DOI:
10.1039/C4RA09397F
(Paper)
RSC Adv., 2014,
4, 58964-58975
Control of cholesterol homeostasis by entero-hepatic bile transport – the role of feedback mechanisms†
Received
28th August 2014
, Accepted 22nd October 2014
First published on 24th October 2014
Abstract
Cholesterol homeostasis is achieved through a tight regulation between synthesis, dietary absorption, utilization of bile salts, and excretion. These processes are regulated through three known feedback mechanisms, namely auto-negative regulation of hepatic bile salt synthesis, and positive regulation of intestinal bile salts on cholesterol absorption and excretion. A model for entero-hepatic cholesterol metabolism in conjunction with dietary inputs for cholesterol was used to obtain insights into the role of the feedback. The analysis demonstrated the significance of the negative feedback in maintaining physiological levels of cholesterol. Furthermore, the positive feedback by the intestinal bile salts on the cholesterol absorption/excretion processes plays an important role in plasma cholesterol homeostasis. Under familial hypercholesterolemia (FH), perturbations in the hepato-intestinal reversible bile transport revealed that an increase in the transport of bile salts from the intestine to the liver decreased the hepatic cholesterol absorption rate. Under such a condition, a twofold decrease in intestinal bile salt transport resulted in an approximately 20% reduction in the plasma cholesterol level, thereby restoring it to normalcy. This suggests that the bile transport control strategy presents an alternative therapeutic method that can effectively reduce cholesterol absorption to attain cholesterol homeostasis.
Introduction
The liver plays a central role in the regulation and maintenance of cholesterol levels in the body.1 It houses the largest pool of free cholesterol, which is directed towards various sections of the body, depending upon their needs. The intestine is responsible for the uptake of dietary cholesterol2–5 and its excretion from the body and significantly interacts with the liver to maintain cholesterol homeostasis.6 High cholesterol levels in the blood can lead to deposition on the arterial walls, which thicken as a result of the accumulation. This leads to a chronic inflammatory response in the walls, causing atherosclerosis7,8 and cardiovascular diseases.9–11 The common causes of increased cholesterol levels in the plasma are high intake of dietary cholesterol and/or impaired cholesterol synthesis, absorption, and excretion.
Popular strategies for the treatment of elevated cholesterol include the use of statins, bile acid sequestrants, and cholesterol absorption inhibitors. Statins target the cholesterol biosynthesis pathway, which is responsible for most of the body's cholesterol needs.11–16 Suppressing this pathway reduces the de novo cholesterol synthesis, thus decreasing plasma cholesterol. Dietary cholesterol is absorbed in the intestine as well as cholesterol that is excreted with biliary secretions.2,4,17 Inhibitors of this process, such as ezetimibe, prevent the uptake of dietary cholesterol and are used to reduce cholesterol in the body. Abnormal plasma cholesterol levels may occur due to perturbations in the normal functioning of any of these processes. However, these processes are subject to drug therapies irrespective of the actual causes for elevated cholesterol. Despite statin treatments, the events leading to atherosclerosis have not been reasonably controlled, providing us with room for more potential therapies to control total plasma cholesterol levels.
A prominent process for cholesterol utilization in the body is the synthesis of bile acids from cholesterol. Bile acid sequestrants promote the excretion of bile salts and boost the conversion of cholesterol into bile, thus reducing the blood cholesterol levels.18–21 In animal studies,22–24 it has been observed that despite a higher dietary intake of cholesterol, plasma cholesterol levels do not substantially increase when there is a higher excretion of bile salts. Cholesterol homeostasis is strongly interconnected with bile metabolism, and the mechanisms by which these two pathways regulate each other are still unclear. Therefore, developing a mathematical model of the biological network comprising of the interactions (feedback) between cholesterol and bile metabolism in the entero-hepatic compartment and the analysis of the network is essential to decipher the control mechanisms prevailing in the structure. For this purpose, we resorted to the integration of two different models in the literature25,26 and analyzed the role of the feedback and bile transport in cholesterol homeostasis.
Here, we highlight the major interactions involved with entero-hepatic cholesterol metabolism. Intestinal cholesterol is absorbed in the liver, where it is converted into hepatic bile salts (HBS). Bile salts are known to act as carriers of cholesterol to facilitate micelle formation. A portion of the hepatic cholesterol pool is also transported to the blood to maintain plasma cholesterol levels. In the liver, an auto-negative feedback of bile salts regulates its own synthesis. The bile salts from the liver are transported into the intestinal bile salt (IBS) pool. The intestinal cholesterol (IC) pool has two inlets – biliary cholesterol release from the liver and dietary cholesterol, and two outlets – cholesterol excretion and cholesterol absorption back into the liver. Intestinal bile salts are required for facilitating the excretion as well as absorption of intestinal cholesterol, thereby positively regulating the two processes. Thus, three main feedback mechanisms may be identified in entero-hepatic cholesterol metabolism.
Cholesterol homeostasis mainly depends on the three primary feedback mechanisms prevalent in entero-hepatic cholesterol metabolism. The focus of the current study is to explore the mechanisms that play a role in cholesterol and bile homeostasis and suggest an alternative therapeutic strategy to control cholesterol levels. Towards this, we developed and utilized a mathematical model to obtain insights into the role of these feedback mechanisms on cholesterol homeostasis. The importance of the feedback mechanism in relation to varying dietary cholesterols is analyzed. The feedback analysis reveals that increased bile excretion has a dual role in reducing cholesterol levels – a lower steady-state level of intestinal bile salts reduces the strength of the positive feedback on cholesterol absorption into the liver, thereby reducing the overall plasma cholesterol levels; secondly, increased bile excretion boosts bile synthesis, thus increasing cholesterol utilization. The analysis indicated that the auto-negative feedback on the synthesis of the hepatic bile salts and the positive feedback on hepatic cholesterol absorption play an important role in regulating cholesterol levels. Therefore, entero-hepatic reversible bile transport is a possible site for perturbation/control to restore the plasma cholesterol levels under hypercholesterolemia.
Materials and methods
Model development and analysis
The whole body model for cholesterol metabolism developed by McAuley et al. (2012),26 which includes the intestine, liver, and peripheral and blood compartments, was adopted. From the literature,27–31 we know that other factors such as dietary nutrients and components along with lifestyle effects also play a significant role in determining cholesterol levels in the body. The effects of dietary components upon cholesterol metabolism were taken from the system dynamics model created by Demirezen & Barlas (2009).25 Fig. 1 shows the interactions in the entero-hepatic compartments in cholesterol metabolism. The rate equations applicable for the process of synthesis, absorption, and excretion of cholesterol and bile salts are listed in the ESI.†
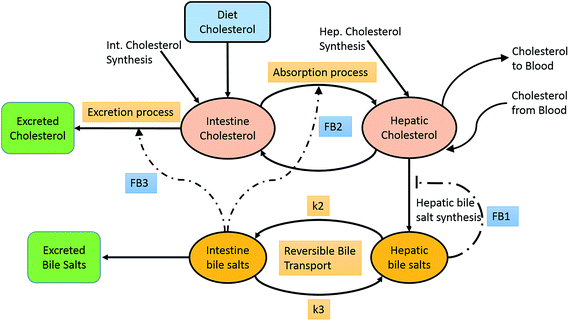 |
| Fig. 1 This module depicts the interactions between entero-hepatic cholesterol and bile metabolism. Dietary cholesterol is absorbed into the intestine, and from there, it is further transported to the liver. A portion of hepatic cholesterol is used for bile synthesis and for maintaining plasma cholesterol levels. FB1 represents the auto-negative feedback on hepatic bile salts, which are reversibly transported to the intestine. The intestinal bile salts assist in hepatic cholesterol absorption and intestinal cholesterol excretion, thereby resulting in positive feedback represented by FB2 and FB3, respectively. | |
The two models, based on different modeling frameworks, were integrated and analyzed in the present study. One of the models by Demirezen & Barlas (2009)25 used the principle of systems dynamics, wherein the concept of stock and flow variables is used to emulate a rough idea of the time dynamics of the model. The system dynamics approach allows us to break down extremely complex non-linear systems into systems with stocks, rate flows, and internal feedback loops, thus allowing for a preliminary analysis of the dynamics under limited mechanistic details. The authors used empirical data to express the rate kinetics of various reaction pathways. The systems dynamics model accounts for changes in cholesterol trends due to various external attributes such as effect of dietary components on cholesterol biosynthesis, effect of body weight, effect of exercise, etc. One of the limitations of this method is that it ignores the actual mechanisms occurring inside the system, as most rate expressions (flows) are based on empirical data with a black box assumption. Therefore, the impact of mechanistic changes in any variable upon the system's phenotypic response will not be reflected in the results. The other model we utilized was the mechanistic model by McAuley et al. (2012).26 This model implements the law of mass action and the Michaelis–Menten formulation to express the rate kinetics of every step involved in cholesterol metabolism. It accounts for the actual mechanisms in greater detail, but ignores the effect of external factors. These two models form the basis of the integrated model that we have presented (see the ESI†).
Our effort has been to merge the two models, thus being able to imitate not only the mechanistic processes with greater detail, but also the effects of the abovementioned external factors. To incorporate the effects of external factors into the current model, we translated the empirical data into convenient Hill expressions to fit the data points and included these effects in the model by McAuley et al. (2012).26 The model was simulated using the MATLAB program (http://www.mathworks.in) and the ODE15s solver. The algorithm used for obtaining the effect of feedback mechanisms and parameters is shown in Fig. 2.
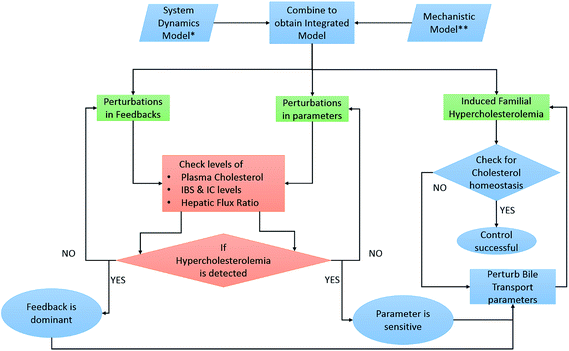 |
| Fig. 2 Flowchart representation of the algorithm followed in this study to investigate the effects of feedback and parametric perturbations in the mathematical model. The flowchart also shows the implementation of the Bile transport strategy, which uses the results of the feedback and parametric perturbations. * represents the systems dynamics model by Demirezen & Barlas (2009); ** represents the mechanistic model by McAuley et al. (2012). | |
The model incorporates the absorption of dietary cholesterol into the intestinal cholesterol pool, which is transported to the liver, where it becomes hepatic cholesterol that is then converted into hepatic bile salts. Furthermore, the model explicitly accounts for the following mechanisms. Negative feedback is maintained on the synthesis of bile salts. The bile salts from the liver are transported into the intestinal bile salt pool, and a reverse transport is also operational that helps to equilibrate the entero-hepatic bile salt balance. The intestinal bile salt pool regulates the outlets of intestinal cholesterol, which are excretion and absorption into the liver. A portion of hepatic cholesterol is secreted into the intestinal cholesterol pool through biliary cholesterol release.17,18,32,33 These interactions and transport processes collectively regulate cholesterol homeostasis. The model thus contains three feedback mechanisms: (1) auto-negative feedback on hepatic bile synthesis, called FB1; (2) positive feedback of intestinal bile salts on hepatic cholesterol absorption, called FB2; and (3) positive feedback of intestinal bile salts on intestinal cholesterol excretion, called FB3.
The developed model was used to obtain steady-state input–output relationships for plasma cholesterol, intestinal cholesterol, and intestinal bile salt levels in relation to varying levels of dietary cholesterol. The model was also used to obtain the effect of the three feedback mechanisms present in the network. To monitor the effect of perturbations on the net cholesterol accumulation in the liver, the ratio of intestinal cholesterol absorption to the sum of hepatic bile synthesis and biliary cholesterol release was recorded. Since this represents the ratio of cholesterol input to cholesterol output from the liver, changes in this ratio represent the effective plasma cholesterol levels. At every value of dietary cholesterol, a thousand simulations were run in which parameters of the module were randomly selected to be varied in a range of 10% on either side of their original values. The variations in the cholesterol values were recorded for all the simulations. The mean and standard deviation of total plasma cholesterol, intestinal cholesterol, intestinal bile salts, cholesterol flux to the blood, and input–output hepatic flux ratio were calculated. The extent of spread around the mean of these values at each dietary level was used as an indicator of the robustness due to the perturbation of each feedback.
Results
Steady-state response for varying dietary cholesterol
The developed model was simulated to obtain the dynamic concentration profile of the total blood cholesterol for different values of dietary cholesterol and for different network perturbations, as shown in Fig. 3. Under normal physiological conditions, the profile shows an invariant dynamic response, indicating homeostatic levels with total plasma cholesterol at 5.38 mM. The total plasma cholesterol is represented by the summation of low density lipoprotein cholesterol (LDLC), very low density lipoprotein cholesterol (VLDLC), intermediate density lipoprotein cholesterol (IDLC), and high density lipoprotein cholesterol (HDLC). Thus, the parameter values used in the model yield physiologically relevant steady-state values. Fig. 3(A) shows the behavior of total plasma cholesterol for different dietary cholesterol values. A 2-fold reduction in dietary cholesterol intake reduces total cholesterol to 5.25 mM, but a 4-fold increase in normal dietary cholesterol results in an increase of 20%. In Fig. 3(B), perturbing the negative feedback on bile synthesis causes total plasma cholesterol to rise by 15%, whereas perturbation of the intestinal bile salt feedback reduces the cholesterol value to 5.25 mM.
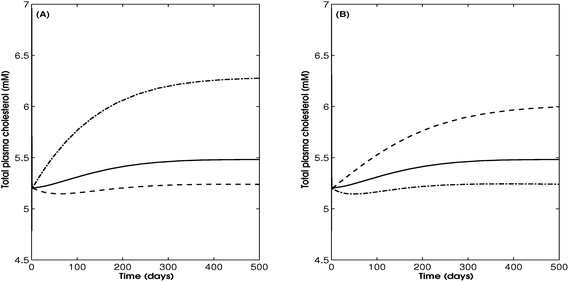 |
| Fig. 3 Dynamic response of total plasma cholesterol in response to (A) variation in dietary cholesterol values; the solid line represents normal dietary cholesterol, the dashed line represents a 2-fold reduction in dietary cholesterol, and the dashed-dotted line represents a 4-fold increase in dietary cholesterol, and (B) perturbations in different feedback regulations: the solid line represents normal phenotypic functioning, the dashed line represents the absence of FB1, and the dashed-dotted line represents absence of FB2 (as in Fig. 1). | |
Fig. 3 also shows the dynamic response for this module without the negative feedback on hepatic bile salt synthesis.34,35 The above analysis suggests that the negative feedback on hepatic bile salt synthesis is essential for regulating blood cholesterol levels. Removal of this negative regulation causes dominance of the indirect positive feedback loop (see Fig. 1), thereby leading to higher cholesterol levels.
The role of individual feedback mechanisms in homeostasis
The analysis of the model indicated that the system attains a monostable steady state at different initial conditions. In order to analyze the system performance at different levels of dietary cholesterol, the steady-state values were obtained and plotted against different dietary cholesterol levels with and without feedback perturbations. The system performance was evaluated using four metrics namely, total plasma cholesterol, intestinal cholesterol (IC) levels, intestinal bile salts (IBS), and the ratio of input to output fluxes for the hepatic cholesterol pool. Fig. 4 shows the effect of dietary cholesterol on the four metrics for three different cases – normal physiological conditions, absence of negative feedback on the hepatic bile salts (HBS), and the absence of positive feedback on intestinal cholesterol absorption by intestinal bile salts.
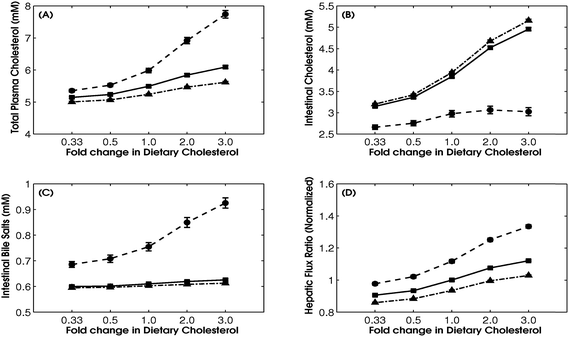 |
| Fig. 4 Perturbation analysis of different feedback mechanisms in the liver–intestine module. Removal of the HBS negative feedback increases the IBS concentration, thus increasing the flux of cholesterol absorption to the liver. This increases the total plasma cholesterol as well. Removal of the IBS positive feedback reduces the flux of cholesterol absorption responsible for cholesterol accumulation in the liver, which leads to a reduction in the hepatic and plasma cholesterol levels. The solid line with squares represents the normal phenotypic response, the dashed line with circles represents the absence of FB1, and the dashed-dotted line with triangles represents the absence of FB2 (as in Fig. 1). | |
The steady-state values are represented as the mean with a standard deviation of 1000 realizations through random multi-parametric perturbations. Under normal physiological levels, a three-fold increase in the dietary cholesterol (relative to approximately 304 mg day−1) raises the plasma cholesterol to approximately 6 mM, indicating the advent of hypercholesterolemia. This is accompanied by an increase in the intestinal cholesterol levels. However, the bile salt levels do not increase upon increasing the dietary cholesterol levels. Furthermore, as expected, the hepatic flux ratio increases by approximately 15% in the range studied. Parametric perturbation resulted in a negligible change in the standard deviations, indicating a robust behavior under physiological conditions.
On the contrary, removal of the negative feedback on the synthesis of hepatic bile salts (FB1) leads to an increase in plasma cholesterol levels, even with a normal dietary cholesterol intake of 304 mg day−1. In such an instance, a reduction in the dietary cholesterol levels can help to maintain healthy plasma cholesterol levels. The perturbation is accompanied by lower intestinal cholesterol due to a higher flux towards hepatic cholesterol, and hence, towards blood cholesterol (see Fig. 4(B) and (D)). The removal of the negative feedback results in a loss of robustness, indicated by a higher standard deviation around the mean when parametric perturbations are performed. This indicates that negative feedback (FB1) is essential for a robust performance by the network.
Next, we analyzed the role of positive feedback provided by intestinal bile salts on intestinal cholesterol absorption (FB2). Interestingly, upon removal of FB2, the total cholesterol levels decrease even at higher dietary cholesterol levels, with a maximum blood cholesterol concentration of 5.4 mM even when the dietary levels are 3 times higher than normal. This indicates that removal of the positive feedback essentially maintains the blood cholesterol levels independently of the dietary cholesterol levels in the range studied. This is also accompanied by lower intestinal bile salts and input/output flux ratio of the hepatic cholesterol pool. The lower flux towards hepatic cholesterol due to the absence of feedback ensures a higher cholesterol concentration in the intestine. The standard deviation due to parametric perturbation was the same as that for the normal physiological condition, indicating that feedback does not play a role in the robustness of the system. Thus, the presence of negative feedback (FB1) essentially controls the perturbation effects in the parameters.
The effect of multiple feedback on homeostasis
The previous results indicate that while negative feedback is essential for maintaining normal plasma cholesterol levels, the absence of positive feedback results in the maintenance of lower cholesterol levels. Since the individual knockout demonstrates a contrast in the module's response, we explored the effect of the simultaneous knockout of both feedback mechanisms (FB1 and FB2). Upon simultaneous removal of the feedback, the steady-state levels for the plasma and intestinal cholesterol were lower than that observed for normal physiological conditions (see Fig. 5). This indicates that positive feedback dominates over negative feedback. In such a situation, plasma cholesterol is low but the intestinal bile salts are higher than that which would be found under normal physiological conditions. Furthermore, the absence of negative feedback increases the standard deviation of the intestinal bile salt levels. Thus, the presence of the two feedback mechanisms is essential for maintaining robust homeostasis of both plasma cholesterol and intestinal bile salt levels.
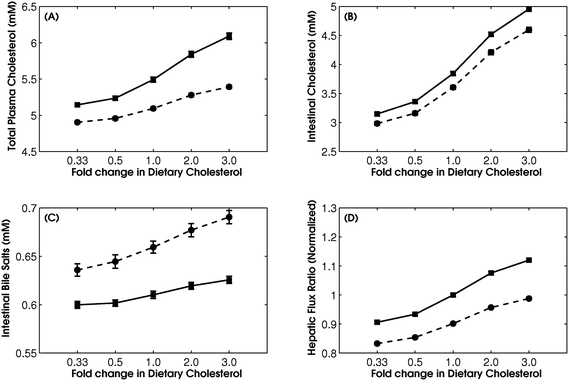 |
| Fig. 5 Simultaneous removal of HBS and IBS feedback. Removal of the HBS negative feedback leads to increased cholesterol due to an uninhibited rise of IBS concentrations. The effect of high levels of IBS on cholesterol absorption flux can be countered by removing the positive feedback of IBS on this process. This brings cholesterol levels back to healthy limits. The solid line with squares represents the normal phenotypic response, and the dashed line with circles represents the simultaneous removal of FB1 and FB2 (as in Fig. 1). | |
Perturbation in bile excretion
In order to evaluate the significance of the functioning of these two feedback mechanisms, we lowered the bile salt excretion flux by reducing the parameter k4 (see the model equation in the ESI†). In such a scenario, the steady-state plasma cholesterol is very high, indicating hypercholesterolemia (see Fig. 6(A)). However, the intestinal cholesterol levels marginally decrease, due to an increased flux towards hepatic cholesterol (see Fig. 6(D)). Upon removal of the two feedback mechanisms along with the faulty bile excretion, the total plasma cholesterol levels are invariant to dietary cholesterol levels. Although healthy physiological cholesterol levels are observed, the intestinal bile salt levels show a 16-fold increase as compared to the normal physiological range. Thus, this loss of bile homeostasis indicates the significance of these two feedback mechanisms. Furthermore, as observed before, the absence of the feedback mechanisms increases the standard deviations, indicating a sensitive response to perturbations.
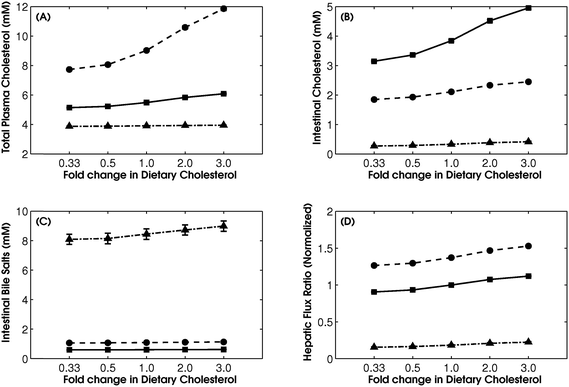 |
| Fig. 6 Feedback perturbations in the scenario of faulty bile excretion. The importance of the HBS and IBS feedback mechanisms is not apparent in (A)–(D) as far as cholesterol regulation is concerned. However, in (C), it is quite significant that absence of feedback leads to a deregulation of bile homeostasis. Due to the uninhibited synthesis of bile salts, an uncontrolled increase in bile concentrations is observed (dashed-dotted line), in contrary to the case where feedback exists (dotted line). A significant rise in standard deviation denotes a lack of robustness due to feedback removal ((C) dashed-dotted curve). The solid line with squares represents the normal phenotypic response, the dashed line with circles represents a scenario of faulty bile excretion, and the dashed-dotted line with triangles represents the absence of FB1 and FB2 in the scenario of faulty bile excretion (as in Fig. 1). | |
The role of feedback in cholesterol excretion
Next, we eliminated the feedback from the intestinal bile salts on the excretion of cholesterol from the intestine (FB3). It can be observed that feedback does not significantly affect the physiological response (see Fig. 7). The feedback removal, however, increases the variation in response to the parametric perturbations.
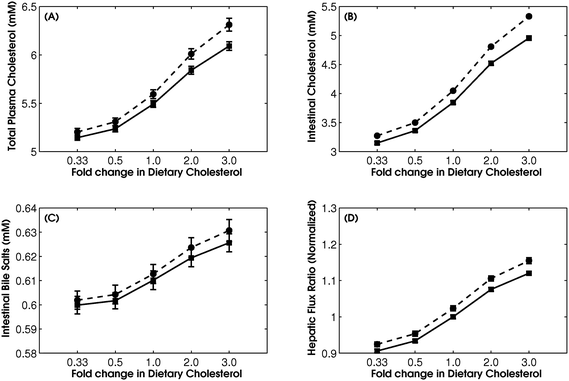 |
| Fig. 7 Absent IBS-positive feedback on cholesterol excretion. The impact of removal of this feedback is not significant, and the cholesterol levels show little deviation from the normal values. The solid line with squares represents the normal phenotypic response, and the dashed line with circles represents the removal of FB3 (as in Fig. 1). | |
The effect of increased bile transport
These studies indicate that positive regulation of intestinal bile salts over absorption (FB2) plays a more significant role than that over excretion (FB3). This motivated the idea for a control strategy that involves reducing intestinal bile salt concentrations to reduce liver and plasma cholesterol levels. The strategy is implemented by boosting the rate of bile salt transport from the intestine to the liver, which depletes the intestinal bile salt concentration.
Fig. 8 shows the steady-state response when the bile transport parameter from the intestine to the liver is increased by 1.5-fold, which results in reduced blood cholesterol levels. The reduced intestinal bile concentration causes a decrease in the intestinal cholesterol absorption, as seen in the hepatic flux ratio (see Fig. 8(D)). As this is an outflow for the intestinal cholesterol pool, the intestinal cholesterol levels increase. The decrease in absorption can be directly correlated to a decreased flux towards the blood. Thus, the above strategy of lowering the intestinal bile transport is a possible mechanism to regulate plasma cholesterol levels.
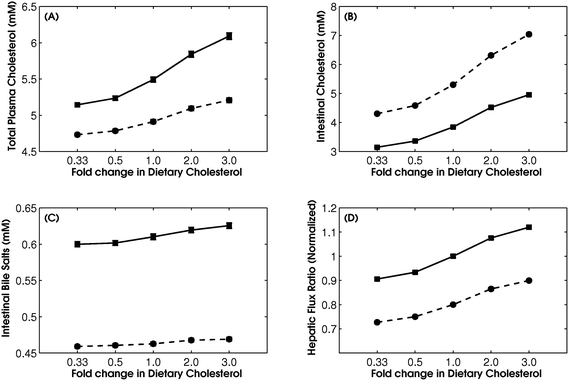 |
| Fig. 8 The effect of increased bile transport on cholesterol homeostasis. The increased bile transport reduces intestinal bile salts, thus reducing the excretion and absorption fluxes. Since absorption is affected more significantly, cholesterol returning to the liver is reduced, thus resulting in healthier plasma cholesterol values. The reduction in flux values leads to an accumulation of cholesterol in the intestine depicted in (B). The solid line with squares represents the normal phenotypic response, and the dashed line with circles represents the scenario with increased bile transport. | |
To test the efficacy of such a control strategy, a scenario of familial hypercholesterolemia (FH) was induced in the model. FH is a genetically acquired disease in which patients suffer from high cholesterol levels due to faulty low density lipoprotein (LDL) receptor metabolism.36 This causes a buildup of cholesterol levels in the blood, which is linked to a high risk of heart disease.9 In the model, FH was induced by reducing the rate of hepatic LDL receptor synthesis (khrs) to 0.8-fold the original value. In this case, the plasma cholesterol levels increased to 6.25 mM from 5.5 mM under normal dietary cholesterol levels, indicating FH. Under FH, if the bile transport from intestine to liver is increased, the plasma cholesterol levels decrease back to healthy limits (see Fig. 9(A)). As previously observed, the intestinal cholesterol levels increase from 3.9 mM to 6.9 mM (see Fig. 9(B)). Thus, healthy physiological homeostasis is regulated through intestinal bile transport in coordination with feedback.
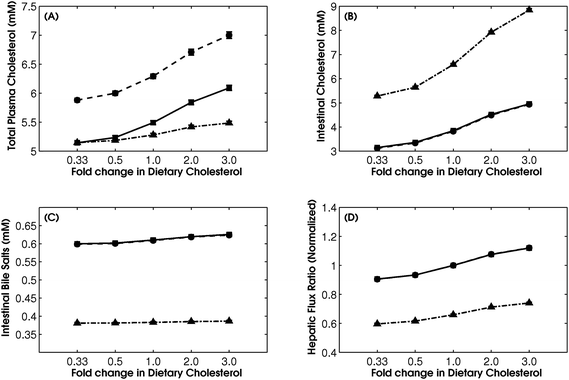 |
| Fig. 9 The significance of increased bile transport as a therapeutic method. The method of increased bile transport to decrease cholesterol levels is implemented in the scenario of familial hypercholesterolemia. This method helps to control cholesterol levels and lower them to healthy limits. The solid line with squares represents the normal phenotypic response, the dashed line with circles represents familial hypercholesterolemia, and the dashed-dotted line with triangles represents increased bile transport in a scenario of familial hypercholesterolemia. | |
The effect of parameter sensitivity
The positive feedback by intestinal bile salts on hepatic cholesterol absorption is represented by a Hill equation with a Hill coefficient of n = 2.5. The role of sensitivity was studied by decreasing feedback sensitivity, and this was accomplished by assuming the Hill coefficient value to be sub-sensitive (with n = 0.7). Fig. 10(A) plots the reaction rates of the hepatic cholesterol absorption for the two Hill coefficient values (dashed line for n = 0.7 and solid line for n = 2.5) and the cholesterol excretion rate (dashed-dotted line) at various intestinal bile salt conditions. In case of the sensitive positive feedback and at low concentrations of IBS, the rate of excretion is higher than the hepatic cholesterol absorption rate. This results in lower plasma cholesterol (see the phase-plane plot in Fig. 10(B)). If the sensitivity is lowered (i.e., n = 0.7), under a similar situation, the response shifts to a higher plasma cholesterol level due to a higher absorption rate over the excretion rate (see Fig. 10(B)). A complete reversal in the response is observed at higher IBS concentrations (see Fig. 10(C)). The results demonstrate the role of IBS concentrations and the sensitivity of the positive feedback regulation in steady-state plasma cholesterol levels.
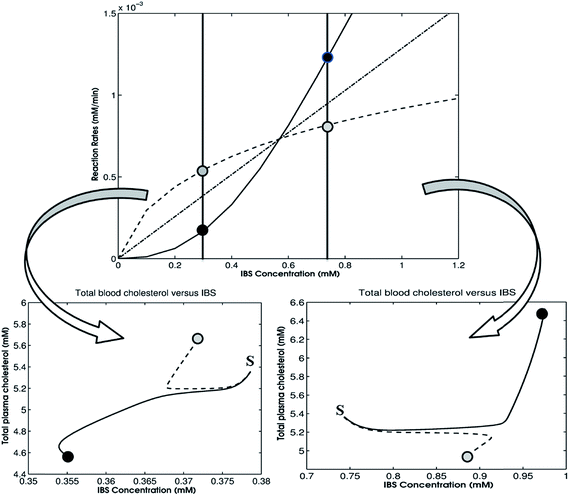 |
| Fig. 10 The flip response due to varying sensitivity in the intestinal cholesterol absorption. The solid line represents cholesterol absorption with n = 2.5, the dashed line represents absorption with n = 0.7, and the dashed-dotted line represents the reaction rate of cholesterol absorption. | |
Discussion
Apart from using statins to target some key enzymes of the biosynthesis pathway,16,37 few alternative measures have been proposed to control cholesterol metabolism. Moreover, statin therapy does not account for the actual causes of cholesterol increase, but rather, focuses on just the biosynthesis pathway. Thus, there exists the potential for elucidating different therapeutic mechanisms that can maintain cholesterol levels in the normal range by targeting the source of hypercholesterolemia. In our study, a control systems approach to understand cholesterol metabolism was applied to a mechanism-based kinetic model using previously published models.38–44 This system engineering approach deals with interactions between several modules in the network and the effect of each module on the overall performance of the network. In our study, we simulated an integrated model to obtain insights on the role of the major feedback mechanisms involved in entero-hepatic cholesterol and bile metabolism. We also ascertained the effect of perturbation on the bile transport mechanism as a potential control point in cholesterol homeostasis. The major insights obtained regarding the feedback perturbations in the model are discussed below.
The role of individual feedback mechanisms in cholesterol homeostasis
In this study, we have analyzed the importance of various feedback regulations in the entero-hepatic bile transport module on the overall cholesterol homeostasis. Identifying the mechanisms responsible for elevated cholesterol consequently allows us to suggest therapeutic strategies to reduce cholesterol levels to a healthy range. The knockout of the negative feedback on hepatic bile salts enhanced the effect of the positive feedback, thereby increasing the plasma cholesterol levels. It has been reported that hypercholesterolemia is observed in patients suffering from defects in the FXR gene45,46 that is responsible for employing the negative feedback mechanism.47,48 Furthermore, the analysis also highlighted the significance of the feedback mechanisms to maintain robustness in the system by demonstrating a higher standard deviation of the parametric perturbations upon removal of the feedback. The knockout of the feedback of intestinal bile salts reduced the absorption flux for the intestine, thereby reducing the cholesterol levels both in the liver and plasma. However, the knockout of the positive feedback on excretion of intestinal cholesterol was not significant relative to the feedback on the absorption process.
The role of multiple feedback mechanisms in cholesterol homeostasis
The simultaneous knockout of the positive feedback of IBS and negative feedback of HBS demonstrated no significant difference in plasma cholesterol as compared to normal. The cholesterol absorption into the liver is positively regulated by the intestinal bile salts. When the bile salts uncontrollably increase due to the absence of the HBS negative feedback, the flux to the liver increased, causing an increase in cholesterol levels. Hence, removal of the positive regulation of IBS on cholesterol absorption can help counter the pro-atherogenic effects of removing the negative regulation on bile synthesis. Therefore, the negative regulation of HBS and positive regulation of IBS exhibit a balance in action, i.e., there is a need for both to exist together, and if one is perturbed, the other needs to be perturbed as well so as to maintain homeostasis. Thus, the intestinal cholesterol absorption plays a crucial role in determining the cholesterol levels in the body at steady state. As more refined therapeutic strategies are investigated to control cholesterol, drugs can be designed to target this particular process and direct the net flux of cholesterol transport towards the liver or excretion, thus effectively controlling the increase or decrease of cholesterol.
The role of bile excretion
Because the collective removal of positive and negative feedback mechanisms did not demonstrate any significant change in cholesterol levels, a pertinent question arises regarding the roles of the two feedback mechanisms to regulate cholesterol homeostasis. To explore this, a perturbation in bile excretion was simulated. The simulations demonstrated an increase in cholesterol levels due to an increase in bile salt concentrations (see Fig. 6). In the perturbed bile excretion case, the removal of the two feedback mechanisms restored the plasma cholesterol levels. Essentially, the knockout of both feedback mechanisms resulted in a decoupling of the cholesterol and bile pathways in the liver–intestine module, which eliminated the regulatory effect of bile on cholesterol levels. However, feedback removal caused an increase in the bile salt levels. Moreover, the increased standard deviations in the bile levels indicate a loss of robustness in bile homeostasis. Thus, the joint action of the positive feedback of IBS and negative feedback of HBS ensures control of the bile salt concentrations in the body, and hence, is necessary for bile homeostasis.
The effect of increased bile transport
The difference in regulatory strengths of IBS on absorption and excretion processes motivated the idea for a strategy in which cholesterol levels can be controlled by altering the concentration of intestinal bile salts. This was implemented by increasing the rate of bile transport from the intestine to the liver, thereby reducing the IBS concentration. As shown in Fig. 8, this results in a lower input–output hepatic flux ratio, leading to a reduction of the cholesterol pool in the liver and a subsequent reduction in plasma cholesterol. Similar effects can be obtained by decreasing the liver-to-intestine bile transport. Due to reduced rates of absorption and excretion, the net outflow of cholesterol from the intestine is decreased, resulting in an accumulation of intestinal cholesterol. However, the accumulation is not significant and can be mitigated by increasing the dietary fiber content, which increases the excretion of intestinal cholesterol. To test the efficacy of this strategy, an increase in bile transport was implemented in a case of familial hypercholesterolemia. In a hypercholesterolemia case due to genetic defects, the bile transport perturbation resulted in restoration of normalcy in cholesterol levels (see Fig. 9). This suggests that increased bile transport from the intestine to the liver can be used as a control strategy to alleviate hypercholesterolemia.
A review article by Charach et al.49 raises the point that improper excretion could possibly lead to a cholesterol buildup, thus causing coronary diseases associated with it. In fact, a popularly suggested cure for lowering cholesterol involves including flaxseed in the patient's diet. This seed has a high dietary fiber content that binds to the cholesterol in the intestine, preventing its absorption and directing it to the excretion system. Other remedies such as Chinese green tea and dietary plant proteins lower the cholesterol content by increasing its excretion. Similar to a purge performed in a chemical plant to balance a buildup in the concentration of certain compounds, metabolic processes in a healthy human also work towards balancing a cholesterol buildup by purging.
The effect of feedback sensitivity
The increased bile transport trends displayed a dependence on the sensitivity of positive feedback on the intestinal cholesterol absorption. The intestinal cholesterol pool is maintained by three major fluxes – cholesterol excretion, absorption into the liver (outflow), and biliary cholesterol release from the liver (inflow). The balance of the intestinal cholesterol pool is mainly governed by the nature of the kinetics of cholesterol excretion and absorption. Here, in our model, the excretion process is represented by mass action kinetics, whereas the positive regulation of bile salts on the absorption process is represented by a Hill function. To assess the importance of the positive regulation sensitivity, we varied the Hill function from sub-sensitive to sensitive and observed the variation in plasma cholesterol. Interestingly, it was noted that there was a flip in the cholesterol level responses. The Hill function was varied from sensitive and sub-sensitive regulation (see Fig. 10(B)). In the sub-sensitive case, increasing the bile transport resulted in an increase in the plasma cholesterol, contrary to that observed for the sensitive case, where a substantial decrease was noted. This was essentially due to the relatively higher transport rate in the case of sub-sensitive regulation of absorption, which was higher than that of the excretion flux that follows mass action kinetics. However, in the sensitive case, the mass action kinetics of the excretion process would dominate over the sensitively regulated cholesterol absorption in the range of intestinal bile salts less than its own half saturation constant for the positive regulation (i.e., its Km value). Therefore, the differences in the kinetic nature of the absorption and excretion rates of intestinal cholesterol and its regulation by IBS can be exploited to obtain the desired levels of cholesterol. It is expected that in a large population with different lifestyles, the parameter space for a flux (Km,n) may vary within a certain range. Hence, differences in the kinetics of these fluxes can lead to a different response in a population.
Summary
Our overall rigorous analysis of the hepato-intestinal bile transport systems demonstrates significant diversity in the ways they can regulate plasma cholesterol levels. In the case of feedback studies, the negative feedback on bile synthesis tightly controls the bile salts to ensure that liver cholesterol does not accumulate. The positive feedback on intestinal cholesterol absorption maintains a balance between the amount of cholesterol leaving the system and that returning to the liver, and perturbing this balance can shift the system in either direction. The positive and the negative feedback mechanisms display a tender balance in their role, as disturbing one requires disturbing the other for a subsequent entry into healthy limits. The combined absence of both, while aiding the control of cholesterol homeostasis, is detrimental to bile salt homeostasis, giving us an indication of their action. Modifying the bile transport between the liver and intestine can be therapeutically used to reduce plasma cholesterol levels, and this method was shown to be extremely efficient in controlling familial hypercholesterolemia. The balance of the fluxes in the intestinal cholesterol transport plays an even greater importance in such a perturbation, due to the sensitivity of the positive feedback that determines the homeostatic state of the system.
The current study highlights the significance of the feedback mechanisms and excretion rates in cholesterol homeostasis. The parameter space influencing these mechanisms would play a significant role in maintaining the homeostatic state. Perturbations in the parametric space may thus shift the homeostatic behavior. The heterogeneity in the parametric space in human populations may indicate the susceptibility or the robustness of an individual towards hypercholesterolemia. This study indicates that these mechanisms may be possible therapeutic targets for regulation.
References
- J. M. Dietschy, S. D. Turley and D. K. Spady, Role of liver in the maintenance of cholesterol and low density lipoprotein homeostasis in different animal species, including humans, J. Lipid Res., 1993, 34, 1637–1659 CAS
. - S. W. Altmann, et al. Niemann-Pick C1 Like 1 protein is critical for intestinal cholesterol absorption, Science, 2004, 303, 1201–1204 CrossRef CAS PubMed
. - P. A. Dawson and L. L. Rudel, Intestinal cholesterol absorption, Curr. Opin. Lipidol., 1999, 10(4), 315–320 CrossRef CAS PubMed
. - E. Levy, et al. Intestinal cholesterol transport proteins: an update and beyond, Curr. Opin. Lipidol., 2007, 18(3), 310–318 CrossRef CAS PubMed
. - D. Q.-H. Wang, Regulation of Intestinal Cholesterol Absorption, Annu. Rev. Physiol., 2007, 69, 221–248 CrossRef CAS PubMed
. - A. H. Lichtenstein, Intestinal Cholesterol Metabolism, Ann. Med., 1990, 22, 49–52 CrossRef CAS PubMed
. - P. Amarenco, J. Labreuche and P.-J. Touboul, High-density lipoprotein-cholesterol and risk of stroke and carotid atherosclerosis: a systematic review, Atherosclerosis, 2008, 196, 489–496 CrossRef CAS PubMed
. - L. a. Solberg and J. P. Strong, Risk factors and atherosclerotic lesions. A review of autopsy studies, Arterioscler., Thromb., Vasc. Biol., 1983, 3, 187–198 CrossRef CAS
. - M. a. Austin, C. M. Hutter, R. L. Zimmern and S. E. Humphries, Familial hypercholesterolemia and coronary heart disease: a HuGE association review, Am. J. Epidemiol., 2004, 160, 421–429 CrossRef PubMed
. - Scandinavian Simvastatin Survival Study Group, Randomised trial of cholesterol lowering in 4444 patients with coronary heart disease: the Scandinavian Simvastatin Survival Study (4S), Lancet, 1994, 344, 1383–1389 Search PubMed
. - J. Shepherd, et al. Prevention of Coronary Heart Disease with Pravastatin in Men with Hypercholesterolemia, N. Engl. J. Med., 1995, 333, 1301–1308 CrossRef CAS PubMed
. - D. B. Ott and P. A. Lachance, Biochemical controls of liver cholesterol biosynthesis, Am. J. Clin. Nutr., 1981, 34, 2295–2306 CAS
. - J. Thomas, T. P. Shentu and D. K. Singh, Cholesterol: Biosynthesis, Functional Diversity, Homeostasis and Regulation by Natural Products, Biochemistry, 2012, 419–441 CAS
. - G. Silbernagel, et al. Cholesterol synthesis is associated with hepatic lipid content and dependent on fructose/glucose intake in healthy humans, Exp. Diabetes Res, 2012, 2012, 361863 CrossRef PubMed
. - E. Reihnér, et al. Influence of pravastatin, a specific inhibitor of HMG-CoA reductase, on hepatic metabolism of cholesterol, N. Engl. J. Med., 1990, 323, 224–228 CrossRef PubMed
. - D. C. Bauer, HMG CoA reductase inhibitors and the skeleton: a comprehensive review, Osteoporosis Int., 2003, 14, 273–282 CrossRef CAS PubMed
. - R. E. Temel, et al. Hepatic Niemann-Pick C1-like 1 regulates biliary cholesterol concentration and is a target of ezetimibe, J. Clin. Invest., 2007, 117, 1968–1978 CrossRef CAS PubMed
. - E. Sehayek, et al. Biliary cholesterol excretion: a novel mechanism that regulates dietary cholesterol absorption, Proc. Natl. Acad. Sci. U. S. A., 1998, 95, 10194–10199 CrossRef CAS
. - A. Dikkers and U.-J. Tietge, Biliary cholesterol secretion: more than a simple ABC, World J. Gastroenterol., 2010, 16, 5936–5945 CAS
. - M. Meissner, et al. Bile acid sequestration normalizes plasma cholesterol and reduces atherosclerosis in hypercholesterolemic mice. No additional effect of physical activity, Atherosclerosis, 2013, 228, 117–123 CrossRef CAS PubMed
. - H. R. Casdorph, Hypercholesteremia. Treatment with cholestyramine, a bile acid sequestering resin, Calif. Med., 1967, 106, 293–295 CAS
. - H. B. Lofland, T. B. Clarkson, R. W. S. Clair and N. D. M. Lehner, Studies on the regulation of plasma cholesterol levels in squirrel monkeys of two genotypes, J. Lipid Res., 1972, 13, 39–47 CAS
. - B. G. Bhat, et al. Inhibition of ileal bile acid transport and reduced atherosclerosis in apoE-/- mice by SC-435, J. Lipid Res., 2003, 44, 1614–1621 CrossRef CAS PubMed
. - S. M. Post, R. de Crom, R. van Haperen, A. van Tol and H. M. G. Princen, Increased fecal bile acid excretion in transgenic mice with elevated expression of human phospholipid transfer protein, Arterioscler., Thromb., Vasc. Biol., 2003, 23, 892–897 CrossRef CAS PubMed
. - E. M. Demirezen and Y. Barlas, A simulation model for blood cholesterol dynamics and related disorders, in 27th Int. Conf. Syst. Dyn. Soc., Albuquerque, New Mex. USA, sn26, 30, 2009 Search PubMed
. - M. T. McAuley, D. J. Wilkinson, J. J. L. Jones and T. B. L. Kirkwood, A whole-body mathematical model of cholesterol metabolism and its age-associated dysregulation, BMC Syst. Biol., 2012, 6, 130 CrossRef CAS PubMed
. - L. Brown, B. Rosner, W. W. Willett and F. M. Sacks, Cholesterol-lowering effects of dietary fiber: a meta-analysis, Am. J. Clin. Nutr., 1999, 69, 30–42 CAS
. - D. M. Hegsted, L. M. Ausman, J. A. Johnson and G. E. Dallal, Dietary fat and serum lipids: an evaluation of the experimental data, Am. J. Clin. Nutr., 1993, 57, 875–883 CAS
. - D. Kritchevsky, Metabolic effects of dietary fiber, West. J. Med., 1979, 130, 123–127 CAS
. - R. P. Mensink, P. L. Zock, A. D. M. Kester and M. B. Katan, Effects of dietary fatty acids and carbohydrates on the ratio of serum total to HDL cholesterol and on serum lipids and apolipoproteins: a meta-analysis of 60 controlled trials, Am. J. Clin. Nutr., 2003, 77, 1146–1155 CAS
. - G. Schonfeld, et al. Effects of dietary cholesterol and fatty acids on plasma lipoproteins, J. Clin. Invest., 1982, 69, 1072–1080 CrossRef CAS
. - P. Mardones, et al. Hepatic cholesterol and bile acid metabolism and intestinal cholesterol absorption in scavenger receptor class B type I-deficient mice, J. Lipid Res., 2001, 42, 170–180 CAS
. - L. Yu, et al. Expression of ABCG5 and ABCG8 Is Required for Regulation of Biliary Cholesterol Secretion, J. Biol. Chem., 2004, 280, 8742–8747 CrossRef PubMed
. - E. L. Forker, Mechanisms of Hepatic Bile Formation, Annu. Rev. Physiol., 1977, 39, 323–347 CrossRef CAS PubMed
. - D. W. Russell, The Enzymes, Regulation, And Genetics Of Bile Acid Synthesis, Annu. Rev. Biochem., 2003, 72, 137–174 CrossRef CAS PubMed
. - a. D. Marais, Familial hypercholesterolaemia, Clin. Biochem. Rev., 2004, 25, 49–68 Search PubMed
. - I. Buhaescu and H. Izzedine, Mevalonate pathway: a review of clinical and therapeutical implications, Clin. Biochem., 2007, 40, 575–584 CrossRef CAS PubMed
. - B. S. Bhattacharya, Mathematical Modelling of Low Density Lipoprotein Metabolism. Intracellular Cholesterol Regulation, 2011 Search PubMed
. - N. a. Mazer and M. C. Carey, Mathematical model of biliary lipid secretion: a quantitative analysis of physiological and biochemical data from man and other species, J. Lipid Res., 1984, 25, 932–953 CAS
. - N. C. A. van de Pas, A physiologically based kinetic model for the prediction of plasma cholesterol concentrations in mice and man, 2011 Search PubMed
. - T. Pearson, et al. Mathematical modelling of competitive LDL/VLDL binding and uptake by hepatocytes, J. Math. Biol., 2009, 58, 845–880 CrossRef CAS PubMed
. - A. V. Ratushny & V. A. Likhoshvai, Mathematical modeling of the gene network controlling homeostasis of intracellular cholesterol, This issue, 2006 Search PubMed
. - J. Sy, A Model of Cholesterol Metabolism and Transport, 2008 Search PubMed
. - J. Lu, K. Hübner, M. N. Nanjee, E. a. Brinton and N. a. Mazer, An in-silico model of lipoprotein metabolism and kinetics for the evaluation of targets and biomarkers in the reverse cholesterol transport pathway, PLoS Comput. Biol., 2014, 10, e1003509 Search PubMed
. - T. Lundasen, Studies on the hormonal regulation of bile acid synthesis, 2007 Search PubMed
. - C. R. Pullinger, et al. Human cholesterol 7alpha-hydroxylase (CYP7A1) deficiency has a hypercholesterolemic phenotype, J. Clin. Invest., 2002, 110, 109–117 CrossRef CAS
. - J. Y. L. Chiang, Bile acids: regulation of synthesis, J. Lipid Res., 2009, 50, 1955–1966 CrossRef CAS PubMed
. - T. Lu, Molecular Basis for Feedback Regulation of Bile Acid Synthesis by Nuclear Receptors, Mol. Cell, 2000, 6, 507–515 CrossRef CAS
. - G. Charach, A. Rabinovich, O. Argov, M. Weintraub and P. Rabinovich, The role of bile Acid excretion in atherosclerotic coronary artery disease, Int. J. Vasc. Med., 2012, 2012, 949672 Search PubMed
.
Footnotes |
† Electronic supplementary information (ESI) available. See DOI: 10.1039/c4ra09397f |
‡ SM and PRS contributed equally to this work. |
|
This journal is © The Royal Society of Chemistry 2014 |
Click here to see how this site uses Cookies. View our privacy policy here.