Fig. 4B), which can produce long-term drug release profiles. However, native proteins could partially unfold when they are exposed to hydrophobic groups that reduce enzyme activities. Amphiphilic compounds such as sodium dodecyl sulfate (SDS), Triton X-100, Tween-20 and pluronics can be used to decrease the hydrophobicity of carbon nanotubes and they can suspend carbon nanotubes in water. However, surfactants might be irrelevant in biological applications since they may denature proteins and lyse cell membranes. Functionalization of carbon nanoparticles is one strategy to promote hydrophilic property to carbon nanoparticles and change the release profile. The loading efficiency of catalase in functionalized carbon nanoparticles is 93.2 ± 1.5% for GO and 96.6 ± 2.2% for CNT with 2.0 wt% of –COOH, which is almost same loading efficiency as GA. The release rate of catalase from GO or CNT with 2.0 wt% of –COOH is 10–20% in 4 hours, which is much higher than GA (Fig. 4B).
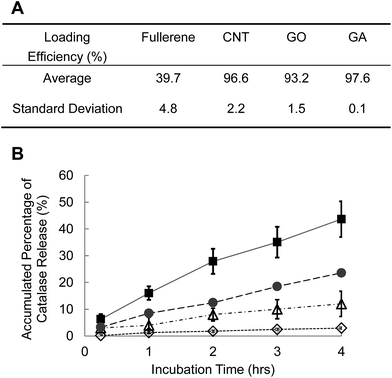 |
| Fig. 4 A. Loading efficiency of catalase in four carbon nanoparticles, zero-dimensional (0D) fullerenes, 1D carbon nanotubes (CNTs), 2D graphene oxide (GO), and 3D graphene aerogel (GA), B. accumulated release of FITC-labelled catalase from the carbon nanoparticles – fullerene (triangle), CNT (circle), GO (square), and GA (diamond). Error bar = mean ± standard deviation. | |
The activity of catalase encapsulated in carbon nanoparticles was also investigated. As shown in Fig. 5, catalase in fullerene, CNT and GO retained 40–60% activity of intact catalase and the decrease of catalase activity probably resulted from the hydrophobicity interaction between catalase and carbon nanoparticles during the encapsulation. Proteins are easily unfolded or denatured when they are exposed to hydrophobic environments. GA might provide more hydrophobic environment and catalase in GA lost its activity more than the activities in other delivery vehicles.
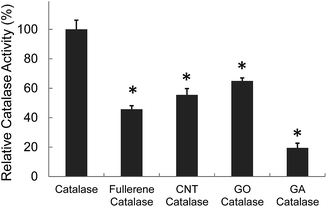 |
| Fig. 5 Enzyme activity of catalase in carbon nanoparticles (0D fullerenes, 1D CNTs, 2D GO, and 3D GA) in vitro catalase activity was determined by measuring the reduction rate of H2O2. Significance of results was determined via the t-test compared to catalase only with *p < 0.05. Error bar = mean ± standard deviation. | |
In COPD, alveolar macrophages are markedly increased and they stimulate the secretion of cytokines and ROS that cause all pathophysiological features of COPD.7 Alveolar macrophages are a major target for anti-inflammatory therapy. We performed experiments to determine if the carbon nanoparticles could enhance the delivery of catalase into macrophages.
For the uptake study, the carbon nanoparticles are loaded with FITC-labeled catalase and incubated with macrophages (RAW264.7) in 10% serum. The uptake of catalase by cells is then measured by flow cytometry. Fig. 6 demonstrates that the uptake of GA by macrophage is less than 20% for 3 hours. In contrast, more than 90% of fullerene is delivered inside the cells in 3 hours. The uptake of GO and CNT by cells are between 20% and 40% at the same time point. Cellular uptake of nanoparticles depends on several factors such as hydrophobicity, surface charge, shape, and particle size. Among the four carbon nanoparticles, fullerene, the smallest in size, has more cellular uptake than those of others.
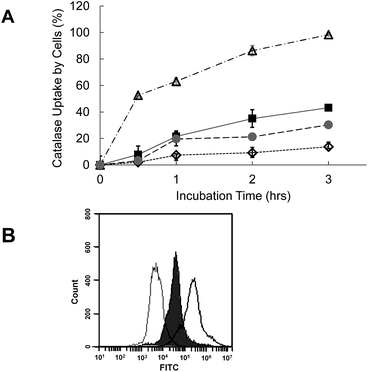 |
| Fig. 6 The carbon nanoparticles can enhance the uptake of catalase in vitro. A. Cellular uptake of the catalase by macrophages was measured in vitro (flow cytometry) – fullerene (triangle), CNTs (circle), GO (square), and GA (diamond), error bar = mean ± standard deviation, catalase uptake by cells (%) = (Fcnp − Ffc)/(Fcnp0 − Ffc) × 100 where Fcnp0: total fluorescence of carbon nanoparticles before the cellular uptake, Fcnp: fluorescence of carbon nanoparticles after the cellular uptake, and Ffc: fluorescence of FITC-catalase (free catalase with no carbon nanoparticles) after the cellular uptake. B. A flow cytometer detected macrophages without any carbon nanoparticles (gray solid line) or with GO (filled line) or fullerene (black solid line) after 3 hour incubation. All carbon nanoparticles encapsulated FITC-labeled catalase. | |
We have looked into the question of whether the carbon nanoparticles could deliver catalase into macrophages and scavenge H2O2 in vitro. Fig. 7 demonstrates that the carbon nanoparticles can enhance the delivery of catalase to macrophages in the presence of 10% serum. Free catalase by itself scavenged H2O2 by 13% compared to the H2O2 level without catalase, whereas catalase in GO or GA removed H2O2 by 20–24% (Fig. 7A). Catalase in GO or GA protected macrophages from the toxicity caused by H2O2 more efficiently (Fig. 7B). Especially the catalase-loaded fullerene scavenged H2O2 by 60–80%. Presumably the activity of catalase in fullerene was enhanced by the increased uptake of fullerene by macrophages.
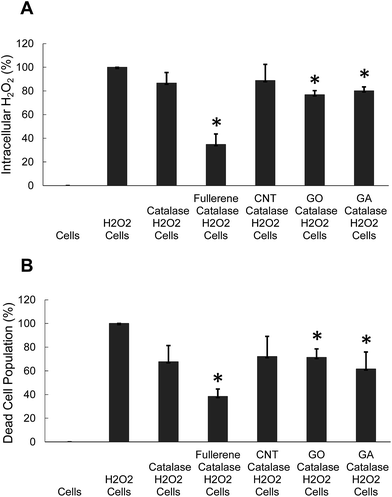 |
| Fig. 7 Catalase-loaded fullerene protects macrophages from the toxicity of H2O2. Macrophages were incubated with either catalase-loaded carbon nanoparticles or free catalase. The cells were washed 3 times before they were treated with H2O2. A. The intracellular level of H2O2 and B. dead cell population were measured. For statistical analysis, 3 independent wells were measured for each sample. Significance of results was determined via the t-test compared to cells only with *p < 0.05. Error bar = mean ± standard deviation. | |
Conclusion
In this work, we investigated the basic characteristics of four types of carbon nanoparticles, namely, 0D fullerenes, 1D CNT, 2D GO, and 3D GA, as drug delivery vehicles. Over 90% of catalase, a therapeutic protein, was encapsulated in CNT, GO, and GA while 39.7% of catalase was encapsulated in fullerene. Graphene aerogel can be used for a long-term release of catalase since less than 3% catalase was released in 24 hours. The release rate was modified by functionalization of carbon nanoparticles. The release rate of catalase from COOH with 2.0 wt% of –COOH and GO was 10–20% in 4 hours, which was much higher than GA. Based on the results, we anticipate a wide range of application of carbon nanoparticles in drug delivery, due to high loading efficiency of therapeutic drugs, tunable surface property, high surface area, and enhanced cellular uptake.
Notes and references
- P. G. Gibson and J. L. Simpson, Thorax, 2009, 64, 728–735 CrossRef CAS PubMed
. - H. Magnussen, K. Richter and C. Taube, Clin. Exp. Allergy, 1998, 28(suppl. 5), 187–194 CrossRef
; discussion 203–185. - R. A. Pauwels, A. S. Buist, P. M. Calverley, C. R. Jenkins, S. S. Hurd and G. S. Committee, Am. J. Respir. Crit. Care Med., 2001, 163, 1256–1276 CrossRef CAS PubMed
. - R. R. Wright, Am. J. Pathol., 1960, 37, 63–77 CAS
. - R. Guo, M. H. Pittler and E. Ernst, Eur. Respir. J., 2006, 28, 330–338 CrossRef CAS PubMed
. - R. Pires-Yfantouda, G. Absalom and F. Clemens, Respir. Care, 2013, 58, 1955–1962 CrossRef PubMed
. - P. J. Barnes, Pharmacol. Rev., 2004, 56, 515–548 CrossRef CAS PubMed
. - I. Rahman and W. MacNee, Curr. Opin. Pharmacol., 2012, 12, 256–265 CrossRef CAS PubMed
. - S. S. Gill and N. Tuteja, Plant Physiol. Biochem., 2010, 48, 909–930 CrossRef CAS PubMed
. - T. Finkel and N. J. Holbrook, Nature, 2000, 408, 239–247 CrossRef CAS PubMed
. - H. Sies and H. de Groot, Toxicol. Lett., 1992, 64–65 Search PubMed
spec no, 547–551. - J. Bai and A. I. Cederbaum, Biol. Signals Recept., 2001, 10, 189–199 CrossRef CAS PubMed
. - G. P. Bienert, J. K. Schjoerring and T. P. Jahn, Biochim. Biophys. Acta, 2006, 1758, 994–1003 CrossRef CAS PubMed
. - X. Luo, B. Chen, R. Zheng, P. Lin, J. Li and H. Chen, Neurosci. Lett., 2010, 485, 60–64 CrossRef CAS PubMed
. - E. W. Kellogg III and I. Fridovich, J. Biol. Chem., 1975, 250, 8812–8817 Search PubMed
. - H. N. Kirkman and G. F. Gaetani, Proc. Natl. Acad. Sci. U. S. A., 1984, 81, 4343–4347 CrossRef CAS
. - J. M. McCord, B. B. Keele Jr and I. Fridovich, Proc. Natl. Acad. Sci. U. S. A., 1971, 68, 1024–1027 CrossRef CAS
. - P. Ghosh, X. Yang, R. Arvizo, Z. J. Zhu, S. S. Agasti and Z. Mo, et al., J. Am. Chem. Soc., 2010, 132, 2642–2645 CrossRef CAS PubMed
. - S. Lee and N. Murthy, Biochem. Biophys. Res. Commun., 2007, 360, 275–279 CrossRef CAS PubMed
. - S. Lee, S. C. Yang, M. J. Heffernan, W. R. Taylor and N. Murthy, Bioconjugate Chem., 2007, 18, 4–7 CrossRef CAS PubMed
. - Z. Liu, J. T. Robinson, S. M. Tabakman, K. Yang and H. Dai, Mater. Today, 2011, 14, 316–323 CrossRef CAS
. - J. Shi, H. Zhang, L. Wang, L. Li, H. Wang and Z. Wang, et al., Biomaterials, 2013, 34, 251–261 CrossRef CAS PubMed
. - J. Shi, L. Wang, J. Gao, Y. Liu, J. Zhang and R. Ma, et al., Biomaterials, 2014, 35, 5771–5784 CrossRef CAS PubMed
. - B. S. Wong, S. L. Yoong, A. Jagusiak, T. Panczyk, H. K. Ho and W. H. Ang, et al., Adv. Drug Delivery Rev., 2013, 65, 1964–2015 CrossRef CAS PubMed
. - H. Dong, C. Dong, T. Ren, Y. Li and D. Shi, J. Biomed. Nanotechnol., 2014, 10, 2086–2106 CrossRef PubMed
. - F. Karchemski, D. Zucker, Y. Barenholz and O. Regev, J. Controlled Release, 2012, 160, 339–345 CrossRef CAS PubMed
. - Y. Li, Y. A. Samad, K. Polychronopoulou, S. M. Alhassan and K. Liao, Sci. Rep., 2014, 4, 1–6 CAS
. - Y. Q. Li, R. Umer, A. Isakovic, Y. A. Samad, L. X. Zheng and K. Liao, RSC Adv., 2013, 3, 8849–8856 RSC
.
|
This journal is © The Royal Society of Chemistry 2014 |