DOI:
10.1039/C4RA08564G
(Paper)
RSC Adv., 2014,
4, 49831-49841
Protective effect of Artemisia campestris extract against aspirin-induced gastric lesions and oxidative stress in rat
Received
12th August 2014
, Accepted 18th September 2014
First published on 18th September 2014
Abstract
The present study aims at evaluating the antiulcer and antioxidant effect of Artemisia campestris aqueous extract (ACAE) as well as the mechanism of action involved in such gastroprotection. The use of LC/MS allowed the identification of 11 phenolic compounds and the colorimetric analysis demonstrated that the ACAE exhibited an important in vitro antioxidant activity. We first showed that in vivo ACAE protected against macroscopic and histological changes induced by aspirin in stomach mucosa. Aspirin administration was accompanied by an oxidative stress status assessed by an increase in malondialdehyde (MDA) level, a decrease in the content of sulfhydryl –(SH) groups and a depletion of antioxidant enzyme activities such as superoxide dismutase (SOD), catalase (CAT) and glutathione peroxidase (GPx). Pre-treatment with ACAE protected against aspirin-induced gastric oxidative stress. More importantly, aspirin administration increased plasma and tissue hydrogen peroxide (H2O2), free iron and calcium levels, while the ACAE pre-treatment reversed all the effects of aspirin-induced intracellular mediators. In conclusion, we suggest that Artemisia campestris aqueous extract has potent antiulcer and antioxidant properties. This gastroprotection offered by ACAE might be related partly to the safety of sulfhydryl group as well as its opposite effect on some intracellular mediators such as hydrogen peroxide, free iron and calcium.
1. Introduction
Ulcer is a multifactorial gastrointestinal disorder caused by the loss of balance between aggressive and defensive factors of the gastric and duodenal mucosa.1 The nonsteroidal anti-inflammatory drugs (NSAIDs), Helicobacter pylori, stress, and steroids, are the aggressive factors that increase the risk of gastric ulcer formation.2–4 NSAIDs are widely used for their analgesic, anti-pyretic and anti-inflammatory effects.5,6 However, these drugs are in fact associated with gastrointestinal toxicity including ulcer formation.6,7 The pathogenesis of NSAIDs-induced gastric ulceration is accompanied by lower mucus and bicarbonate secretion, decreased mucosal blood flow, neutrophil infiltration, alteration of microvascular structures, and increased acid and pepsinogen secretion.8,9 In addition, the pathogenesis of NSAIDs-induced ulcers, such as aspirin, is also accompanied by an increase in reactive oxygen species (ROS).10 These in turn induce lipid peroxidation and antioxidant enzymes inhibition, which are the basis of many toxicological and pathological processes.11 However, plant extracts, known for their richness in phenolic compounds and antioxidant ability, are mainly used by researchers to produce new drugs and obtain promising results in the treatment of gastric ulcers.12
Artemisia campestris L. is a medicinal plant used for centuries in traditional folk medicine in many countries in the world including Tunisia, and is well-known for its beneficial effects in the treatment of gastrointestinal disorders, including peptic ulcer.13 In addition, this plant is well-known as a versatile source of components with bioactive properties. Because of its antioxidant properties,14 A. campestris presents many beneficial health effects such as hepatoprotective,15 renoprotective,14 antidiabetic16 and anticancer13 activities. Recently, Houicher et al.17 demonstrated that ethanolic extract obtained from A. campestris prevents food spoilage and extends the shelf life of vacuum-packed refrigerated sardines (Sardina pilchardus).
The aim of the present study is to evaluate the antioxidant properties of an aqueous extract of A. campestris as well as its protective effect against aspirin-induced gastric ulceration in rats. We also study the implication of sulfhydryl groups and some intracellular mediators in such gastroprotection.
2. Materials and methods
2.1. Chemicals
Epinephrine, bovine catalase, 2-thio-barbituric acid (TBA) and butylated hydroxytoluene (BHT) were purchased from Sigma chemicals Co (Germany). All the other chemicals used were of analytical grade.
2.2. Preparation of Artemisia campestris aqueous extract
Artemisia campestris plants were cultivated in the region of Metlaoui (South-western Tunisia) during August 2012 and were identified by Mrs Mouhiba Ben-Naceur, Professor of taxonomy in the Higher Institute of Biotechnology of Béja – Tunisia. Plant material was subsequently dried in an incubator at 50 °C for 72 hours and powdered in an electric blender. The extract was prepared in double distilled water (1/5; w/v) under magnetic agitation for 24 hours and centrifuged at 10
000 g for 10 min to eliminate insoluble material. Supernatant was lyophilised and stored at −80 °C until use.
2.3. Characterisation of phenolic compounds by HPLC-PDA-ESI-MS/MS
The lyophilised extract of A. campestris (1 mg mL−1) was dissolved in methanol18 and analyzed by LC-MS/MS using an Agilent Series 1100 LC system (Agilent Technologies, Palo Alto, Ca, USA) equipped with a photodiode array detector (PDA) and a triple quadrupole mass spectrometer type Micromass Autospec Ultima Pt (Kelso, UK) interfaced with an ESI ion source. Separation was achieved using a Superspher® 100 (12.5 cm × 2 mm i.d., 4 μm, Agilent Technologies, Rising Sun, MD) at 45 °C. Samples (20 μL) were eluted through the column with a gradient mobile phase consisting of A (0.1% acetic acid) and B (acetonitrile) with a flow rate of 0.25 mL min−1. The following multi-step linear solvent gradient was employed: 0–5 min, 2% B, 5–75 min, 88% B, 75.1–90 min, 2% B.
PDA was detected in the 200–400 nm wavelength range and the mass spectra were recorded in negative ion mode under the following operating conditions: capillary voltage, 3.2 kV; cone voltage, 115 V; probe temperature, 350 °C; ion source temperature, 110 °C. The spectra were acquired in an m/z range of 150–750 amu.
The identification of phenolic compounds was based on co-chromatography with authentic standards, when available. PDA and mass spectra were used to affirm the identity of compounds previously reported in the literature.19–21 The content percentage of each component in the crude extract was determined by a UV chromatogram at 210 nm.18
2.4. Free radical-scavenging activity on 2,2-diphenyl-1-picrylhydrazyl (DPPH)
The antioxidant capacity of CDE was determined using DPPH radical-scavenging activity, as previously described by Grzegorczyk et al.22 Briefly, various concentrations of ACAE (20, 50, 100, 150, and 200 μg mL−1) were added to 1 mL of a 0.1 mM methanol solution of DPPH and incubated at 27 °C for 30 min. The optical density of the sample was quantified at 517 nm. DPPH radical-scavenging activity (RSA), expressed as percentage, was estimated by the following formula:
Ascorbic acid in the same concentration as the test extract was used as a reference molecule.
All the analyses were executed in triplicate. The efficacy concentration 50 (EC50) value was determined as the concentration (in μg mL−1) of the compound required to scavenge 50% of the DPPH radical.
2.5. Animals and treatment
Adult male Wistar rats (weighing 200–220 g; housed five per cage) and adult male Swiss Albino mice (weighing approximately 25 g; housed ten per cage) were purchased from the Pasteur Institute of Tunis and were used in accordance with the local ethics committee of Tunis University for the use and care of animals in accordance with the NIH recommendations. The rats were provided with food (standard pellet diet, Badr Utique-TN) and water ad libitum and were maintained in an animal house at controlled temperature (22 ± 2 °C) with a 12 hours light–dark cycle. The rats were divided into seven groups of 10 animals each. Groups 1 and 2 served as controls and had bidistilled water (5 mL kg−1, b.w., p.o.). Groups 3, 4, and 5 were pre-treated with various doses of ACAE (100, 200 and 400 mg kg−1, b.w. p.o.), while groups 6 and 7 were pre-treated with famotidine (20 mg kg−1, b.w. p.o.) and caffeic acid (50 mg kg−1, b.w. p.o.), respectively. Rats were fasted for 24 h prior to the administration of ACAE or reference molecules.
After 60 min, each animal, except group 1, received aspirin (300 mg kg−1, b.w.) by oral administration. Two hours later, rats were sacrificed. Blood was collected in heparinized tubes. After centrifugation at 3000 g for 15 min, free iron, H2O2 and calcium were determined from free plasma.
2.6. Acute toxicity study
A. campestris aqueous extract in the dose range of 12.5, 25, 50, 100, 200, 400, 800, 1600 and 3200 mg kg−1 was orally administrated to different groups of mice (n = 10). Animals were examined every 30 min for 4 h and then occasionally for an additional period of 8 h. After 24 h, the mortality was recorded. The mice were also observed for other signs of toxicity such as motor co-ordination, righting reflex and respiratory changes.
2.7. Evaluation of gastric mucosal damage
The stomach of each animal was removed and opened along its greater curvature. The tissue was gently rinsed with 0.9% NaCl. The lesions in the gastric mucosa were macroscopically examined, and the photographs of hemorrhagic erosions were acquired with a Photometrics Quantix digital camera. Ulcer indexes were determined as the sum of the lengths of the entire gastric lesions (in mm2). Two independent, blinded observers performed the measurements of lesion lengths.
2.8. Stomach mucosa preparation
After the macroscopic analyses, the stomach mucosa was rapidly excised and homogenized in phosphate buffer saline (KH2PO4/K2HPO4, 50 mM, pH 7.4) with Potter-Elvehjem homogenizer. After centrifugation at 10
000 g for 10 min at 4 °C, supernatant was used for the biochemical determination of protein, free iron, H2O2, calcium, SH– groups, MDA and antioxidant enzyme activities.
2.9. Histopathological analysis
Immediately after sacrifice, small pieces of stomach were harvested and washed with ice cold saline. Tissue fragments were then fixed in 10% neutral buffered formalin solution, embedded in paraffin and used for histopathological examination. 5 μm thick sections were cut, deparaffinized, hydrated and stained with hematoxylin and eosin (HE). The gastric sections were examined in blind fashion for all the treatments.
2.10. Lipid peroxidation measurement
Gastric mucosa lipid peroxidation was determined by MDA measurement according to the double heating method.23 Briefly, aliquots from gastric mucosa homogenates were mixed with BHT–TCA solution containing 1% BHT (w/v) dissolved in 20% TCA (w/v) and centrifuged at 1000 g for 5 min at 4 °C. Supernatant was blended with solution containing (0.5 N HCl 120 mM TBA in 26 mM Tris) and then heated at 80 °C for during 10 min. After cooling, the absorbance of the resulting chromophore was determined at 532 nm using a UV-visible spectrophotometer (Beckman DU 640B). MDA levels were determined by using an extinction coefficient for MDA–TBA complex of 1.56 × 105 M−1 cm−1.
2.11. Antioxidant enzyme activity assays
SOD activity was determined using modified epinephrine assays.24 At alkaline pH, superoxide anion O2− causes the autoxidation of epinephrine to adenochrome; while competing with this reaction, SOD decreased the adenochrome formation. One unit of SOD is defined as the amount of extract that inhibits the rate of adenochrome formation by 50%. Enzyme extract was added in 2 mL reaction mixture containing 10 μL of bovine catalase (0.4 U μL−1), 20 μL epinephrine (5 mg mL−1) and 62.5 mM sodium carbonate/bicarbonate buffer of pH 10.2. Changes in absorbance were recorded at 480 nm.
CAT activity was assessed by measuring the initial rate of H2O2 disappearance at 240 nm.25 The reaction mix contained 33 mM H2O2 in 50 mM phosphate buffer at pH 7.0, and the activity of CAT was calculated using the extinction coefficient of 40 mM−1 cm−1 for H2O2.
GPx activity was quantified by the procedure reported by Flohé and Günzler.26 Briefly, 1 mL of the reaction mixture containing 0.2 mL of liver supernatant, 0.2 mL of phosphate buffer (0.1 M, pH = 7.4), 0.2 mL of GSH (4 mM) and 0.4 mL of H2O2 (5 mM) was incubated at 37 °C for 1 min, and the reaction was stopped by adding 0.5 mL TCA (5%, w/v). After centrifugation at 1500 g for 5 min, an aliquot (0.2 mL) from the supernatant was combined with 0.5 mL of phosphate buffer (0.1 M, pH = 7.4) and 0.5 mL DTNB (10 mM), and the absorbance was determined at 412 nm. GPx activity was expressed as nmol of GSH consumed/min/mg of protein.
2.12. Thiol groups measurement
The total concentration of thiol groups (–SH) was performed according to the Ellman's method.27 Briefly, aliquots from stomach mucosa were mixed with 100 μL of 10% SDS and 800 μL of 10 mM phosphate buffer (pH 8), and optical density was measured at 412 nm (A0). Then, 100 μL of DTNB was added and incubated at 37 °C for 60 min. After incubation, the absorbance of the sample was quantified at 412 nm (A1). The concentration of thiol groups was calculated from A1 to A0 subtraction using a molar extinction coefficient of 13.6 × 103 M−1 × cm−1. The results were expressed as the nmol of thiol groups per mg of protein.
2.13. H2O2 determination
The gastric mucosal H2O2 level was determined following Dingeon et al.28 Briefly, hydrogen peroxide reacts with p-hydroxybenzoic acid and 4-aminoantipyrine in the presence of peroxidase leading to the formation of quinoneimine that has a pink color detected at 505 nm.
2.14. Iron measurement
Gastric mucosa and plasma non haem iron were measured colorimetrically using ferrozine, as described by Leardi et al.29 Briefly, the iron dissociated from transferrin-iron complex by guanidine acetate solution was reduced by ascorbic acid and was reacted with ferrozine, leading to the formation of a pink complex measured at 562 nm.
2.15. Calcium determination
Gastric mucosa and plasma calcium were determined using a colorimetric method following the method reported by Stern and Lewis.30 Briefly, in alkaline medium, calcium reacted with cresolphtalein forming a colored complex detectable at 570 nm.
2.16. Protein determination
Protein concentration was determined according to Hartree31 as a slight modification of the Lowry method. Serum albumin was used as a standard.
2.17. Statistical analysis
The data were analyzed by unpaired Student's t-test and were expressed as means ± standard error of the mean (S.E.M.). The data are representative of 10 independent experiments. All the statistical tests were two-tailed, and a p value of 0.05 or less was considered significant.
3. Results
3.1. HPLC-PDA-ESI-MS/MS analysis
The maximum absorption wavelengths (λmax), parent ion, and fragment ion masses of the components detected in the aqueous extract of A. campestris are shown in Table 1, where the compounds are numbered according to their retention time (tR) in the obtained chromatograms (Fig. 1).
Table 1 Characterisation of phenolic compounds of Artemisia campestris aqueous extract by HPLC-DAD-ESI-MS/MS
Peak no. |
tR (min) |
UV λmax (nm) |
[M − H]− (m/z) |
Fragment ion (m/z) |
Composition (%) |
Tentative identification |
1 |
16.5 |
242, 298 (sh), 325 |
353 |
191; 179 |
4.3 |
Chlorogenic acid |
2 |
17.2 |
242, 296 (sh), 323 |
179 |
|
2.15 |
Caffeic acid |
3 |
21.4 |
259, 357 |
477 |
315 |
5.37 |
Isorhamnetin hexoside |
4 |
23.9 |
241, 298 (sh), 327 |
515 |
353, 191, 179 |
0.24 |
3,4-Dicaffeoylquinic acid |
5 |
24.1 |
241, 298 (sh), 329 |
515 |
353, 191, 179 |
0.78 |
3,5-Dicaffeoylquinic acid |
6 |
24.6 |
241, 298 (sh), 329 |
515 |
353, 191, 179 |
0.93 |
4,5-Dicaffeoylquinic acid |
7 |
25.1 |
265, 349 |
477 |
301 |
0.05 |
Quercetin-3-O-glucuronide |
8 |
25.7 |
267, 337 |
431 |
269 |
6.42 |
Apigenin-7-O-hexoside |
9 |
27.7 |
267, 339 |
577 |
517, 401, 269 |
15.52 |
Apigenin-6-C-glucuronide-8-C-pentoside |
10 |
30.03 |
253, 270 (sh), 347 |
285 |
|
2.74 |
Kaempferol |
11 |
32.88 |
267, 337 |
269 |
|
62.42 |
Apigenin |
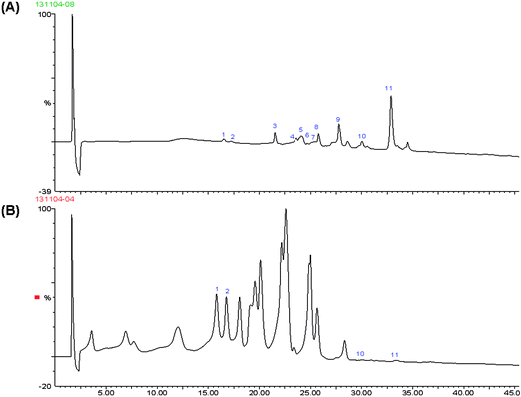 |
| Fig. 1 HPLC-DAD chromatograms monitored at 280 nm of (A) Artemisia campestris aqueous extract (ACAE) and (B) standard mixture. | |
Overall, 11 phenolic compounds including 5 hydroxycinnamic acid derivatives, 2 glycosylated flavonols, 2 glycosylated flavones, 1 flavonol aglycone and 1 flavone aglycone were identified.
The identification of hydroxycinnamic acids was based on UV-DAD spectra conjugated with the MS fragmentation data, and their retention time was compared with those of the authentic standards, when available. Consequently, peak 1 (tR = 16.5 min) was identified as chlorogenic acid (3-O-caffeoylquinic acid). It showed UV absorption maxima at 325 nm with a shoulder at 290–300 nm and exhibited deprotonated molecular ion at m/z 353 with the main MS2 fragment at m/z 191 and 179, indicating that the caffeoyl group is linked to the 3-OH position of quinic acid.19 The identity of this compound was confirmed by comparing its UV and MS2 spectra and HPLC retention time with the standard chlorogenic acid. Peak 2 (tR = 17.2 min; λmax 323 nm; [M − H]− at m/z 179) was identified as caffeic acid by comparing its UV and mass spectra and its tR with those of a reference standard.
Peaks 4 (tR = 23.9 min; λmax 327 nm), 5 (tR = 24.1 min; λmax 329 nm) and 6 (tR = 24.6; λmax 329) displayed the same deprotonated molecular ion at m/z 515 and the [M − H]− ion at m/z 353, indicating the loss of a caffeoyl residue. Based on the hierarchical key proposed by Clifford et al.19 and their order of elution, they were tentatively identified as 3,4-dicaffeoylquinic acid (compound 4), 3,5-dicaffeoylquinic acid (compound 5) and 4,5-dicaffeoylquinic acid (compound 6).
Peak 3 (tR = 21.4 min; λmax 259–357 nm) showed characteristic UV absorption of flavonols and exhibited [M − H]− ion at m/z 477. Its MS2 spectra gave [M − H-162]− ion at m/z at 315, indicative of the aglycone isorhamnetin. This compound was tentatively identified as isorhamnetin hexoside.20
Peak 7 (tR = 25.1 min; λmax 260–359 nm) gave a [M − H]− ion at m/z 477 and its MS2 fragmentation yielded a fragment ion at m/z 301 (quercetin aglycone) due to the loss of a glucuronic acid moiety (176 da). This compound was tentatively identified as quercetin-3-O-glucuronide.
Peaks 8 (tR = 25.7 min) and 9 (tR = 27.7 min) showed similar UV spectra with maximum absorbance at 267 and 337 nm, respectively, which represented characteristic UV absorption of flavone. Compound 8 displayed a deprotonated molecular ion at m/z 431 and gave a fragment ion at m/z 269 (apigenin aglycone), suggesting the loss of hexose moiety (162 da). As it is known, the most common substitution position for flavones is the 7-OH. Therefore, compound 8 was tentatively identified as apigenin-7-O-hexoside.21 Compound 9 exhibited [M − H]− ion at m/z 577 and its MS2 spectrum gave a fragment ion at m/z 517[M − H-60]−, at m/z 401 (loss of glucuronic acid moiety [M − H-176]−) and a fragment ion at m/z 269 (loss of pentose moiety [M − H-glucuronic acid-132]−) assigned as the aglycone apigenine. Because the fragment ion [M − H-60]− had a higher intensity for C-6 glycoside than for C-8 glycoside and indicated the fragmentation of a C-pentose unit,19 it is plausible to identify compound 9 as apigenin-6-C-glucuronide-8-C-pentoside.
Peak 10 (tR = 30.03 min), which displayed a [M − H]− ion at m/z 285 and had maximum UV absorbance at 253 and 347 nm, was identified as kaempferol by comparing its UV and MS spectra and HPLC retention time with those of a reference standard.
By using the same procedure, compound 11 (tR = 30.03 min; λmax 267–337 nm) with [M − H]− ion at m/z 269 was unequivocally identified as apigenin.
3.2. Antioxidant capacity of ACAE
Concerning the antioxidant capacity, we found that the radical-scavenging activity of ACAE and ascorbic acid against DPPH radical increased significantly in a dose-dependant manner (Fig. 2). The EC50 values calculated from the graph demonstrated a higher rate of RSA for ACAE (EC50 = 160.64 μg mL−1) but lesser than that of ascorbic acid (EC50 = 72.59 μg mL−1), a well-known antioxidant reference molecule.
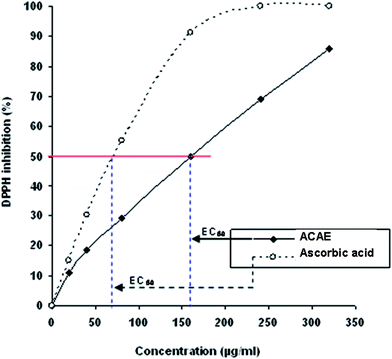 |
| Fig. 2 Free radical-scavenging activity of the Artemisia campestris aqueous extract (ACAE) and ascorbic acid on 2,2-diphenyl-1-picrylhydrazyl (DPPH). | |
3.3. Acute oral toxicity of CDE
In the acute oral toxicity study, neither abnormal behavior nor mortality was detected during the observation period. Thus, the LD50 value was greater than 3200 mg kg−1 b.w. for A. campestris aqueous extract.
3.4. Effect of ACAE on aspirin-induced acute macroscopic gastric injury
The macroscopic examination of gastric mucosa showed that aspirin administration exhibited injuries, including hemorrhage and hyperemia. ACAE, famotidine and caffeic acid treatment showed a dose dependant decrease in all the macrospical toxic signs compared with the aspirin treated group (Fig. 3). Moreover, quantitative analysis showed that pre-treatment with Artemisia campestris or reference molecules significantly and dose-dependently reduced the ulcer index and ameliorated the protection of injury induced by aspirin administration (Table 2).
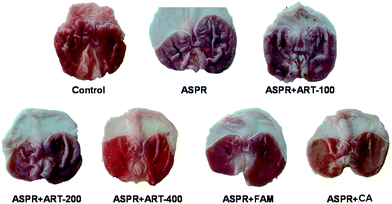 |
| Fig. 3 Subacute effect of Artemisia campestris aqueous extract (ACAE), famotidine and caffeic acid on macroscopic changes induced by aspirin in rats. Animals were pre-treated with various doses of ACAE (100, 200 and 400 mg kg−1, b.w., p.o.), famotidine (20 mg kg−1, b.w., p.o.), caffeic acid (50 mg kg−1, b.w., p.o.) or bi-distilled water, challenged with a single oral administration of aspirin (300 mg kg−1, b.w., p.o.) or NaCl (9%) for one hour. | |
Table 2 Subacute effect of Artemisia campestris aqueous extract (ACAE), famotidine and caffeic acid on macroscopic changes induced by aspirin in rats: ulcer index and inhibition percentage. Animals were pre-treated with various doses of ACAE (100, 200 and 400 mg kg−1, b.w., p.o.), famotidine (20 mg kg−1, b.w., p.o.), caffeic acid (50 mg kg−1, b.w., p.o.) or bi-distilled water, and they were challenged with a single oral administration of aspirin (300 mg kg−1, b.w., p.o.) or NaCl (9%) for one houra
Group |
Rats number |
Ulcer index (mm2) |
Protection percentage (%) |
*: p < 0.05 compared to control group and #: p < 0.05 compared to aspirin group. |
Control |
10 |
— |
— |
Aspirin |
10 |
62.75 ± 2.28* |
00 |
Aspirin + ART-100 |
10 |
42.25 ± 2.75# |
32.66 |
Aspirin + ART-200 |
10 |
31.52 ± 2.41# |
49.76 |
Aspirin + ART-400 |
10 |
13.66 ± 4.23 # |
78.23 |
Aspirin + FAM |
10 |
15.5 ± 1.32# |
75.29 |
Aspirin + CA |
10 |
09.25 ± 1.54# |
85.25 |
3.5. Effect of ACAE on aspirin-induced acute histopathological gastric injury
Histological observation demonstrated that gastric mucosa of rats pre-treated with H2O and intoxicated with aspirin at 300 mg kg−1 showed marked erosive lesions in the gastric tissue. ACAE, famotidine and caffeic acid pre-treatment significantly reduced the structural changes induced by aspirin intoxication (Fig. 4).
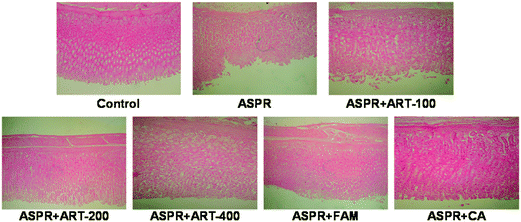 |
| Fig. 4 Subacute effect of Artemisia campestris aqueous extract (ACAE), famotidine and caffeic acid on histological changes induced by aspirin in rats. Animals were pre-treated with various doses of ACAE (100, 200 and 400 mg kg−1, b.w., p.o.), famotidine (20 mg kg−1, b.w., p.o.), caffeic acid (50 mg kg−1, b.w., p.o.) or bi-distilled water, challenged with a single oral administration of aspirin (300 mg kg−1, b.w., p.o.) or NaCl (9%) for one hour. | |
3.6. Effects of ACAE on aspirin-induced gastric mucosa lipoperoxidation
To investigate the implication of oxidative stress in the gastroprotective effect of Artemisia campestris, stomach mucosa was first assessed for MDA determination. We showed that aspirin administration significantly increased stomach mucosal MDA level. More importantly, ACAE pre-treatment significantly and dose-dependently reduced gatric lipoperoxidation induced by NSAIDs intoxication (Fig. 5). Lipoperoxidation was also corrected by famotidine and caffeic acid administration.
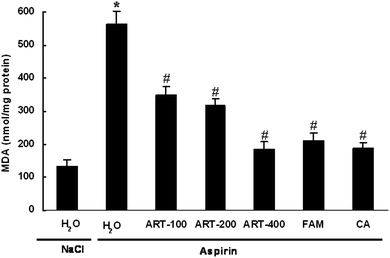 |
| Fig. 5 Subacute effect of Artemisia campestris aqueous extract (ACAE), famotidine and caffeic acid on aspirin-induced changes in stomach mucosal MDA level in rats. Animals were pre-treated with various doses of ACAE (100, 200 and 400 mg kg−1, b.w., p.o.), famotidine (20 mg kg−1, b.w., p.o.), caffeic acid (50 mg kg−1, b.w., p.o.) or bi-distilled water, challenged with a single oral administration of aspirin (300 mg kg−1, b.w., p.o.) or NaCl (9%) for one hour. Assays were carried out in triplicate. *: p < 0.05 compared to control group and #: p < 0.05 compared to aspirin group. | |
3.7. Effects of ACAE on depletion of aspirin-induced mucosa antioxidant enzyme activities
We also studied the gastric antioxidant enzyme activities (Fig. 6). As expected, aspirin treatment significantly decreased stomach mucosa antioxidant enzyme activities as SOD (A), CAT (B) and GPx (C). Pre-treatment with ACAE, famotidine or caffeic acid significantly reversed all the aspirin-induced reductions in antioxidant enzyme activities to near control in a dose-dependent manner.
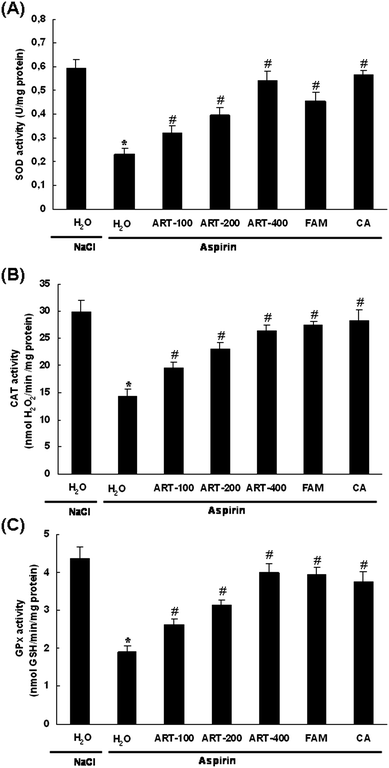 |
| Fig. 6 Subacute effect of Artemisia campestris aqueous extract (ACAE), famotidine and caffeic acid on aspirin-induced changes in stomach mucosa antioxidant enzyme activities: SOD (A), CAT (B) and GPx (C) in rats. Animals were pre-treated with various doses of ACAE (100, 200 and 400 mg kg−1, b.w., p.o.), famotidine (20 mg kg−1, b.w., p.o.), caffeic acid (50 mg kg−1, b.w., p.o.) or bi-distilled water, challenged with a single oral administration of aspirin (300 mg kg−1, b.w., p.o.) or NaCl (9%) for one hour. Assays were carried out in triplicate. *: p < 0.05 compared to control group and #: p < 0.05 compared to aspirin group. | |
3.8. Effects of ACAE on aspirin-induced changes in gastric mucosa –SH group's content
Moreover, we examined the effect of ACAE and aspirin treatment on sulfhydril levels. As expected, gastric mucosa injuries were accompanied by a significant decrease in –SH groups content. Subacute Artemisia campestris pre-treatment significantly and dose-dependently corrected the decrease in thiol groups induced by aspirin administration. This effect was also significantly corrected by subacute famotidine and caffeic acid pre-treatment (Fig. 7).
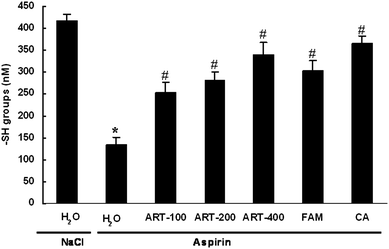 |
| Fig. 7 Subacute effect of Artemisia campestris aqueous extract (ACAE), famotidine and caffeic acid on aspirin-induced changes in stomach mucosa SH– groups level in rats. Animals were pre-treated with various doses of ACAE (100, 200 and 400 mg kg−1, b.w., p.o.), famotidine (20 mg kg−1, b.w., p.o.), caffeic acid (50 mg kg−1, b.w., p.o.) or bi-distilled water, challenged with a single oral administration of aspirin (300 mg kg−1, b.w., p.o.) or NaCl (9%) for one hour. Assays were carried out in triplicate. *: p < 0.05 compared to control group and #: p < 0.05 compared to aspirin group. | |
3.9. Effects of ACAE on aspirin-induced gastric mucosa elevation of iron, H2O2 and calcium
We further examined the effect of aspirin and ACAE on intracellular mediators as hydrogen peroxide, free iron and calcium levels in gastric mucosa (Table 3). Aspirin per se significantly increased free iron, H2O2 and calcium levels. However, sub-acute pre-treatment with ACAE, famotidine or caffeic acid significantly reduced the deregulation of aspirin-induced intracellular mediators in a dose-dependent manner. ACAE alone had no considerable effects on these parameters.
Table 3 Subacute effect of Artemisia campestris aqueous extract (ACAE), famotidine and caffeic acid on aspirin-induced changes in stomach mucosa and plasma hydrogen peroxide, free iron and calcium levels in rats. Animals were pre-treated with various doses of ACAE (100, 200 and 400 mg kg−1, b.w., p.o.), famotidine (20 mg kg−1, b.w., p.o.), caffeic acid (50 mg kg−1, b.w., p.o.) or bi-distilled water, and were challenged with a single oral administration of aspirin (300 mg kg−1, b.w., p.o.) or NaCl (9%) for one hour. Assays were carried out in triplicatea
Group |
Hydrogen peroxide |
Free iron |
Calcium |
Gastric mucosa (nmol per mg per protein) |
Plasma (nmol mL−1) |
Gastric mucosa (μmol per mg per protein) |
Plasma (nmol mL−1) |
Gastric mucosa (μmol per mg per protein) |
Plasma (μmol mL−1) |
*: p < 0.05 compared to control group and #: p < 0.05 compared to aspirin group. |
Control |
95.6 ± 6.72 |
956 ± 35.58 |
22.75 ± 1.84 |
0.97 ± 0.04 |
115.52 ± 7.26 |
1.68 ± 0.1 |
Aspirin |
309.5 ± 14.59* |
1800 ± 113.73* |
60.00 ± 4.40* |
1.23 ± 0.04* |
285.20 ± 9.64* |
2.78 ± 0.08* |
Aspirin + ART-100 |
159.2 ± 18.57# |
1451.67 ± 16.18# |
46.67 ± 2.94# |
1.10 ± 0.04# |
183.11 ± 11.85# |
2.19 ± 0.1# |
Aspirin + ART-200 |
136.8 ± 10.66# |
1210 ± 91.76# |
33.75 ± 2.72# |
1.05 ± 0.03# |
141.47 ± 8.14# |
1.93 ± 0.09# |
Aspirin + ART-400 |
109.8 ± 11.13# |
967.5 ± 39.85# |
20.00 ± 2.39# |
1.03 ± 0.02# |
119.74 ± 11.70# |
1.77 ± 0.05# |
Aspirin + FAM |
108.5 ± 16.07# |
1117.5 ± 71.96# |
43.50 ± 4.11# |
0.96 ± 0.04# |
165.91 ± 10.70# |
1.92 ± 0.1# |
Aspirin + CA |
135.6 ± 11.02# |
1003 ± 53.35# |
30.75 ± 4.14# |
0.96 ± 0.04# |
213.20 ± 12.72# |
2.09 ± 0.09# |
4. Discussion
The present study was undertaken to investigate the protective effect of Artemisia campestris aqueous extract on aspirin-induced gastric ulceration. We also investigated the implication of oxidative stress as well as some gastric mucosal defence mechanisms in this eventual gastroprotection.
A phytochemical study of the ACAE using HPLC-PDA-ESI-MS/MS analysis allowed the identification of 11 phenolic compounds: chlorogenic acid, caffeic acid, isorhamnetin hexoside, 3,4-dicaffeoylquinic acid, 3,5-dicaffeoylquinic acid, 4,5-dicaffeoylquinic acid, quercetin-3-O-glucuronide, apigenin-7-O-hexoside, apigenin-6-C-glucuronide-8-C-pentoside, kaempferol and apigenin. These molecules were previously reported in many plant extracts and are known for their antioxidant and gastroprotective properties.32 In this respect, the DPPH radical-scavenging assay was investigated. We showed that ACAE presents a higher scavenging capacity but lesser than that of ascorbic acid used as reference molecule. The antioxidant capacity of ACAE is mainly related to the higher levels of polyphenols and flavonoids (data not shown). However, a positive correlation between phenolic compounds and antioxidant capacity is common in the majority of natural extracts.33,34
In vivo, we first showed that acute aspirin administration clearly induced macroscopic injuries, including hemorrhage and hyperemia, as well as histopatological changes such as erosive lesions. Synthetic non-steroidal anti-inflammatory drugs (NSAIDs), such as aspirin, causes mucosal damage by interfering with prostaglandin synthesis, increasing acid secretion and blocking H+ diffusion.35 Nevertheless, the key mechanism of NSAIDs-induced gastric ulceration is the irreversible and non-selective inhibition of cyclooxygenase activity, which interferes with the synthesis of prostaglandins. This triggers the effects derived from PG depletion, and shuttles the arachidonic acid metabolism toward the lipoxygenase pathway, increasing the formation of vasoconstrictor leukotrienes.36,37
Our data also showed that sub-acute pre-treatment with ACAE, famotidine or caffeic acid abolished all macroscopic and microscopic changes induced by aspirin intoxication in a dose-related manner. Concerning ACAE acute toxicity, we showed that the LD50 value was greater than 3200 mg kg−1 b.w. and neither mortality nor behavior impairment were observed during the observation period. However, aspirin-induced gastric ulceration has been previously shown to be attenuated by many plants extracts such as Chamaemelum nobile,38 Rubia cordifolia,39 Allium sativum,40 Centaurium erythraea,41 Cucurbita pepo42 as well as grape seed extract.9
Importantly, in the present study, we showed that acute aspirin administration induced an oxidative stress status in the gastric mucosa assessed by an increase in lipoperoxidation level as well as a depletion of antioxidant enzyme activities such as SOD, CAT and GPx. These data fully corroborated previous reports, indicating that NSAIDs-induced gastric mucosal injuries, including aspirin, are accompanied by an oxidative stress status.43–45
Aspirin, when used at the therapeutic level, is safe and broadly utilized as NSAIDs but higher doses or prolonged use of this drug may induce gastrointestinal erosions and apoptotic lesions.46 NSAIDs also exert other biological effects, including the generation of ROS and the inhibition of NF-κB-mediated signals.47 Excessive ROS production causes lipid membranes and protein attack as well as ultimately disrupting cellular integrity, leading to incontrollable oxidative stress status.48 Importantly, in the present work, we showed that Artemisia campestris pre-treatment protects against aspirin-induced oxidative stress in gastric mucosa. A. campestris has recently been proven to be rich in bioactive compounds such as polyophenols.13,15 These molecules are the primal source of the antioxidant ability of this plant by scavenging free radicals, such as hydroxyl radical (OH˙), which is the major cause of lipid peroxidation.49 Because of their antioxidant ability, A. campestris extrats were shown to have many beneficial health effects in vivo14–16 and in vitro.15 However, kaempferol, apigenin or its derivatives identified in the ACAE were previously presented as having both antiulcer and antioxidant properties.50,51 To the best of our knowledge, our report is the first to deal with the protective effect of A. campestris aqueous extract on acute aspirin-induced injuries in the rat gastric mucosa.
In this respect, ACAE has also been shown to protect against the decrease of thiol groups levels induced by aspirin treatment. It is well-known that sulfhydryls are in part involved in gastric cytoprotection.52,53 However, sulfhydryls are known to maintain the integrity of mucosal barrier and scavenge free radicals formed due to the action of noxious agents.54 The capacity of aspirin to decrease the level of sulfhydryl groups was previously shown in gastric mucosa55 and liver.56 The problem of NSAIDs, such as aspirin, effect on sulfur metabolism has already been an interesting domain of investigation. In this respect, it has been demonstrated that aspirin is effective in promoting increased sulphate incorporation into the bone-forming regions of the chick.57 However, it is tempting to speculate that aspirin and ACAE exert opposite effects on sulfhydryl groups in gastric mucosa. The same mechanism was previously described but for an EtOH-induced SH-group level decrease using silymarin as a gastoprotective mediator.55
More interestingly, in the present work, we showed that A. campestris pre-treatment abolished acute aspirin-induced increases in H2O2, free iron and calcium levels in plasma and gastric mucosa. However, iron and H2O2 accumulation catalyzed the highly toxic hydroxyl radical (OH˙) production via the Fenton reaction, leading to membrane lipoperoxidation and enhancement of its permeability to calcium.58
It is generally reported that oxidants can cause a rapid increase in cytoplasm calcium levels in various cell types.59,60 This increase may be due to both the release of calcium from internal cellular organelles, such as the endoplasmic or sarcoplasmic reticulum, or its import from extracellular space across the plasma membrane. This function is dependent on calcium concentration and the involved oxidants.61 Indeed, high concentrations of hydrogen peroxide were previously shown to induce a cytosolic calcium increase accompanied by a reduction in extracellular levels.62 Thus, we can speculate that aspirin and ACAE exerted opposite effects on free iron and hydrogen peroxide leading to calcium homeostasis.
5. Conclusion
The results of the present study clearly indicate that A. campestris aqueous extract protected against aspirin-induced lesions in gastric mucosa. Our results also demonstrated that AEAC gastroprotection might be related, at least in part, to its antioxidant properties as well as to various gastric mucosal defence mechanisms, including the protection of gastric sulfhydryls and an opposite effect on some intracellular mediators such as free iron, hydrogen peroxide and calcium.
Declaration of interest
The authors alone are responsible for the contents of this paper.
Abbreviations
ACAE | Artemisia campestris aqueous extract |
ART | Artemisia campestris |
CA | Caffeic acid |
CAT | Catalase |
FAM | Famotidine |
GPx | Glutathione peroxidase |
H2O2 | Hydrogen peroxide |
MDA | Malondialdehyde |
NSAIDs | Non-steroidal anti-inflammatory drugs |
SOD | Superoxide dismutase |
SH | Sulfhydryl |
Acknowledgements
Financial support from the Tunisian Ministry of Higher Education and Scientific Research is gratefully acknowledged. Financial disclosures: none declared.
References
- G. B. Glavin and S. Szabo, Experimental gastric mucosal injury: laboratory models reveal mechanisms of pathogenesis and new therapeutic strategies, FASEB J., 1992, 6, 825–831 CAS.
- C. Tasman-Jones, Pathogenesis of peptic ulcer disease and gastritis: importance of aggressive and cytoprotective factors, Scand. J. Gastroenterol., 1986, 22, 1–5 CrossRef.
- K. Ramakrishnan and R. C. Salinas, Peptic ulcer disease, Am. Fam. Physician, 2007, 76, 1005–1012 Search PubMed.
- L. Laine, K. Takeuchi and A. Tarnawski, Gastric mucosal defense and cytoprotection: bench to bedside, Gastroenterology, 2008, 135, 41–60 CrossRef CAS PubMed.
- A. R. Dehpour, M. E. Zolfaghari, T. Samadian and Y. Vahedi, The protective effect of liquorice components and their derivatives against gastric ulcer induced by aspirin in rats, J. Pharm. Pharmacol., 1994, 46, 148–149 CrossRef CAS PubMed.
- I. Wiklund, Quality of life in arthritis patients using nonsteroidal anti-inflammatory drugs, Can. J. Gastroenterol., 1999, 13, 129–133 CAS.
- A. Saggioro, V. Alvisi, A. Blasi, G. Dobrilla, A. Fioravant and R. Marcolongo, Misoprostol prevents NSAID-induced gastroduodenal lesions in patients with osteoarthritis and rheumatoid arthritis, Ital. J. Gastroenterol., 1991, 23, 119–123 CAS.
- A. R. Dehpour, M. E. Zolfaghari, T. Samadian, F. Kobarfard, M. Faizi and M. Assari, Antiulcer activities of liquorice and its derivatives in experimental gastric lesion induced by ibuprofen in rats, Int. J. Pharm., 1995, 119, 133–138 CrossRef CAS.
- V. M. Cuevas, Y. R. Calzado, Y. P. Guerra, A. O. Yera, S. J. Despaigne, R. M. Ferreiro and D. C. Quintana, Effects of grape seed extract, vitamin C, and vitamin E on ethanol- and aspirin-induced ulcers, Adv. Pharmacol. Sci., 2011, 2011, 740 Search PubMed.
- T. Pohle, T. Brzozowski and J. C. Becker, Role of reactive oxygen metabolites in aspirin-induced gastric damage in humans: gastroprotection by vitamin C, Aliment. Pharmacol. Ther., 2001, 15, 677–687 CrossRef CAS.
- M. Ikeda, K. Nakabayashi, M. Shinkai, Y. Hara, T. Kizaki, S. Oh-ishi and H. Ohno, Supplementation of antioxidants prevents oxidative stress during a deep saturation dive, Tohoku J. Exp. Med., 2004, 203, 353–357 CrossRef CAS PubMed.
- C. A. Hiruma-Lima, J. S. Gracioso, W. Toma, A. B. Almeida, A. C. Paula and D. S. Brasil, Gastroprotective effect of aparisthman, a diterpene isolated from Aparisthmium cordatum on experimental gastric ulcer models in rats and mice, Phytomedicine., 2001, 8, 94–100 CrossRef CAS PubMed.
- A. Akrout, L. A. Gonzalez, H. El Jani and P. C. Madrid, Antioxidant and antitumor activities of Artemisia campestris and Thymelaea hirsuta from southern Tunisia, Food Chem. Toxicol., 2010, 49, 342–347 CrossRef PubMed.
- M. Sefi, A. Troudi, F. B. Hamida, N. Soudani, T. Boudawara and N. Zeghal, Protective effects of Artemisia campestris upon fenthion-induced nephrotoxicity in adult rats and their progeny, Gen. Physiol. Biophys., 2013, 32, 577–588 CrossRef PubMed.
- Y. Aniya, M. Shimabukuro, M. Shimoji, M. Kohatsu, M. A. Gyamfi, C. Miyagi, D. Kunii, F. Takayama and T. Egashira, Antioxidant and hepatoprotective actions of the medicinal herb Artemisia campestris from the Okinawa Islands, Biol. Pharm. Bull., 2000, 23, 309–312 CAS.
- M. Sefi, H. Fetoui, M. Makni and N. Zeghal, Mitigating effects of antioxidant properties of Artemisia campestris leaf extract on hyperlipidemia, advanced glycation end products and oxidative stress in alloxan-induced diabetic rats, Food Chem. Toxicol., 2010, 48, 1986–1993 CrossRef CAS.
- A. Houicher, E. Kuley, B. Bendeddouche and F. Ozogul, Effect of Mentha spicata L. and Artemisia campestris extracts on the shelf life and quality of vacuum-packed refrigerated sardine (Sardina pilchardus) fillets, J. Food Prot., 2013, 76, 1719–1725 CrossRef CAS PubMed.
- T. Carbonara, R. Pascale, M. P. Argentieri, P. Papadia, F. P. Fanizzi, L. Villanova and P. Avato, Phytochemical analysis of a herbal tea from Artemisia annua L, J. Pharm. Biomed. Anal., 2012, 62, 79–86 CrossRef CAS PubMed.
- M. N. Clifford, S. Knight and N. Kuhnert, Discriminating between the six isomers of dicaffeoylquinic acid by LC–MSn, J. Agric. Food Chem., 2005, 53, 3821–3832 CrossRef CAS PubMed.
- J. Han, M. Ye, X. Qiao, M. Xu, B. R. Wong and D. A. Guo, Characterisation of phenolic compounds in the Chinese herbal drug Artemisia annua by liquid chromatography coupled to electrospray ionisation mass spectrometry, J. Pharm. Biomed. Anal., 2008, 47, 516–525 CrossRef CAS PubMed.
- S. Gouveia and P. C. Castilho, Characterisation of phenolic acid derivatives and flavonoids from different morphological parts of Helichrysum obconicum by a RP-HPLC–DAD-(-)–ESI-MSn method, Food Chem., 2011, 129, 333–344 CrossRef CAS PubMed.
- I. Grzegorczyk, A. Matkowski and H. Wysokinska, Antioxidant activity of extracts from in vitro cultures of Salvia officinalis L, Food Chem., 2007, 104, 536–541 CrossRef CAS PubMed.
- H. H. Draper and M. Hadley, Malondialdehyde determination as index of lipid peroxidation, Methods Enzymol., 1990, 186, 421–431 CAS.
- H. P. Misra and I. Fridovich, The role of superoxide anion in autoxidation of epinephrine and a simple assay for superoxide dismutase, J. Biol. Chem., 1972, 247, 3170–3175 CAS.
- H. Aebi, Catalase in vitro, Methods Enzymol., 1984, 105, 121–126 CAS.
- L. Flohé and W. A. Günzler, Assays of glutathione peroxidase, Methods Enzymol., 1984, 105, 114–121 Search PubMed.
- G. L. Ellman, Tissue sulfhydryl groups, Arch. Biochem. Biophys., 1959, 82, 70–77 CrossRef CAS.
- B. Dingeon, J. P. Ferry and A. Roullet, Automatic assay of blood sugar by Trinder's method, Ann. Biol. Clin., 1975, 33, 3–13 CAS.
- A. Leardi, M. Caraglia, C. Selleri, S. Pepe, C. Pizzi, R. Notaro, A. Fabbrocini, D. S. Lorenzo, M. Musicò, A. Abbruzzese, A. R. Bianco and P. Tagliaferri, Desferioxamine increases iron depletion and apoptosis induced by ara-C of human myeloid leukaemic cells, Br. J. Haematol., 1998, 102, 746–752 CrossRef CAS.
- J. Stern and W. H. Lewis, The colorimetric estimation of calcium in serum with ocresolphthalein complexone, Clin. Chim. Acta, 1957, 2, 576–580 CrossRef CAS.
- E. F. Hartree, Determination of protein: a modification of the Lowry method that gives a linear photometric response, Anal. Biochem., 1972, 48, 422–427 CrossRef CAS.
- P. A. Rodrigues, S. M. Morais, C. M. Souza, D. V. Magalhães, I. G. Vieira, G. M. Andrade, V. S. Rao and F. A. Santos, Gastroprotective effect of Byrsonima sericea DC leaf extract against ethanol-induced gastric injury and its possible mechanisms of action, An. Acad. Bras. Cienc., 2012, 84, 113–122 CrossRef PubMed.
- S. U. Chon, B. U. Heo, Y. S. Park, D. K. Kim and S. Gorinstein, Total phenolics level, antioxidant activities and cytotoxicity of young sprouts of some traditional Korean salad plants, Plant Foods Hum. Nutr., 2009, 64, 25–31 CrossRef CAS PubMed.
- İ. Hamad, Ö. Erol-Dayi, M. Pekmez, E. Önay-Uçar and N. Arda, Antioxidant and cytotoxic activities of Aphanes arvensis extracts, Plant Foods Hum. Nutr., 2010, 65, 44–49 CrossRef PubMed.
- C. V. Roa, R. N. Maiti and R. K. Goel, Effect of mid irritant on gastric mucosal offensive and defensive factors. Med, J. Physiol. Pharmacol., 1999, 44, 185–191 Search PubMed.
- B. J. Whittle, Gastrointestinal effects of nonsteroidal anti-inflammatory drugs, Fundam. Clin. Pharmacol., 2003, 17(3), 301–313 CrossRef CAS.
- M. Jainu and C. S. Devi, Gastroprotective action of Cissus quadrangularis extract against NSAID induced gastric ulcer: role of proinflammatory cytokines and oxidative damage, Chem.–Biol. Interact., 2006, 161, 262–270 CrossRef CAS PubMed.
- H. Nomiri, S. J. Aldavood and R. Sedaghat, Comparative efficacy of chamomile against omeprazole in aspirin-induced gastric ulcer in rats, Comp. Clin. Pathol., 2014, 23, 673–678 CrossRef CAS PubMed.
- R. S. Deoda, D. Kumar and S. S. Bhujbal, Gastroprotective Effect of Rubia cordifolia Linn. on Aspirin Plus Pylorus-Ligated Ulcer, J. Evidence-Based Complementary Altern. Med., 2011, 2011, 541–624 Search PubMed.
- M. A. Mabrouk, F. I. Nnawodu, Y. Tanko, F. Dawud and A. Mohammed, Effect of Aqueous Garlic (Ag) Extract on Aspirin Induced Gastric Mucosal Lesion in Albino Wistar Rats, J. Biol. Sci., 2009, 1, 15–19 Search PubMed.
- Y. Tuluce, H. Ozkol, I. Koyuncu and H. Ine, Gastroprotective effect of small centaury (Centaurium erythraea L) on aspirin-induced gastric damage in rats, Toxicol. Ind. Health, 2011, 27, 760–768 CrossRef PubMed.
- S. Sarkar and D. Buha, Effect of ripe fruit pulp extract of Cucurbita pepo Linn. in aspirin induced gastric and duodenal ulcer in rats, Indian J. Exp. Biol., 2008, 46, 639–645 CAS.
- M. Jainu, V. Mohan and S. Devi, Protective effect of Cissus quadrangularis on neutrophil mediated tissue injury induced by aspirin in rats, J. Ethnopharmacol., 2006, 104, 302–305 CrossRef PubMed.
- L. Liu, J. Cui, C. J. Song, J. S. Bian, A. Sparatore, P. D. Soldato, X. Y. Wang and C. D. Yan, H(2)S-releasing aspirin protects against aspirin-induced gastric injury via reducing oxidative stress, PLoS One, 2012, 7, 46301 Search PubMed.
- J. Y. Zhang, Q. F. Wu, Y. Wan, S. D. Song, J. Xu, X. S. Xu, H. L. Chang, M. H. Tai, Y. F. Dong and C. Liu, Protective role of hydrogen-rich water on aspirin-induced gastric mucosal damage in rats, World J. Gastroenterol., 2014, 20, 1614–1622 CrossRef CAS PubMed.
- N. R. Jana, NSAIDs and apoptosis, Cell. Mol. Life Sci., 2008, 65, 1295–1301 CrossRef CAS PubMed.
- M. Adachi, H. Sakamoto, R. Kawamura, W. Wang, K. Imai and Y. Shinomura, Nonsteroidal anti-inflammatory drugs and oxidative stress in cancer cells, Histol. Histopathol., 2007, 22, 437–442 CAS.
- L. Gaté, J. Paul, G. N. Ba, K. D. Tew and H. Tapiero, Oxidative stress induced in pathologies: the role of antioxidants, Biomed. Pharmacother., 1999, 53, 169–180 CrossRef.
- D. A. Kogiannou, N. Kalogeropoulos, P. Kefalas, M. G. Polissiou and A. C. Kaliora, Herbal infusions; their phenolic profile, antioxidant and anti-inflammatory effects in HT29 and PC3 cells, Food Chem. Toxicol., 2013, 61, 152–159 CrossRef CAS PubMed.
- R. K. Goel, V. B. Pandey, S. P. Dwivedi and Y. V. Rao, Antiinflammatory and antiulcer effects of kaempferol, a flavone, isolated from Rhamnus procumbens, Indian J. Exp. Biol., 1988, 26, 121–124 CAS.
- A. S. Awaad, N. A. Al-Jaber, J. E. Moses, R. M. El-Meligy and M. E. Zain, Antiulcerogenic activities of the extracts and isolated flavonoids of Euphorbia cuneata Vahl, Phytother. Res., 2013, 27, 126–130 CrossRef CAS PubMed.
- O. Strubelt and R. Hoppenkamps, Relations between gastric glutathione and the ulcerogenic action of non-steroidal anti-inflammatory drugs, Arch. Int. Pharmacodyn. Ther., 1983, 262, 268–278 CAS.
- S. Szabo, Role of sulfhydryls and early vascular lesions in gastric mucosal injury, Acta Physiol. Hung., 1984, 64, 203–214 CAS.
- S. Szabo and P. Vattay, Experimental gastric and duodenal ulcers. Advances in pathogenesis, Clin. Gastroenterol., 1990, 19, 67–85 CAS.
- J. H. Shin, C. W. Lee, S. J. Oh, J. Yun, K. Lee, S. K. Park, H. M. Kim, S. B. Han, Y. Kim, H. C. Kim and J. S. Kang, Protective effect of silymarin against ethanol-induced gastritis in rats: role of sulfhydryls, nitric oxide and gastric sensory afferents, Food Chem. Toxicol., 2013, 55, 353–357 CrossRef CAS PubMed.
- A. Bilska, M. Iciek, I. Kwiecień, K. Kaniecki, M. Paliborek, E. Somogyi, J. Piotrowska, B. Wiliński, M. Góralska, Z. Srebro and L. Włodek, Effects of aspirin on the levels of hydrogen sulfide and sulfane sulfur in mouse tissues, Pharmacol. Rep., 2010, 62, 304–310 CrossRef CAS.
- R. P. Dowdy and F. H. Nielsen, Effect of histidine, histamine, and aspirin on sulfur-35 metabolism in zinc-deficient chick bone, J. Nutr., 1972, 102, 529–534 CAS.
- L. W. Powell, The role of alcoholism in hepatic iron storage disease, Ann. N. Y. Acad. Sci., 1975, 252, 124–134 CrossRef CAS PubMed.
- T. A. Rooney, D. C. Renard, E. J. Sass and A. P. Thomas, Oscillatory cytosolic calcium waves independent of stimulated inositol 1,4,5-trisphosphate formation in hepatocytes, J. Biol. Chem., 1991, 266, 12272–12282 CAS.
- H. Wang and J. A. Joseph, Mechanisms of hydrogen peroxide-induced calcium dysregulation in PC12 cells, Free Radical Biol. Med., 2000, 28, 1222–1231 CrossRef CAS.
- K. Bielefeldt, C. A. Whiteis, R. V. Sharma, F. M. Abboud and J. L. Conklin, Reactive oxygen species and calcium homeostasis in cultured human intestinal smooth muscle cells, Am. J. Physiol., 1997, 272, 1439–1450 Search PubMed.
- A. Roveri, M. Coassin, M. Maiorino, A. Zamburlini, F. T. van Amsterdam, E. Ratti and F. Ursini, Effect of hydrogen peroxide on calcium homeostasis in smooth muscle cells, Arch. Biochem. Biophys., 1992, 297, 265–270 CrossRef CAS.
Footnote |
† The first two authors contributed equally to this work. |
|
This journal is © The Royal Society of Chemistry 2014 |