DOI:
10.1039/C4RA07419J
(Paper)
RSC Adv., 2014,
4, 35803-35807
Versatile and sustainable alcoholysis of amides by a reusable CeO2 catalyst†
Received
22nd July 2014
, Accepted 31st July 2014
First published on 4th August 2014
Abstract
CeO2 catalyzed the esterification between an equivalent molar ratio of primary amides and alcohols under neutral conditions, which provides the first versatile reusable catalytic system for direct alcoholysis of amides to esters with wider scope and 67 times higher turnover number (TON) than previous catalytic systems.
The amide bond is thermodynamically stable, and thus amide solvolysis usually requires heating under strongly acidic or basic conditions because of the difficulty of cleaving an amide bond. Therefore, the synthetic application of amide solvolysis is limited. Synthesis of ester by amide alcoholysis has attracted the attention of organic chemists, but all of the reported methods are not effective in terms of generality and atom economy.1–5 Although various non-catalytic methods of amide alcoholysis have been reported, they suffer from use of excess amount of promoter (such as HCl and NaNO2), generation of inorganic or organic wastes, and limited substrate scope.1–10 Twisted amides undergo alcoholysis under mild and neutral conditions,11–13 but synthetic application of this reaction is quite limited. Mashima and co-workers demonstrated the first example of catalytic amide alcoholysis using Zn(OTf)2,14 though the method is only applicable to the reaction of β-hydroxyethylamides with n-BuOH, and stoichiometric amount of promoter was required. Very recently, Mashima et al.15 developed combined catalytic system of Sc(OTf)3 and boronic ester for alcoholysis of primary amide to the corresponding ester. However, the method is only applicable to the ethanol and 1-propanol and has drawbacks such as inability of catalyst reuse, low TON, necessity of excess amount of alcohol, and difficulty in catalyst/product separation. To the best of our knowledge, there are no reports on reusable catalysts for the alcoholysis of amide to the corresponding ester. As a part of our continuing interest in CeO2-catalysed green organic syntheses,16–20 we report herein a general catalytic system for esterification between equivalent molar ratios of amide and alcohol under additive-free neutral conditions using CeO2 as reusable heterogeneous catalyst.
First, we performed catalyst screening adopting a model reaction of equimolar amount of n-valeramide and benzylalcohol in mesitylene under reflux conditions. Table 1 lists initial rate per gram of catalyst under the conditions in which conversions were below 25%. Among various metal oxides and solid acids tested (metal oxides: CeO2, CaO, MgO, ZnO, Y2O3, TiO2, ZrO2, Nb2O5, Al2O3, SiO2, solid acids: Amberlyst-15, HBEA, niobic acid, mont. K10, Nafion-SiO2), CeO2 showed 1–3 orders of magnitude higher rate of ester formation than the other metal oxides. Thermal reaction of n-valeramide and benzyl alcohol in the absence of any catalysts was found completely inactive for the ester formation.
Table 1 Ester formation from amide and alcohols by metal oxide catalysts

|
Catalyst |
Va/mmol h−1 g−1 |
Initial rate per gram of catalyst under the conditions in which conversions were below 25%. |
CeO2 |
41 |
CaO |
2.7 |
MgO |
0.22 |
ZnO |
0.07 |
Y2O3 |
0.33 |
TiO2 |
0.32 |
ZrO2 |
0.37 |
Nb2O5 |
0.29 |
Al2O3 |
0.10 |
SiO2 |
0.03 |
Amberlyst-15 |
2.17 |
HBEA |
1.06 |
Niobic acid |
1.39 |
Mont-K10 |
0.16 |
Nafion-SiO2 |
0.72 |
Blank |
0 |
Using the most effective catalyst, CeO2, we studied general applicability of the present catalytic system. Table 2 shows the yields of the corresponding esters from the reaction of various primary amides with equimolar amount of benzylalcohol. Various aliphatic linear amides with short to long carbon chain (entries 1–5) were tolerated, giving 100% conversion of amide and high isolated yield (86–93%) of corresponding esters. Benzylamide, benzamides with electron-donating and electron-withdrawing groups (entries 6–11) and a heteroaromatic amide (entry 12) were converted to the corresponding esters in good to excellent isolated yield (75–95%) at 100% conversions of alcohols and amides. α, α-Disubstituted amide (entry 13) and aliphatic diamide (entry 14) were also tolerated with good yield (86% and 75%). Gram-scale experiments with 10 mg of CeO2 (0.58 mol% Ce) for the reaction of valeramide and benzylalcohol (eqn (1)) and that of p-methoxybenzamide with 1-propanol (eqn (2)) gave 95% and 85% yields, respectively. Based on the number of Ce cations on the surface of CeO2 (1.067 mmol g−1),21 turnover number (TON) for the latter reaction is 888, which is 67 times higher than the TON (13.2) of homogeneous catalytic system with Sc(OTf)3 and boronic ester for the same reaction under excess amount of 1-propanol (6.7 equivalent).
|
 | (1) |
|
 | (2) |
Table 2 CeO2-catalyzed ester formation from various amide and benzylalcohol
Next we investigated the scope of alcohols. Table 3 shows the reaction of various alcohols with equimolar amount of benzamide. Linear and branched aliphatic alcohols (entries 1–12) and an allylalcohol were (entry 13) converted to the corresponding ester with 100% conversions and high isolated yields (80–90%). Benzyl alcohols with electron-donating or electron-withdrawing group (entries 14–19) reacted to afford the corresponding ester in good to high yields (75–89%). A heteroaromatic alcohol (entry 20) was also successfully converted to the ester with high yield (83%). The GC-MS analysis for all the reactions in Tables 2 and 3 showed no formation of byproducts.
Table 3 CeO2-catalyzed ester formation from benzamide and various alcohols
Finally, we studied reusability of the catalytic system. After the reaction of n-valeramide with benzylalcohol (entry 1 in Table 2), the catalyst was separated from the reaction mixture by centrifugation. The recovered catalyst was washed with acetone, followed by drying in air at 90 °C for 1 h. As shown in Fig. 1, the recovered catalyst showed high yields (89–95%) in the successive 4 cycles. Although the yield was slightly decreased at 4th run, the activity was recovered in the 5th and 6th cycles simply by calcination (600 °C, 0.5 h, in air) of the catalyst after 4th run. The present system is truly heterogeneous catalytic system as evidenced by the following results. For the reaction of n-valeramide and benzylalcohol, we removed the catalyst from the reaction mixture at the initial stage of the reaction (28% yield after 1 h). Further continuation of the reaction under reflux conditions did not increase the product yield. ICP analysis of the filtrate confirmed that the content of Ce in the solution was below the detection limit. These results indicate that CeO2 is a leaching-resistant and reusable heterogeneous catalyst for this reaction.
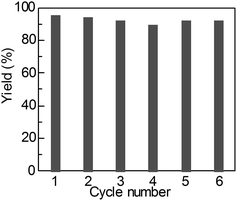 |
| Fig. 1 Catalyst reuse for the reaction of n-valeramide with benzylalcohol at 165 °C (22 h). Conditions are same as in Table 2. | |
Conclusion
We found CeO2-catalyzed esterification between equivalent molar ratios of amide and alcohol. This is the first additive-free and reusable heterogeneous catalytic system for direct alcoholysis of amides to esters with wider scope and 67 times higher TON than the previous homogeneous catalyst, and thus it provides a sustainable, general, and economic synthetic method of a wide range of esters.
Experimental section
General
Commercially available organic and inorganic compounds (from Tokyo Chemical Industry, Wako Pure Chemical Industries, Kishida Chemical, or Mitsuwa Chemicals) were used without further purification. The GC (Shimadzu GC-14B) and GCMS (Shimadzu GCMS-QP2010) analyses were carried out with Ultra ALLOY capillary column UA+-5 (Frontier Laboratories Ltd.) using nitrogen as the carrier gas.
Catalyst
CeO2 (JRC-CEO3, surface area = 81 m2 g−1) pre-calcined at 600 °C before being supplied from the Catalysis Society of Japan was used as a standard catalyst. Other metal oxides (CaO, MgO, ZnO, Y2O3, TiO2, ZrO2, Nb2O5, Al2O3, SiO2), commercially available or supplied from the Catalysis Society of Japan, where calcined at 500 °C for 3 h. These metal oxides were stored under air and used without any pretreatment. Solid acid HBEA was supplied from Catalysis Society of Japan, niobic acid (supplied by CBMM), Montmorillo-nite K10 clay (mont. K10), a sulfonic resin (Amberlyst-15) and Nafion-SiO2 were purchased from Aldrich.
Typical procedures of catalytic test
The mixture n-valeramide (1.0 mmol) and benzylalcohol (1.0 mmol) in mesitylene (1.5 g) was injected to the catalyst inside the reactor (cylindrical glass tube), followed by filling N2. Then, the resulting mixture was magnetically stirred for 22 h under reflux condition; the bath temperature was 180 °C and reaction temperature was ca. 165 °C. After cooling the mixture, followed by removal of the catalyst, the mixture was isolated by column chromatography using silica gel 60 (spherical, 63-210 μm, Kanto Chemical Co. Ltd.) and the eluting solvent of hexane–ethylacetate (19
:
1) and analyzed by 1H NMR, 13C NMR and GCMS. For the standard reaction of n-valeramide and benzyl alcohol for catalyst screening, catalyst recycle, conversion and yields of products were determined by GC using n-dodecane as an internal standard adopting the GC sensitivity estimated using the isolated product.
Acknowledgements
This work was supported by a MEXT program “Elements Strategy Initiative to Form Core Research Center” (since 2012), Japan.
References
- H. A. Taylor and T. W. Davis, J. Phys. Chem., 1928, 32, 1467 CrossRef CAS.
- W. L. Greenlee and E. D. Thorsett, J. Org. Chem., 1981, 46, 5351 CrossRef CAS.
- L. E. Fisher, J. M. Caroon, S. R. Stabler, S. Lundberg and S. Zaidi, Can. J. Chem., 1994, 72, 142 CrossRef CAS.
- E. H. White, J. Am. Chem. Soc., 1954, 76, 4497 CrossRef CAS.
- D. M. Shendage, R. Fröhlich and G. Haufe, Org. Lett., 2004, 6, 3675 CrossRef CAS PubMed.
- D. T. Glatzhofer, R. R. Roy and K. N. Cossey, Org. Lett., 2002, 4, 2349 CrossRef CAS PubMed.
- P. L. Anelli, M. Brocchetta, D. Palano and M. Visigalli, Tetrahedron Lett., 1997, 38, 2367 CrossRef CAS.
- A. B. Charette and P. Chua, Synlett, 1998, 163 CrossRef CAS PubMed.
- L. C. Li, J. Ren, T. G. Liao, J. X. Jiang and H. J. Zhu, Eur. J. Org. Chem., 2007, 1026 CrossRef CAS PubMed.
- M. C. Bröhmer, S. Mundinger, S. Bräse and W. Bannawarth, Angew. Chem., Int. Ed., 2011, 50, 6175 CrossRef PubMed.
- J. Aubé, Angew. Chem., Int. Ed., 2012, 51, 3063 CrossRef PubMed.
- S. Yamada, Angew. Chem., Int. Ed., 1993, 32, 1083 CrossRef PubMed.
- M. Hutchby, C. E. Houlden, M. F. Haddow, S. N. G. Tyler, G. C. Lloyd-Jones and K. I. Booker-Milburn, Angew. Chem., Int. Ed., 2012, 51, 548 CrossRef CAS PubMed.
- Y. Kita, Y. Nishii, T. Higuchi and K. Mashima, Angew. Chem., Int. Ed., 2012, 51, 5723 CrossRef CAS PubMed.
- Y. Kita, Y. Nishii, A. Onoue and K. Mashima, Adv. Synth. Catal., 2013, 355, 3391 CrossRef CAS PubMed.
- M. Tamura, H. Wakasugi, K. Shimizu and A. Satsuma, Chem.–Eur. J., 2011, 17, 11428 CrossRef CAS PubMed.
- M. Tamura, T. Tonomura, K. Shimizu and A. Satsuma, Green Chem., 2012, 14, 984 RSC.
- M. Tamura, T. Tonomura, K. Shimizu and A. Satsuma, Appl. Catal., A, 2012, 417, 6 CrossRef PubMed.
- M. Tamura, T. Tonomura, K. Shimizu and A. Satsuma, Green Chem., 2012, 14, 717 RSC.
- M. Tamura, S. M. A. H. Siddiki and K. Shimizu, Green Chem., 2013, 15, 1641 RSC.
- The number of Ce cations on the surface of CeO2 was calculated with the density of the Ce atom on the (111) surface and the surface area of CeO2.
Footnote |
† Electronic supplementary information (ESI) available. See DOI: 10.1039/c4ra07419j |
|
This journal is © The Royal Society of Chemistry 2014 |