DOI:
10.1039/C4RA07004F
(Paper)
RSC Adv., 2014,
4, 50153-50162
Design, synthesis and preliminary evaluation of a novel SPECT DTPA-bis-triazaspirodecanone conjugate for D2 receptor imaging†
Received
11th July 2014
, Accepted 19th September 2014
First published on 19th September 2014
Abstract
On the basis of pharmacological behaviour, dopamine receptors are divided into D1 and D2 subtype, which are primarily responsible for several neurophysiological anomalies. Assessment and validation with SPECT based conjugates provides a promising and cost effective insight to detect molecular changes in such neurological diseases. Spiperone, a well known “butryophenone” based D2 receptor antagonist, has been explored to design a novel conjugate for SPECT evaluation. The molecular docking pose analysis of the designed molecule was explored with dopamine D2 receptor. The molecule showed high affinity (−51.318 kcal mol−1) due to conserved interactions with Asp114 and Phe389 of D2 receptor. DTPA with triazaspirodecanone moiety of spiperone was synthesized using bifunctional chelation approach with 88% yield and has been characterized using NMR and mass spectroscopy. The radiolabeling efficiency of 99mTc-DTPA-bis-(1-phenyl-1,3,8-triazaspiro[4,5]decan-4-one) was 98%. The complex showed appreciable brain uptake in mice. Receptor binding experiments revealed a Kd of 6.26 nM with maximum localization of the conjugate in the striatum. Thus, these studies could be viewed as novel and informative, initial proof-of-concept approach to the field of 99mTc-labeled radioligand design.
Introduction
Several single photon emission computed tomography (SPECT) and positron emission tomography (PET) (S)-(pyrrolidinyl)methyl-2-methoxybenzamides-based agents, such as [123I]IBZM,1 [11C]raclopride,2,3 [18F]fallypride,4 [18F]haloperidol,5 and [18F]spiperone,6,7 etc. are routinely used for imaging D2/D3-receptor availability in the human brain. These agents are also used to study neurological disorders.5 The dopamine D2 receptor led to a repeated confirmation that it is the primary site of action for all antipsychotics.8,9 The affinity of antagonists toward a receptor and their occupancy in vitro and in vivo have a strong correlation because they provide important pharmacological tools for the determination of different roles of a receptor.10 The D1- and D2-R genes have the most widespread and highest levels of expression among the dopamine receptors.11
Spiperone is a butryophenone derivative and a well-known D2 receptor antagonist.12,13 Spiperone analogues have also been explored as biomarkers with various radiometals for the assessment of D2 receptor imaging.6,14,15 The triazaspirodecanone moiety of this potent D2 receptor antagonist serves as an efficient organic chelator and has been derivatized with appreciable binding activity.6 Despite significant chemical modification in the structure of the original spiperone agent, the triazaspirodecanone acts as an affinity, selectivity and activity preserver for the target receptors.14,15 This moiety has been conjugated for the synthesis of specific radiopharmaceuticals with the DOTA (1,4,7,10-tetraazacyclododecane-N,N′,N′′,N′′′-tetraacetic acid) cyclic chelating agents for D2 receptor imaging.15 The relationship between the D2 receptor occupancy and brain activity can be well-monitored using common imaging modalities like SPECT, PET and magnetic resonance imaging (MRI).16–18 The routine application of the 11C and 18F-labeled PET derivatives are limited due to the steep cost, cumbersome methodology and the requirement for a cyclotron to produce these nuclides.19 We have utilized the dimeric triazaspirodecanone part of spiperone for the synthesis of the reported SPECT-based agent.
These ligands/pharmacophores with the active moiety can be derivatized with a chelator and then used as specific non-invasive probes for diagnostic imaging purposes.20 The development of diagnostic and therapeutic radiopharmaceuticals has taken a new turn with the design of bifunctional chelating agents (BFCAs). Diethylene triamine pentaacetic acid (DTPA), a polydentate ligand with the ease of commercial availability, provides an appropriate framework for the entrapment of Technetium-99m (99mTc).21 DTPA has been extensively used as a metal chelator in the development of radiopharmaceuticals due to the facile nature of synthesis involved.22 Many structural variants with DTPA as a bifunctional chelator have been synthesized using various radiometals and biomarkers for spanning the abundance and paucity of D2 receptors in the brain.23,24 However, limiting stability and affinity for the target site demands the synthesis of conjugates with higher stability and affinity to the desired receptor. This limitation prompted us to explore the efficiency of triazaspirodecanone with DTPA. DTPA as a choice for the right bifunctional chelating agent can considerably affect the radiolabeling kinetics and can also increase the stability of the synthesized compounds, and to some degree influence the pharmacokinetics with 99mTc.25
99mTc is one of the most desirable radionuclides for imaging in diagnostic nuclear medicine because of the emission of gamma rays of optimal energy (140 keV), a suitable half-life (6 h), and availability from 99Mo-99mTc generator systems. The longer half-life of 99mTc, which broadens the observational time window and the simplicity of the kit chemistry, are the main reasons for the wide use of SPECT imaging.26 The first Tc-99m-labeled dopamine transporter imaging agent was ([99mTc]TRODAT-1).27 The 99mTc-SPECT analogs that are highly selective for dopaminergic neurotransmitter receptor sites are uncommon. A 99mTc labeled with medium-affinity D2-postsynaptic receptor ligand would allow a cost-effective technique for neurological disorders.
Herein, we report the design, synthesis and biological evaluation of the bifunctionally chelated DTPA-bis-(1-phenyl-1,3,8-triazaspiro[4,5]decan-4-one). The conjugate was radiolabeled with 99mTc to evaluate the in vitro and in vivo stability to affirm its suitability as an imaging agent.
Results and discussion
We have described the design and synthetic procedure for enhanced D2 target specificity with bis-pharmacophores conjugated to DTPA. The 3D structure of dopamine receptors D2 was modeled and validated using the crystal structure 3PBL. After the optimization of model and the docking active site, docking studies were performed for the ligand dataset and structural validations has been performed for DTPA-bis(1-phenyl-1,3,8-triazaspiro[4,5]decan-4-one) on the basis of docking performance and binding affinity. The results of the dataset and DTPA-bis conjugate involves ranking of the ligands posse as per the Glide XP score and Emodel. The further calculations of Prime MM-GBSA binding affinity was performed on the screened molecule. The DTPA-bis(1-phenyl-1,3,8-triazaspiro[4,5]decan-4-one) shows a good binding pose with an XP Gscore of −7.719 corresponding to the contribution of the bis triazaspirodecanone moieties that were used for the synthesis (Fig. 1). The aromatic moieties of the triazaspirodecanone molecule were involved in the π–π stacking interactions with Phe389 and Tyr39 in addition to a hydrogen bond with the backbone of the Asp114 with NH (imidazolidinone moiety), side chain hydrogen-bonding interactions of the carbonyl group (DTPA backbone) with Ile184 and a hydrogen bond with the side chain of Glu99 (Fig. 2). These interactions resulted in the high affinity binding of −51.318 kcal mol−1. These interactions are enhanced due to the presence of bis-triazaspirodecanone moieties in the DTPA-bis(1-phenyl-1,3,8triazaspiro[4,5]decan-4-one) molecule compared with the native 1-phenyl-1,3,8-triazaspiro[4,5]decan-4-one (Fig. S1; see the ESI†). The designed DTPA-bis(1-phenyl-1,3,8-triazaspiro[4,5]decan-4-one) shows the conserved interaction of the hydrogen bonding of Asp114 and π–π stacking interactions with Phe389 as compared with the known spiperone analogues (Fig. S2 and S3, ESI†). The results reflect the importance of incorporating the bis-triazaspirodecanone moieties in our ligand for increased D2 receptor affinity. These conserved residue interactions with Asp114 and π–π stacking interactions with Phe389 were available in all the ligand dataset molecules, which validates the skeleton of our molecule for high affinity to D2 receptor.
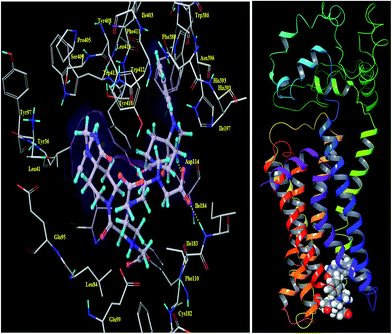 |
| Fig. 1 Three-dimensional view of the DTPA-bis-(1-phenyl-1,3,8-triazaspiro[4,5]decan-4-one) with a D2 homology-modeled receptor. | |
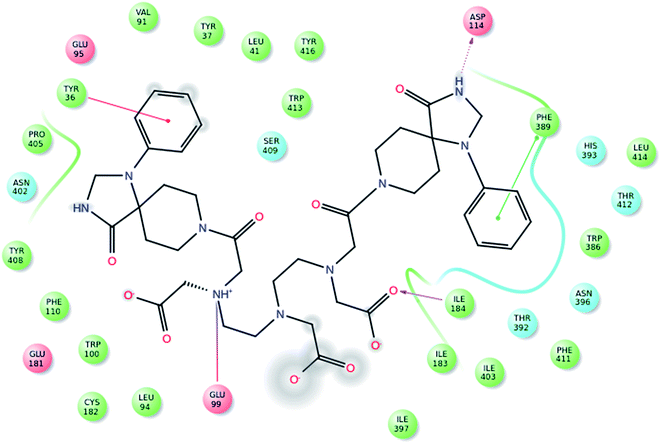 |
| Fig. 2 Two-dimensional view of DTPA-bis-(1-phenyl-1,3,8-triazaspiro[4,5]decan-4-one) with D2 receptor, where green-colored were hydrophobic, light blue were polar and red were positively charged residues. Pink lines represent hydrogen bonds, green lines for pi–pi stacking. | |
The DTPA-bis(1-phenyl-1,3,8-triazaspiro[4,5]decan-4-one) was synthesized by reacting DTPA bis anhydride with 1-phenyl-1,3,8-triazaspiro[4,5]decan-4-one (Scheme 1). The conjugate was obtained in 88% yield after HPLC purification. The compound was characterized by its retention time by analytical RP-HPLC. For DTPA-bis(1-phenyl-1,3,8-triazaspiro[4,5]decan-4-one), the retention time was Rt = 8.9 min and it was successfully characterized by IR, proton and carbon NMR and mass spectrometry. The DTPA-bis(1-phenyl-1,3,8-triazaspiro[4,5]decan-4-one) was evaluated for its ability to induce cytotoxicity on the HT22 cell line using a methylthiazole tetrazolium (MTT) assay. The cells exposed to DTPA-bis-pharmacophore conjugate showed concentration-dependent cell death that was statistically significant above 250 μM. The IC50 value of the DTPA-bisconjugate was 249.51 ± 0.38 μM (Fig. 3). The high value of IC50 accounted for low receptor affinity because the hippocampal HT22 cell line has been reported to show low endogenous D4 receptor expression.22,24 The working concentration of the radiolabeled conjugate was 20 μM and hence with an IC50 value of 250 μM, the synthesized conjugate can be nontoxic and safe to be used as a diagnostic agent.
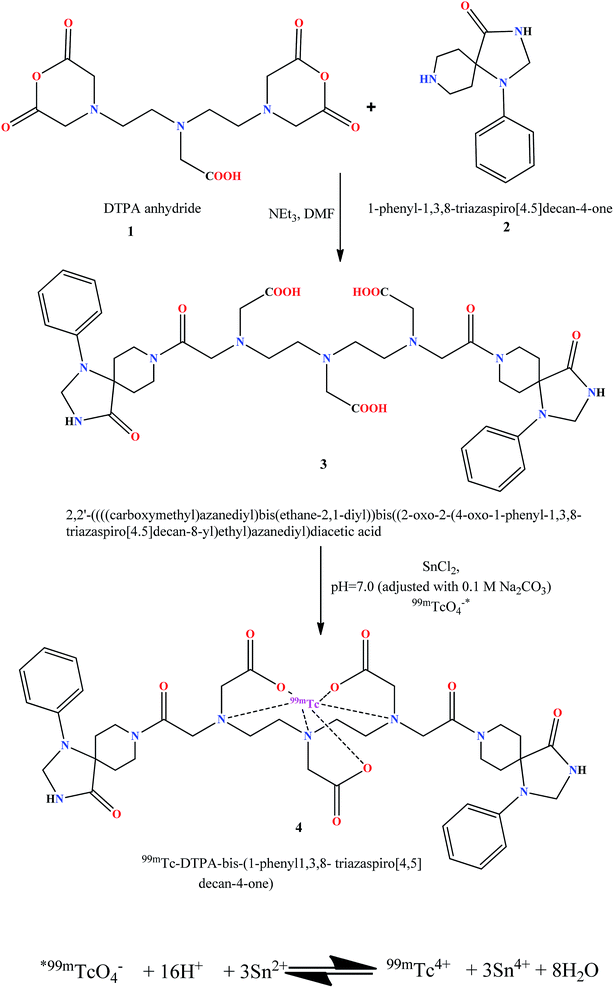 |
| Scheme 1 Synthesis of DTPA-bis-(1-phenyl-1,3,8-triazaspiro[4,5]decan-4-one). | |
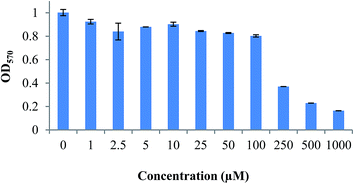 |
| Fig. 3 Cells from the HT-22 cell line were treated with various concentrations of DTPA-bis-(1-phenyl-1,3,8-triazaspiro[4,5]decan-4-one) for duration of 24 h; at the end of the treatment period, cell viability was measured using an MTT assay. Values are the means of three different experiments ± SE, each comprising six wells/experiment. (*p < 0.05)*. *error that is considered as statistically insignificant. | |
The conjugate was radiolabeled with 99mTc; the radiolabeling yield was found to be 98%, which included reduced/hydrolyzed technetium (0.2%) that was purified by a C-18 reversed phase extraction cartridge. The after-purification radiochromatogram on ITLC and radio-TLC showed one peak, and thus the effective radiochemical yield was 99.9% yield (Fig. S4 and S5, ESI†). The stability of radiolabeled compounds is one of the major problems in formulating radiopharmaceuticals. The radiolabeled compounds should be stable under both in vitro and in vivo conditions. The stability of many compounds will be affected by temperature, pH, and light effects, and the optimal range of these physicochemical conditions must be established for the preparation and storage of labeled compounds.6 The labeled compound in the present study was highly stable under in vitro and in vivo conditions with only 4.6% and 4.9% of the radiolabeled conjugate dissociated at 4 h, respectively (Fig. S6, ESI†). The complex formed by the chelation of 99mTc with the synthesized DTPA derivative was found to be stable, as suggested by in vitro serum stability and cysteine challenge tests. The transcomplexation studies performed with the different concentrations of cysteine confirmed that the compound was stable as approximately 95% of the radioactivity remained bound to the conjugate even at 100 mM concentration. Radiolabeled conjugates challenged with proteins (albumin and transferrin) present in the serum proved that 99mTc-DTPA-bis(1-phenyl-1,3,8-triazaspiro[4,5]decan-4-one) was sufficiently stable up to 24 h because only 9% of the radiolabeled conjugate dissociated in serum at 24 h.
The affinity of the 99mTc-DTPA-bis conjugate for D2 receptor imaging was assessed by receptor binding assay in striatum dissected from a mice brain. Binding curve analysis exhibited saturable binding of the radioconjugate in the nanomolar range. The value of Kd for 99mTc-DTPA-bis conjugate calculated from the Scatchard plot was 6.26 nM (Fig. 4). The competitive binding assay with a D1 [(+)-R-SCH-23990] and D2 (domperidone) antagonist exhibit 1000-fold more D2 (39.8 nM) affinity compared with D1 (>1000 nM) (Table 1). These results imply that despite being significantly modified from its native structure, the 99mTc-labeled bis-triazaspirodecanone based conjugate manages to show appreciable binding towards a D2 receptor. The results clearly validate our choice of selecting triazaspirodecanone as an efficient SPECT imaging agent. The molecule is chemically modified to form an efficient SPECT agent for brain receptor. The bifunctional chelation approach works very well for achieving higher lipophilic character in the conjugate to permeate the blood brain barrier, resulting in brain tissue uptake.
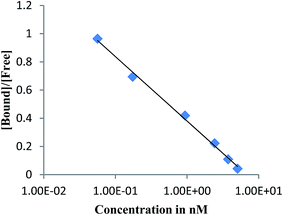 |
| Fig. 4 Scatchard plot of the specific binding data to the ratio of bound to free (B/F) radiopharmaceutical in rat striatum. | |
Table 1 Dissociation constants (Kd) of 99mTc-DTPA-bis-(1-phenyl-1,3,8-triazaspiro[4,5]decan-4-one) for D1 and D2 receptorsa
Complex |
D*2 |
D**2Kd (nM) |
D***1 |
Non specific binding was determined by * spiperone,** domperidone,*** [(+)-R-SCH-23990]. |
99mTc-DTPA-bis-(1-phenyl-1,3,8-triazaspiro[4,5]decan-4-one) |
6.2 nM |
39.8 nM |
>1000 nM |
The 99mTc-DTPA-bisconjugate shows a biphasic clearance with a biological half-life of t1/2 (fast), 16 min; and t1/2 (slow) 11 h. There was rapid clearance of the conjugate as only 15.7% activity remained in the circulation at 30 min and only 1.7% remains in the blood after 24 h time span (Fig. 5). The biodistribution pattern of the 99mTc-DTPA-bisconjugate carried out in BALB/C mice displays uptake in the brain at 5 min post-injection (0.84% ID/g) (Fig. 6). This was also validated by scintigraphic images that display an appreciable accumulation of radioconjugate in the rabbit brain in early images. Dynamic imaging performed in the rabbit showed the radiolabeled uptake in brain in early images as well (Fig. 7). High uptake in the kidneys (13.96 ± 1.02 at 15 min post-injection) and excretion through the urinary bladder was also observed, indicating that the major route for excretion was renal. The appreciable uptake was also shown by the liver (4.17 ± 1.1 at 15 min post-injection), which suggests partial clearance through the hepatobiliary route.
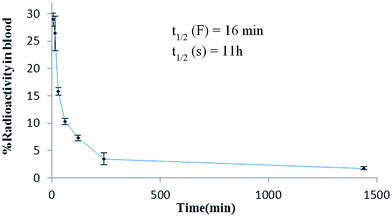 |
| Fig. 5 Blood clearance of 99mTc-DTPA-bis-(1-phenyl-1,3,8-triazaspiro[4,5]decan-4-one) administered through the ear vein in normal rabbits. | |
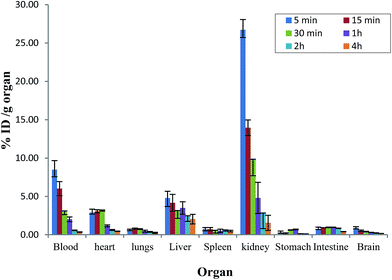 |
| Fig. 6 Biodistribution studies of 99mTc-DTPA-bis-(1-phenyl-1,3,8-triazaspiro[4,5]decan-4-one) in BALB/c mice. Data from four groups of five mice each expressed as mean % ID per g ± S.D. | |
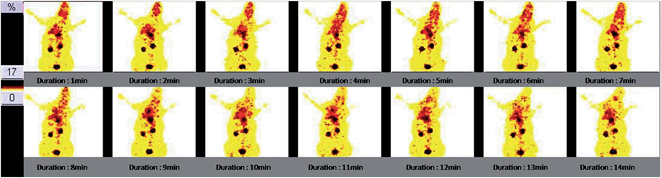 |
| Fig. 7 Dynamic images of a normal rabbit up to 15 min post injection of 99mTc labeled DTPA-bis-(1-phenyl-1,3,8-triazaspiro[4,5]decan-4-one). The early phase shows uptake in the brain. | |
The D2 receptor has been found mainly in the striatum region of the brain, where it is expressed by the GABAergic neurons followed by the cortex region.28 The high density of this receptor in these regions was assessed by a time activity curve. The activity in different parts of the brain was calculated as a percentage with respect to the entire brain activity. 41% of total brain uptake observed in the striatum region at 5 min shows selective preference of the synthesized conjugate for D2-rich regions. This value is followed by a decreasing uptake in the cortex (29% and 26%) and cerebellum (17% and 14%) at 5 and 15 min, respectively. The decreasing value of percentage radioactivity in the cerebellum with time indicates nonspecific binding because it is commonly assumed that radioactivity in the cerebellum measures non-specific binding.29 The total uptake in the brain, as shown in the time–activity curve, remains in concordance with the biodistribution results (Fig. 8). The regional distribution and blocking experiment with a known D2 receptor (haloperidol) in the rat brain was also studied (Table 2). The experiment conducted showed low uptake (<1) in the striatum, cortex and cerebellum. However, pretreatment with the haloperidol dose relatively reduced the ratio of striatum, cortex and cerebellum, suggesting the blockade for the specific D2 receptor binding.
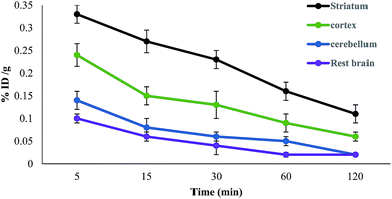 |
| Fig. 8 Time activity curve of 99mTc-DTPA-bis-(1-phenyl-1,3,8-triazaspiro[4,5] decan-4-one) in different parts of the BALB/c mice brain. | |
Table 2 Regional distribution of radioactivity 99mTc-DTPA-bis-(1-phenyl-1,3,8-triazaspiro[4,5] decan-4-one) in rat braina
Region |
5 minutes post-injection |
30 minutes post-injection |
30 minutes post-injection-HPb |
Each value is mean ± S.D., n = 3. Rats pretreated with 200 μg of haloperidol before 99mTc-DTPA-bis-(1-phenyl-1,3,8-triazaspiro[4,5]decan-4-one). |
Striatum (STR) |
0.024 ± 0.005 |
0.009 ± 0.001 |
0.003 ± 0.001 |
Cortex (CTX) |
0.021 ± 0.004 |
0.006 ± 0.002 |
0.002 ± 0.001 |
Cerebellum (CB) |
0.052 ± 0.01 |
0.012 ± 0.003 |
0.005 ± 0.002 |
![[thin space (1/6-em)]](https://www.rsc.org/images/entities/char_2009.gif) |
Ratios |
STR/CB |
0.461 ± 0.11 |
0.75 ± 0.16 |
0.6 ± 0.13 |
CTX/CB |
0.4 ± 0.12 |
0.5 ± 0.15 |
0.4 ± 0.11 |
STR/CTX |
1.14 ± 0.38 |
1.5 ± 0.41 |
1.5 ± 0.40 |
Experimental
Materials and methods
All the reagents and solvents used were of the purest grade as available commercially without further purification. DTPA bis anhydride, 1-phenyl-1,3,8-triazaspiro[4,5]decan-4-one, domperidone and haloperidol were purchased from Sigma. [(+)-R-SCH-23990] was purchased from Tocris Biosciences, USA. 99mTc was procured from the Regional Centre for Radiopharmaceuticals (Northern Region), Board of Radiation and Isotope Technology (BRIT), Institute of Nuclear Medicine and Allied Sciences (INMAS), DRDO, India. Column chromatography was carried out using silica MN60 (60–120), TLC sheets coated with silica gel 60, F254 (Merck).
Instrumentation
1H and 13C NMR spectra were recorded on a Bruker Avance II 400 MHz. Chemical shifts are reported relative to TMS. Mass spectra, electrospray ionization-mass spectrometry (ESI-MS) was performed on an Agilent 6310 ion trap LCMS system from Germany with ion trap detection in the positive and negative mode. HPLC analyses on an Agilent 1200 LC coupled to a UV detector at 265 nm with C-18 RP Agilent columns (250 × 4.6 mm, 5 μm, 1 mL min−1) using CH3CN and water are described in the text. The melting point was recorded on a Buchi M-560 apparatus, and the IR spectra were obtained using a Perkin Elmer FTIR SPECTRUM 2000 spectrophotometer. Lyophilisation was performed using a Labconco system. Radioactive samples were counted using a Capintech automated well-type counter. The scintigraphic imaging and biodistribution studies were conducted using a planar gamma camera Symbia TruePoint from Siemens. The scintigraphs were obtained using a rectangular large field of view gamma camera (Symbia TruePoint dual head) with a low energy all-purpose collimator.
Computational methodology
All the computational studies were performed using the Schrödinger Software; Maestro 9.7 (Schrödinger, LLC, New York, NY, 2014).30 The in silico analysis involved the homology modeling-assisted docking analysis and comparison of the ligand dataset6 and DTPA-bis-(1-phenyl-1,3,8-triazaspiro[4,5]decan-4-one) with the dopamine D2 receptor. Ligands were prepared by using a LigPrep tool30 that generates low energy conformers of ligands and all the possible protonation states at pH = 7.0 ± 2.0.
The human D2 sequence was retrieved from the SWISS-PROT database.31 Homology modeling was performed using Prime homology modeling tool of Schrödinger.30 The homology models of the dopamine receptors D2 was built and validated using the human dopamine receptor D3 crystal structure PDB:3PBL as template. The ClustalW alignment of D2 over the template was performed based on secondary structural predictions (SSPs) for the receptor. SSPs is supported with PSIPRED, which is best known for optimal prediction. Further, the receptor structure was obtained by building backbone atom coordinates for the aligned regions and side chains of conserved residues, optimization of side chains, and minimization of non-template residues, building of insertions and closing of deletions in the alignment. Finally, the structure was refined and protein preparation was performed using the Schrödinger's Protein Preparation Wizard. Further, active site residues were identified for performing grid generation and docking calculations using the Glide docking and binding affinity analysis.
Synthesis of DTPA-bis-(1-phenyl-1,3,8-triazaspiro[4,5]decan-4-one) (3)
DTPA anhydride 1 (0.15 g, 0.42 mmol) was dissolved in DMF (4 mL) and triethylamine (0.875 ml, 6.30 mmol) was added to the reaction vessel. The temperature of the reaction vessel was allowed to reach 65 °C under an inert nitrogen atmosphere. When the reaction vessel reached the desired temperature, 1-phenyl-1,3,8-triazaspiro[4,5]decan-4-one 2 (0.242 g, 1.05 mmol) was added and the mixture was stirred for 10 h. Completion of the reaction was checked by TLC plates in chloroform and methanol (7.5
:
2.5 v/v). The product was obtained by precipitation using acetone followed by centrifugation to give a white solid (0.301 g, 88%) (Scheme 1). Analytical RP-HPLC was performed on an Agilent HPLC System. An Agilent C-18 reverse phase column (4.6 × 250 mm) was used. The solvent system consisted of H2O (solvent A) and CH3CN (solvent B). Elution was achieved by applying a linear gradient from 90% A to 30% A in 20 min at a flow rate of 1 ml min−1. The retention time was Rt = 8.9 min. The product was obtained as a white-coloured fluffy solid after lyophilisation. The molecular mass of the pure DTPA derivative was determined by LCMS and HRMS. mp: 244.5 °C; IR absorptions: νmax cm−1: 3433.28 (NH, amide), 2928.03 (alkyl, CH), 1617.17 (C
O, acid and amide), 1401.56 (aromatic CH); δH (400 MHz; D2O) 7.18–7.08 (m, 4H), 6.90–6.72 (m, 4H), 6.61 (t, 2H), 4.47 (s, 2H), 3.86 (brs, 1H), 3.57 (s, 2H), 3.53–3.27 (m, 8H), 3.25–3.04 (m, 10H), 2.93–2.85 (m, 2H), 2.83 (s, 2H), 2.67 (s, 1H), 2.34–2.26 (m, 2H), 2.08–1.80 (m, 3H), 1.77–1.66 (m, 2H), 1.55–1.28 (m, 3H); δC (100 MHz; D2O) 178.23(2), 177.09, 170.40, 142.43, 129.62, 122.40, 119.44, 58.8(2), 57.49(2), 56.94, 52.85, 49.07, 40.52, 26.03; ESI-MS: m/z C40H53N9O10 found: 821.8 [M + 2H]+, 843.1 [M + Na]+, calculated = 819.39 [M]+; HRMS: m/z found: 820.3974 [M + H]+, 842.3792 [M + Na]+, calculated = 819.3915 [M]+.
Cell culture and cytotoxicity
HT22 cells (kindly gifted by Dr Dave Schubert, Salk Institute, San Diego, CA, USA to Dr RK Tulsawani), a murine immortalized hippocampal neuronal cell line, was maintained in DMEM medium containing 10% FBS and 1× antibiotic–antimycotic solution. Cells were seeded at a density of 10
000 per well in 96-well tissue culture plates and were allowed to adhere for 24 h at 37 °C. Then, cells were exposed to various concentrations (5–1000 μM) of DTPA-bis (1-phenyl-1,3,8-triazaspiro[4,5]decan-4-one)] dissolved in DMEM media for 24 h and period cell viability was evaluated using an MTT assay at the end of treatment. The MTT assay based on the conversion of yellow tetrazolium salt to purple-formazan crystals by metabolically active cells provides a quantitative determination of viable cells. Briefly, 50 μL of MTT solution (1 mg ml−1) was added to each well and incubated for 4 h at 37 °C. After the incubation period, formazan crystals were solubilized in 100 μL of DMSO. Absorbance was read at 570 nm with 630 nm as reference filter.
Radiolabeling of DTPA-bis-(1-phenyl-1,3,8-triazaspiro[4,5]decan-4-one)
DTPA-bis-(1-phenyl-1,3,8-triazaspiro[4,5]decan-4-one) (50 μL of 1 mg ml−1) was dissolved in a shielded vial and stannous chloride (150 μL), which was freshly dissolved in N2 purged 0.1 N HCl, was added. Freshly eluted (<1 h) 99mTc-pertechnetate (82 MBq; 200 μL) was added to the vial. The pH of the reaction mixture was adjusted to 7.0 with 0.1 M Na2CO3, purged with N2, mixed well and allowed to stand for 15 min at room temperature (25 °C).
Radiochemical purity of 99mTc-DTPA-bis-(1-phenyl-1,3,8-triazaspiro[4,5]decan-4-one)
The radiochemical purity of the labeled complex was determined by instant thin layer chromatography-silica gel (ITLC-SG, Paul Gelman, USA) strips as stationary phase in 100% acetone as the developing solvent. Using this method, the percentage of free Na99mTcO4−, reduced 99mTc and the complex formed between 99mTc and DTPA-bis-(1-phenyl-1,3,8-triazaspiro[4,5]decan-4-one) conjugate could be calculated. The labeled complex along with reduced/hydrolysed 99mTc remained at the base, whereas free pertechnetate moved with the solvent front into the acetone. The amount of reduced/hydrolysed 99mTc was estimated using pyridine
:
acetic acid
:
water (3
:
5
:
1.5) as the mobile phase and ITLC as the developing strip. The reduced/hydrolysed technetium remained at the point of application, whereas free 99mTc and labeled complex moved with the solvent front into the pyridine
:
acetic acid
:
water mobile phase. The activity was measured in a well-type gamma counter (CAPRAC R Capintec, USA) calibrated for 99mTc energy. The absolute radiochemical purity was calculated by subtracting the amount of reduced/hydrolysed 99mTc from the total labeled amount. The radiolabeled conjugate was then purified using a C-18 reversed phase extraction cartridge which was preconditioned with 10 mL methanol and subsequently activated with 30% methanol. The cartridge was successively rinsed with 5 mL distilled water, and the radiolabeled conjugate was eluted in 5 mL of 5% ethanol.
Cysteine challenge studies
Radiolabeled [DTPA-bis(1-phenyl-1,3,8-triazaspiro[4,5]decan-4-one)] complex was incubated with different concentrations of cysteine 25, 50, 100 mM at 37 °C up to 1 h. Periodically, aliquots were taken and spotted on a 10 cm ITLC-SG strip and developed in 0.1 M PBS (pH 7.4). Once the solvent front reached the end of the strip, it was cut into 1 cm fractions and analysed. The portions were analysed for radioactivity, and the amount of the conjugate that transchelated to cysteine was estimated (cysteine Rf was 0.7–0.8 in 0.1 M PBS).
Experimental animals
All animal experiments were performed in compliance with the relevant laws and the guidelines of INMAS (institutional committee, no. INM/DASQA/1AEC/09/015) animal ethics committee. The institutional committee approved the experiments. BALB/c mice were used for biodistribution and regional uptake studies. Rabbits of the New Zealand strain weighing 2.5 to 3.0 kg were used for the blood clearance, serum protein binding study and imaging. Sprague Dawley rats were used for regional uptake and receptor binding studies in the brain. The animals were randomly selected at from the stock colony maintained in the animal in-house facility. The animals were reared on laboratory chow pallets, fed ad libitum and had free access to food and water at all times. The room was maintained at 25 ± 2 °C with natural daytime light and no light after 19 h until morning. Mice were housed on a normal diet of sterile food pellets and water at a controlled temperature of 22 ± 2 °C.
In vitro and in vivo stability studies
For in vitro stability studies, aliquots of the radiolabeled complex were spotted on ITLC-SG paper at different time points and developed in 100% acetone. To perform in vivo stability studies, blood samples from rabbits were withdrawn during the experiments and the serum was separated and spotted on ITLC-SG that was developed in 100% acetone.
Human serum stability
Human serum was prepared by allowing blood collected from healthy volunteers to clot for 1 h at 37 °C in a humidified incubator maintained at 5% carbon dioxide/95% air. Then, the samples were centrifuged and the serum was filtered through a 0.22 μm syringe filter into sterile plastic culture tubes. 100 μL of radiolabeled [DTPA-bis(1-phenyl-1,3,8-triazaspiro[4,5]decan-4-one)] was incubated with 900 μL of serum at 37 °C up to 24 h. Aliquots at different time intervals were applied on ITLC-SG paper strips and run in acetone to check for any dissociation of the labeled complex.
In vitro receptor binding of 99mTc-[DTPA-bis(1-phenyl-1,3,8-triazaspiro[4,5]decan-4-one)]
The 99mTc-[DTPA-bis(1-phenyl-1,3,8-triazaspiro[4,5]decan-4-one)] was tested for in vitro affinity for D1 and D2 receptors by a radioligand binding assay. The specific ligand [(+)-R-SCH-23990] for D1 and domperidone for D2 were used. Non-specific binding was analyzed by nonlinear regression using algorithms in Graph Pad Prism 5.0 (San Diego, CA) and specific binding as the difference between the total and non-specific binding.
D2 receptor binding assay
The striatum of mice brain was homogenized and centrifuged at 20
000g for 10 min. The resulting pellet was resuspended with Ultra-Turrax and centrifuged again at 20
000g for 10 min. The same procedure was repeated. The pellet was then resuspended in 10 volumes of buffer and stored at −80 °C until used in binding studies. The final volume of the binding assay was 5.0 mL tris-HCl buffer (pH 7.4) containing 10 mM MgCl2, 1 mM EDTA, membrane homogenate (about 0.2 mg ml−1 protein), and the purified 99mTc-DTPA-bis(1-phenyl-1,3,8-triazaspiro[4,5]decan-4-one) conjugate was incubated with the striatum of mice brain homogenate and subsequently homogenized. The supernatant and subsequent washings were measured for their counts in the gamma counter. From the counts obtained for all the fractions, the binding constant of the conjugate was calculated from the Scatchard plot.
Blood clearance
Blood clearance studies were carried out in normal rabbits weighing about 2.5 to 3 kg and 0.3 ml (18.5 MBq) of 99mTc-1-phenyl-1,3,8-triazaspiro[4,5]decan-4-one was administered intravenously through the dorsal ear vein of the animal. Blood samples from the other ear veins were collected at different time intervals, and associated radioactivity was counted using a NaI well-type gamma counter (Caprac R Capintec, USA). The percentage radioactivity in the whole blood was calculated assuming the whole blood volume to be 7% of body weight.
Scintigraphy
Whole body images were obtained using a Siemens (Symbia TruePoint dual head) gamma camera. 99mTc-DTPA-bis(1-phenyl-1,3,8-triazaspiro[4,5]decan-4-one) imaging was performed after i.v. administration of 18.5 MBq radiotracer. The entire body (rabbit) scan was conducted at 5 min post-injection on a bed.
Biodistribution studies
The biodistribution study of 99mTc-DTPA-bis(1-phenyl-1,3,8-triazaspiro[4,5]decan-4-one) was performed on BALB/c mice (20–25 g). Mice were divided into six groups based on time point, each group having five mice. Then 100 μL 99mTc-DTPA-bis(1-phenyl-1,3,8-triazaspiro[4,5]decan-4-one) having activity 3.7 MBq was injected intravenously through the tail vein of each mouse. Mice were dissected at 5 min, 15 min, 30 min, 60 min, 120 min and 240 min post-injection. Different tissues freed from adhering tissues were taken out, weighed and counted in a γ-counter calibrated for 99mTc energy (Caprac R Capintec, USA). Uptake of the radiotracer in each tissue was calculated and expressed as percentage injected dose per gram of the tissue.
Regional brain uptake studies
The procedure followed was similar to that for the biodistribution studies. Briefly, the radiolabeled complex was injected in BALB/c mice (n = 3) and Sprague Dawley rats (n = 3). After euthanasia by an overdose of isoflurane inhalation at 5 min, 15 min, 30 min, 60 min and 120 min post-injection in mice and rats, the first and second group were sacrificed at 5 min and 30 min, respectively. For blocking studies, the third group of rats was treated with 200 μg haloperidol and after 30 min the radiolabeled complex was injected. This group was sacrificed at 30 min post-administration of the radiolabeled conjugate. The mouse and rat brain were isolated carefully and immediately washed with ice-cold PBS in respective experiments. Different parts of the brain were separated: the striatum, cortex, cerebellum and the rest of the brain. The radioactive counts were measured separately for all the parts after taking the decay correction factor into account.
Conclusion
The difference in the pharmacological behaviour of dopamine receptors has given us an enormous lead into understanding the approach to design radioligands, thereby gaining an insight into various neuropsychiatric disorders. Herein, we have successfully designed, synthesized and characterized a DTPA bis-triazaspirodecanone conjugate using the bifunctional chelation approach. This innovative and novel conjugate provides a SPECT imaging probe with the ease of synthesis, excellent radiolabeling yield, stability and purity. Furthermore, uptake in the brain followed by accumulation in the D2 receptor-rich regions with appropriate retention time validates our approach. Nevertheless, this work can be considered to be innovative from a radioligand design perspective.
Acknowledgements
We thank Dr R. P. Tripathi, Director, Institute of Nuclear Medicine and Allied Sciences, Defence Research and Development Organization Project no. INM 311 (3.1). We also wish to express our sense of gratitude to the Department of Chemistry, University of Delhi and CSIR for funding the research. The authors wish to acknowledge Dr R.K.Tulsawani and Mani of DIPAS, DRDO for providing assistance in MTT studies.
References
- N. P. Verhoeff, M. Bobeldijk, M. G. Feenstra, G. J. Boer, M. A. Maas, E. Ernste, K. de Bruin and E. A. Van Royen, Int. J. Radiat. Appl. Instrum., Part B, 1991, 18, 837–846 CrossRef CAS.
- L. Farde, L. Eriksson, G. Blomquist and C. Halldin, J. Cereb. Blood Flow Metab., 1989, 9, 696–708 CrossRef CAS PubMed.
- N. D. Volkow, G. J. Wang, J. S. Fowler, J. Logan, D. Schlyer, R. Hitzemann, J. Lieberman, B. Angrist, N. Pappas, R. MacGregor, G. Burr, T. Cooper and A. P. Wolf, Synapse, 2004, 16, 255–262 CrossRef PubMed.
- N. T. Vandehey, J. M. Moirano, A. K. Converse, J. E. Holden, J. Mukherjee, D. Murali, R. J. Nickles, R. J. Davidson, M. L. Schneider and B. T. Christian, J. Cereb. Blood Flow Metab., 2010, 30, 994–1007 CrossRef CAS PubMed.
- G. A. Pinna, M. M. Curzu, M. Sechi, G. Chelucci and E. Maciocco, Il Farmaco., 1999, 54, 542–550 CrossRef CAS.
- O. Prante, S. Maschauer and A. Banerjee, J. Labelled Compd. Radiopharm., 2013, 56, 130–148 CrossRef CAS PubMed.
- S. Nikolaus, R. Larisch, A. Wirrwar, M. Jamdjeu-Nouné, C. Antke, M. Beu, N. Schramm and H. W. Müller, Eur. J. Nucl. Med. Mol. Imaging, 2005, 32, 1305–1310 CrossRef CAS PubMed.
- A. L. Nordstrom, L. Farde, F. A. Wiesel, K. Forslund, S. Pauli, C. Halldin and G. Uppfeldt, Biol. Psychiatry, 1993, 33, 227–235 CrossRef CAS.
- F. A. Wiesel, L. Farde, A. L. Nordstrom and G. Sedvall, Prog. Neuro-Psychopharmacol. Biol. Psychiatry, 1990, 14, 759–767 CrossRef CAS.
- P. Grundt, S. L. Husbands, R. R. Luedtke, M. Taylor and A. H. Newman, Bioorg. Med. Chem. Lett., 2007, 17, 745–749 CrossRef CAS PubMed.
- D. Vallone, R. Picetti and E. Borrelli, Neurosci. Biobehav. Rev., 2004, 24, 125–132 CrossRef.
- A. L. Gundlach, B. L. Largent and S. H. Snyder, Life Sci., 1984, 35, 1981–1988 CrossRef CAS.
- J. E. Leysen, C. J. Niemegeers, J. P. Tollenaere and P. M. Laduron, Nature, 1978, 272, 168–171 CrossRef CAS.
- I. Zigelboim, D. Offen, E. Melamed, H. Panet, M. Rehavi and Y. Cohen, J. Inclusion Phenom. Macrocyclic Chem., 2007, 59, 323–329 CrossRef CAS.
- I. Zigelboim, A. Wiessberg and Y. Cohen, J. Org. Chem., 2013, 78, 7001–7012 CrossRef CAS.
- N. C. Andreasen, Science, 1988, 239, 1381–1388 CAS.
- C. Y. Sander, J. M. Hooker, C. Catana, M. D. Normandin, N. M. Alpert, G. M. Knudsen, W. Vanduffel, B. R. Rosen and J. B. Mandeville, Proc. Natl. Acad. Sci. U. S. A., 2013, 110, 11169–11174 CrossRef CAS PubMed.
- M. Robert, R. M. Kessler, J. R. Votaw, T. D. Paulis, D. R. Bingham, M. S. Ansari, N. S. Mason, G. Holburn, D. E. Schmidt, D. B. Votaw, R. G. Manning and M. H. Ebert, Synapse, 2004, 15, 169–176 Search PubMed.
- J. E. Bishop, C. A. Mathis, J. M. Gerdes, J. M. Whitney, A. M. Eaton and R. B. Mailman, J. Med. Chem., 1991, 34, 1612–1624 CrossRef CAS.
- R. H. Mach, J. R. Jackson, R. R. Luedtke, K. J. Ivins, P. B. Molinoff and R. L. Ehrenkaufer, J. Med. Chem., 1992, 35, 423–430 CrossRef CAS.
- S. Adak, R. Bhalla, K. K. Vijaya Raj, S. Mandal, R. Pickett and S. K. Luthra, Radiochim. Acta, 2012, 100, 95–107 CrossRef CAS.
- A. Safavy, D. C. Smith Jr., A. Bazooband and D. J. Buchsbaum, Bioconjugate Chem., 2002, 13, 317–326 CrossRef CAS PubMed.
- P. P. Hazari, K. Chuttani, N. Kumar, R. Mathur, R. Sharma, B. Singh and A. K. Mishra, Open Nucl. Med. J., 2009, 1, 33–42 CrossRef CAS.
- P. L. Mcgeer, J. C. Eccless and E. G. Mcgeer, in Molecular neurobiology of the mammalian brain, Plenum Press, New York and London, 2nd edn, 1987, ch. 9, pp. 284–308 Search PubMed.
- N. Singh, P. P. Hazari, S. Prakash, K. Chuttani, H. Khurana, H. Chandra and A. K. Mishra, Med. Chem. Commun., 2012, 3, 814 RSC.
- F. A. Metter and M. J. Guiberteau, in Essentials of Nuclear Medicine Imaging, Saunders, Elsevier, Amsterdam, 5th edn, 2006, ch. 1, pp. 1–13 Search PubMed.
- R. Varshney, S. K. Sethi, P. P. Hazari, K. Chuttani, S. Soni, M. D. Milton and A. K. Mishra, Curr. Radiopharm., 2012, 5, 348–355 CrossRef CAS.
- C. Missale, S. R. Nash, S. W. Robinson, M. Jaber and M. G. Caron, Physiol. Rev., 1998, 78, 189–225 CAS.
- B. Maziere, C. Loc'H, J.-C. Baraon, P. Sgouropoulos, N. Duquesnoy, R. D'antona and H. Cambon, Eur. J. Pharmacol., 1985, 114, 267–272 CrossRef CAS.
- L. L. C. Schrödinger, Prime version 2.2, Lig prep version 2.7, Glide version 6.0, QPLD, NY, New York, 2014 Search PubMed.
- B. Boeckmann, A. Bairoch, R. Apweiler, M. C. Blatter, A. Estreicher, E. Gasteiger, M. J. Martin, K. Michoud, C. O'Donovan, I. Phan, S. Pilbout and M. Schneider, Nucleic Acids Res., 2003, 31, 365–370 CrossRef CAS PubMed.
Footnote |
† Electronic supplementary information (ESI) available. See DOI: 10.1039/c4ra07004f |
|
This journal is © The Royal Society of Chemistry 2014 |