DOI:
10.1039/C4RA04165H
(Communication)
RSC Adv., 2014,
4, 30325-30331
Peptidomimetic organocatalysts: efficient Michael addition of ketones onto nitroolefins with very low catalyst loading†
Received
6th May 2014
, Accepted 19th June 2014
First published on 19th June 2014
Abstract
The syntheses of two novel peptidomimetic triazole-based organocatalysts that work for the asymmetric conjugate addition of cyclohexanone to nitroolefins are described. The catalysts worked with very low loading (0.5 mol%) in the absence of any additives to provide high diastereo- and enantio-selectivities.
The asymmetric organo-catalyzed aldol and Michael reactions have co-developed in the recent past and paved way for a new era in asymmetric synthesis.1 Major challenges in organocatalysis still to be addressed include the ease of access to catalyst and its cost, substrate generality and more importantly, catalyst loading. Many of the organocatalysts are substrate/reaction specific, involve multiple steps for synthesis, incorporate sensitive functionalities and are difficult to recover. Thus, the field is wide open for further innovations. The early examples of organocatalytic asymmetric transformations utilized 20 –50 mol% of proline as the catalyst.2 At that time, the recovery of the amino acid was not a criterion for most researchers. With the exploration of newer synthetic scaffolds as catalysts, which were available in smaller quantities, the recovery and recycling of the catalyst became a priority. In addition, experimentation with lower concentrations of catalysts were initiated to mimic reactions in nature. Several groups,3 including ours,4 working in the area of organocatalysis have developed catalysts, which are required in the ratios of 1 –10 mol% for a reaction to be fruitful. Recently, small and medium peptides have been established as organocatalysts.5 Our work on peptidomimetics and turn-inducing properties of the unusual β-amino acids6 inspired us to design and synthesize new scaffolds, which could exhibit improved organocatalytic properties. We conceived that the triazole ring, which is an amide bond surrogate,7 may contribute to form a rigid backbone conformation and thus promote selectivity. We further anticipated that the enhanced number of nitrogen atoms would also have an influence on the basicity of the catalysts. An additional amino-acid appendage as proposed by Wennemers et al.,8 would hopefully provide hydrogen bonding. With this background, we initiated the synthesis of pyrrolidine-linked triazole having an isoaspargine amino acid appendage by following the synthetic strategy as depicted in Scheme 1.
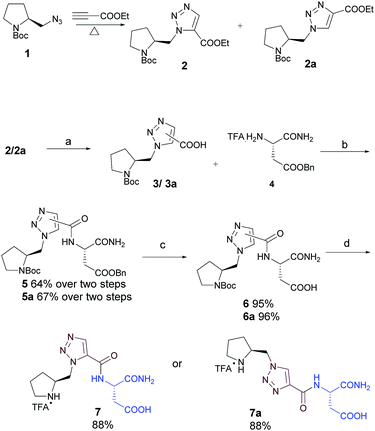 |
| Scheme 1 Synthesis of catalysts 7 and 7a. | |
Thus, known azidopyrrolidine 1,9 was subjected to a thermal Huisgen [3 + 2] cycloaddition in the presence of ethyl propiolate to furnish two regioisomeric triazoles 2 and 2a in 28
:
72 ratio, easily separable by chromatography. Both the isomers (1,5- and 1,4-disubstituted triazoles) were independently transformed to corresponding acids 3 or 3a followed by coupling with isoaspargine 4, under N-(3-dimethylaminopropyl)–N′-ethylcarbodiimide hydrochloride (EDCI), hydroxybenzotriazole (HOBt) conditions to realise pyrrolidine-triazoles 5 or 5a. Hydrogenation of benzyl ester to 6 or 6a followed by treatment with TFA furnished 7 or 7a.
Wennemers et al.8 have extensively studied organocatalysts based on turn-inducing D-proline–L-proline dipeptide10 and have recently reported a novel, highly efficient tripeptide organocatalyst, exhibiting a hydrogen bond with isoaspargine.
Some of the structural demands for high efficiency have been put forth by the group. These studies suggested that 2° amine at the N-terminus, the carboxylic acid side chain of the aspartic acid residue and a well-defined turn conformation are crucial for high catalytic activity and selectivity. Other groups working in the area of organocatalysis have proposed a different set of rules for activity, where the turn induction and/or hydrogen bonding are not featured.11 Thus, based on these studies, it can be inferred that organocatalysis works either by turn induction, thereby forming a pocket, or in a second instance, without these properties but with hydrogen bonding. The catalyst synthesized by Wennemers et al.8 falls in the first category, which works at a low concentration (<1.0 mol%). Computational models have also been put forth to generalise the essential requirements for organocatalytic properties, particularly for Michael-type reactions.11
Before embarking on studying the efficiency of the catalysts synthesized, a detailed NMR investigation was taken up for both compounds to understand the turn pattern and hydrogen-bonding properties. Information on the preferred conformation of 1,5-triazole catalyst has been obtained at a concentration of approximately 5 mM in two independent structure-supporting solvents: water (90% D2O + 10% H2O) and CD3OH, by using 1D and 2D (1H, COSY, TOCSY, and ROESY) NMR spectroscopy techniques. A significant number of intra-residue H–H ROEs is observed for 1,5-triazole catalyst 7. Specifically, the observed unambiguous ROEs (Fig. 1a) for 7: 10H–13H, 13H–(16Hi and 16Hj), 13H–17Hk, 14H–16Nhi, 10H–6Hh, 5H–6Hg, and 6Hg–2Hb are helpful in determining the conformation (Fig. 1a). In order to delineate low-energy structures, ROE-restrained molecular dynamics studies have been carried out using simulated annealing protocol (insight II). Initially the input structures are rapidly heated from 300 K to 900 K, allowing the structure to remain at 300 K, 600 K and 900 K for 5 ps. The velocity-scaling temperature-controlling method was adopted for heating, and the temperature was allowed to vary by an order of 10 K. The velocity verlet integration method was used for integration. Further, the structures were slowly cooled from 900 K to 300 K by allowing the structures to remain for 5 ps at 800 K, 700 K, 600 K, 500 K, 400 K and 300 K. The cycle of simulated annealing is repeated 250 times, and the final structures were stored. Fifteen low-energy structures were selected among the 250 conformations and superimposed. The structures predominantly show single conformation, which is a turn-like compact structure. A similar process followed for the 1,4-triazole catalyst 7a, also shown in the Fig. 1b, and the results showed that 7a adopts an extended conformation, in contrast to 7 (Fig. 1).
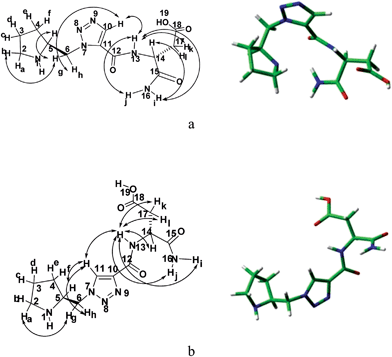 |
| Fig. 1 ROE-restrained minimum energy structures for catalyst 7 (1a) and 7a (1b). | |
The detailed NMR investigations followed by ROE-restrained molecular dynamics10 established that 1,5-disubstituted compound 7 adopts a relatively compact turn-like conformation (Fig. 1a), whereas the other isomer (1,4-disubstituted) 7a exhibited an extended conformation (Fig. 1b). Either of these properties is generally required for the asymmetric organocatalytic properties. To have sufficient material on hand, we have conducted experiments with ruthenium and copper catalysts, which exclusively gave 1,5- or 1,4-di-substituted-1,2,3-triazoles.12–14
It is anticipated that the observed turn structure of 1,5-triazole catalyst 7 may favour its terminal isoaspargine appendage to participate in hydrogen bonding with the nitro group of nitrostyrene, a stabilizing factor akin to that observed for D-Pro–L-Pro–Asp–NH2, for an improved catalytic performance of the catalyst.12 To prove this, a reaction was carried out between cyclohexanone (8) and nitrostyrene (9) in presence of catalyst 7 (5 mol%) and CH2Cl2 as solvent, which resulted in 10 in 55% yield with >99% er (Table 1, entry 1). To optimise the reaction conditions, the solvents used were water, acetonitrile, isopropanol and methanol (Table 1, entries 3, 5, 9 and 11). A neat reaction was also carried out with similar results (Table 1, entry 7). Among the solvents tried, methanol was found to give the optimal conditions of yield and selectivity. Therefore, all subsequent reactions were carried out in methanol. As the catalyst performed well at 5 mol%, we were interested to see the effect of further reduction in catalyst loading.
Table 1 Study of catalyst loading and solvent effect
At first, catalyst loading was decreased to 2 mol% and after obtaining results similar to 5 mol%, the loading of catalyst was further reduced to 1 mol% and then to 0.5 mol% (Table 1, entries 13, 15 and 17). To our surprise, even for 0.5 mol%, catalyst loading dr and er were retained. but the yield was slightly lower (95% to 80% for 0.5 mol%). These results surprised us as the use of 0.5 mol% of catalysts is rarely reported in literature for aldol reactions and conjugate addition of aldehydes but not for the Michael reaction of ketones to nitroolefins.15
A very low loading of the catalyst, the striking feature observed in this study, has prompted us to explore the scope of the catalyst by studying its effect on different substrates for wider application. Thus, substituted aromatic, heteroaromatic nitroolefins were coupled with cyclohexanone, 8, using catalyst 7, and it was observed that the outcome of these reactions was similar to the first reaction of nitrostyrene (Table 2, entries 1 to 10). We next changed the ketones to thiopyranone and acetone to get products in good to average yields (Table 2, entries 11 to 14). Based on these observations one can conclude that both electron-donating and -withdrawing groups had negligible effect on the stereoselectivity of the reaction. This confirms a wider applicability of the catalyst and non-participation of the substituents or ring structure of the nitroolefin.
Table 2 Substrate variation for catalysts 7 and 7a
Interestingly, catalyst 7a having no turn structure was only marginally inferior to 7 (Tables 1 and 2). DFT computations at the B3LYP/6-31G* level provided more insights into the catalytic activities of 7 and 7a.11,12 Standard protocols for observing transition states are followed, by using Gaussian 09 software. Initially, the fully relaxed minimum energy structures of 1,5-triazole catalyst 7, 1,4-triazole catalyst 7a and nitrostyrene obtained from simulated annealing are subjected to optimization at B3LYP/6-31*-level DFT calculations. The optimization was initially carried out in vacuum and then in a MeOD solvent, by adopting polarisable continuum model (PCM). These served as inputs (reactants) for computing transition-state structures.
The transition states for the addition of anti- and syn-enamine to the si and re faces of nitrostyrene were first located in the gas phase. These transition states are labelled a-re, a-si, s-re, and s-si. The noticeable charge separation in the transition state is expected upon the addition of enamine to nitrostyrene. To obtain improved estimates of the reaction, energetic continuum solvent effects are incorporated by computing the zero-point energies by using PCM with methanol as the medium. The preferred transition-state structures arising from anti-enamine for both the catalysts are depicted in Fig. 2.
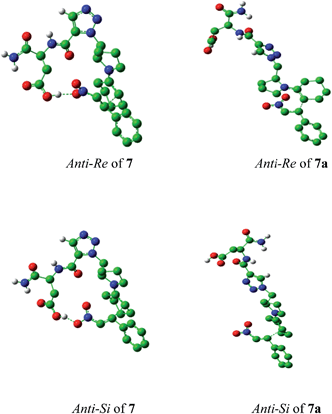 |
| Fig. 2 Preferred transition states for 7 and 7a. | |
On the basis of the DFT results, a mechanism is proposed to account for the observed stereoselectivity. The free acid of 7 behaves as a bifunctional catalyst. The proline ring first reacts with cyclohexanone carbonyl group to form an enamine with the help of an acidic co-catalyst. Subsequently, in the compact turn structure of 7, the proton of the terminal acid hydroxyl group interacts with the nitro group of nitrostyrene, through hydrogen-bonding. The transition state involving the re-face attack on the anti-enamine (Fig. 2) was lower in energy than other possible transition states (anti-si, syn-re, and syn-si). Whereas, in case of 7a, there is no hydrogen-bond interaction between the acid hydroxyl group of catalyst and the nitro group of nitrostyrene to provide an additional stabilisation factor, thus the 7a transition states are higher in energy than 7 (Table 3).
Table 3 Energies for the preferred transition states of 7 and 7a
Transition state |
Transition state energy (Hartree) |
Transition state energy (Kcal mol−1) |
Anti-re (7) |
−1844.567544 |
2.43 |
Anti-si (7) |
−1844.565963 |
3.42 |
Anti-re (7a) |
−1844.551983 |
12.75 |
Anti-si (7a) |
−1844.548347 |
15.07 |
Conclusions
In conclusion, we report the syntheses of two compounds, which have a peptide bond surrogate triazole between a pyrrolidine methylene and isoaspargine, which proved to be excellent catalysts for Michael-addition reactions. The low loading of the catalyst, a little explored area of organocatalysis, is the highlight of this work. Catalyst 7 appears to be following the mechanism established for tripeptides by a turn-like conformation and thereby an intra-molecular hydrogen bonding; 7a appears to be in the category of proline and proline-derived catalysts, which do not depend on turn-like conformation for activity. These results suggest that catalysts having turn-inducing and hydrogen-bonding properties would have slightly superior organocatalytic activity compared with catalysts having either one of the characters. The applications of these catalysts for other reactions are currently being explored.
Acknowledgements
C.P.K. thanks Prof. Helmut Hügel and Dr Julie Nieri of RMIT for their suggestions. T.P.K. thanks DST New Delhi for the INSPIRE Faculty Award (IFA12-CH-30). The authors thank CSIR and DST for funding.
Notes and references
-
(a) B. M. Trost and C. S. Brindle, Chem. Soc. Rev., 2010, 39, 1600–1632 RSC;
(b) Y. Zhang and W. Wang, Catal. Sci. Technol., 2012, 2, 42–53 RSC;
(c) J. Aleman and S. Cabrera, Chem. Soc. Rev., 2013, 42, 774–793 RSC;
(d) H. Pellissier, Tetrahedron, 2007, 63, 9267–9331 CrossRef CAS PubMed;
(e) O. M. Berner, L. Tedeschi and D. Enders, Eur. J. Org. Chem., 2002, 1877–1894 CrossRef CAS and references cited therein;
(f) A. Berkessel and H. Groger, Asymmetric Organocatalysis, Wiley-VCH, Weinheim, Germany, 2005 Search PubMed;
(g) T. Akiyama, J. Itoh and K. Fuchibe, Adv. Synth. Catal., 2006, 348, 999–1010 CrossRef CAS;
(h) P. Perlmutter, Conjugate Addition Reactions in Organic Synthesis, Pergamon, Oxford, 1992 Search PubMed;
(i) K. Sakthivel, W. Notz, T. Buli and C. F. Barbas III, J. Am. Chem. Soc., 2001, 123, 5260–5267 CrossRef CAS PubMed;
(j) B. List, P. Pojarliev and H. J. Martin, Org. Lett., 2001, 3, 2423–2425 CrossRef CAS PubMed;
(k) T. Tokoroyama, Eur. J. Org. Chem., 2010, 2009–2016 CrossRef CAS;
(l) A. Dondoni and A. Massi, Angew. Chem., 2008, 120, 4716–4739 (Angew. Chem., Int. Ed., 2008, 47, 4638–4660) CrossRef;
(m) U. Scheffler and R. Mahrwald, Chem.–Eur. J., 2013, 19, 14346–14396 CrossRef CAS PubMed;
(n) N. Krause and A. Hoffmann-Roder, Synthesis, 2001, 171–196 CrossRef CAS PubMed;
(o) O. M. Berner, L. Tedeschi and D. Enders, Eur. J. Org. Chem., 2002, 1877–1894 CrossRef CAS;
(p) J. Christoffers and A. Baro, Angew. Chem., Int. Ed., 2003, 42, 1688 CrossRef CAS PubMed;
(q) W. Notz, F. Tanaka and C. F. Barbas III, Acc. Chem. Res., 2004, 37, 580–591 CrossRef CAS PubMed;
(r) R. Ballini, G. Bosica, D. Fiorini, A. Palmieri and M. Petrini, Chem. Rev., 2005, 105, 933–972 CrossRef CAS PubMed;
(s) S. B. Tsogoeva, Eur. J. Org. Chem., 2007, 1701–1716 CrossRef CAS;
(t) S. Sulzer-Mosse and A. Alexakis, Chem. Commun., 2007, 3123–3135 RSC;
(u) A. Krkkila, I. Majander and P. M. Pihko, Chem. Rev., 2007, 107, 5416–5470 CrossRef PubMed;
(v) S. Mukherjee, J. W. Yang, S. Hoffmann and B. List, Chem. Rev., 2007, 107, 5471–5812 CrossRef CAS PubMed;
(w) E. A. C. Davie, S. M. Mennen, Y. Xu and S. J. Miller, Chem. Rev., 2007, 107, 5759–5812 CrossRef PubMed.
-
(a) Z. G. Hajos and D. R. Parrish, 2, 102, 623, Ger. Offen. (Hoffmann-La Roche, F., and Co., A. G. DE, 1971, pp. 42;
(b) Z. G. Hajos and D. R. Parrish, J. Org. Chem., 1974, 39, 1615–1621 CrossRef CAS.
-
(a) F. Giacalone, M. Gruttadauria, P. Agrigento and R. Noto, Chem. Soc. Rev., 2012, 41, 2406–2447 RSC;
(b) S. Zhu, S. Yu and D. Ma, Angew. Chem., Int. Ed., 2008, 47, 545–548 CrossRef CAS PubMed;
(c) C. Palomo, S. Vera, A. Mielgo and E. G. Mez-Bengoa, Angew. Chem., Int. Ed., 2006, 45, 59845987 Search PubMed;
(d) Y. Horiuchi, M. Taniguchi, K. Oshima and K. Utimoto, Tetrahedron Lett., 1995, 36, 5353–5356 CrossRef CAS;
(e) J. Burés, A. Armstrong and D. G. Blackmond, J. Am. Chem. Soc., 2012, 134, 14264 CrossRef;
(f) S. H. McCooey and S. J. Connon, Angew. Chem., Int. Ed., 2005, 44, 6367–6370 CrossRef CAS PubMed;
(g) B. Vakulya, S. Varga, A. Csampai and T. Soos, Org. Lett., 2005, 7, 1967–1969 CrossRef CAS PubMed;
(h) S. Mosse and A. Alexakis, Org. Lett., 2005, 7, 4361–4364 CrossRef CAS PubMed;
(i) Y. G. Chi and S. H. Gellman, Org. Lett., 2005, 7, 4253–4256 CrossRef CAS PubMed;
(j) Y. Hayashi, H. Gotho, T. Hayashi and M. Shoji, Angew. Chem., Int. Ed., 2005, 44, 4212–4215 CrossRef CAS PubMed;
(k) S. Z. Luo, L. X. Mi, L. Zhang, S. Liu, H. Xu and J. P. Cheng, Angew. Chem., Int. Ed., 2006, 45, 3093–3097 CrossRef CAS PubMed;
(l) S. V. Pansare and K. Pandya, J. Am. Chem. Soc., 2006, 128, 9624–9625 CrossRef CAS PubMed;
(m) N. Mase, K. Watanabe, H. Yoda, K. Takabe, F. Tanaka and C. F. Barbas III, J. Am. Chem. Soc., 2006, 128, 4966–4967 CrossRef CAS PubMed;
(n) E. Reyes, J. L. Vicario, D. Badia and L. Carrillo, Org. Lett., 2006, 8, 6135–6138 CrossRef CAS PubMed;
(o) M. L. Clarke and J. A. Fuentes, Angew. Chem., Int. Ed., 2007, 46, 930–933 CrossRef CAS PubMed;
(p) H. B. Chen, Y. Wang, S. Y. Wei and J. Sun, Tetrahedron: Asymmetry, 2007, 18, 1308–1312 CrossRef CAS PubMed;
(q) S. L. Zhu, S. Y. Yu and D. W. Ma, Angew. Chem., Int. Ed., 2008, 47, 545–548 CrossRef CAS PubMed;
(r) S. R. Ban, D. M. Du, H. Liu and W. Yang, Eur. J. Org. Chem., 2010, 5160–5164 CrossRef CAS;
(s) D. F. Lu, Y. F. Gong and W. Z. Wang, Adv. Synth. Catal., 2010, 352, 644–650 CrossRef CAS;
(t) W. Yang and D. M. Du, Adv. Synth. Catal., 2011, 353, 1241–1246 CrossRef CAS;
(u) S. Ikeda, M. Shibuya, N. Kanoh and Y. Iwabuchi, Org. Lett., 2009, 11, 1833–1836 CrossRef CAS PubMed;
(v) H. Ma, K. Liu, F. G. Zhang, C. L. Zhu, J. Nie and J. A. Ma, J. Org. Chem., 2010, 75, 1402–1409 CrossRef CAS PubMed;
(w) A. Nakamura, S. Lectard, D. Hashizume, Y. Hamashima and M. Sodeoka, J. Am. Chem. Soc., 2010, 132, 4036–4037 CrossRef CAS PubMed;
(x) B. C. Hong, P. Kotame, C. W. Tsai and J. H. Liao, Org. Lett., 2010, 12, 776–779 CrossRef CAS PubMed;
(y) M. Krayer, M. Ptaszek, H. J. Kim, K. R. Meneely, D. Fan, K. Secor and J. S. Lindsey, J. Org. Chem., 2010, 75, 1016–1039 CrossRef CAS PubMed.
-
(a) S. Chandrasekhar, T. P. Kumar, K. Haribabu, C. R. Reddy and C. R. Kumar, Tetrahedron: Asymmetry, 2011, 22, 697–702 CrossRef CAS PubMed;
(b) S. Chandrasekhar, T. P. Kumar, K. Haribabu and C. R. Reddy, Tetrahedron: Asymmetry, 2010, 21, 2372–2375 CrossRef CAS PubMed;
(c) S. Chandrasekhar, K. Mallikarjun, G. P. K Reddy, B. Jagadeesh and V. Mohan, Chem. Commun., 2009, 4985–4987 RSC;
(d) S. Chandrasekhar, B. Tiwari, B. B. Parida and C. Raji Reddy, Tetrahedron: Asymmetry, 2008, 19, 495–499 CrossRef CAS PubMed;
(e) T. P. Kumar and S. Chandrasekhar, Synthesis, 2012, 2889–2894 CAS;
(f) S. Chandrasekhar, N. Ramakrishna Reddy, S. Shameem Sultana, C. Narsimhulu and K. V. Reddy, Tetrahedron, 2006, 62, 338–345 CrossRef CAS PubMed;
(g) S. Chandrasekhar, K. Johny and C. R. Reddy, Tetrahedron: Asymmetry, 2009, 20, 1742–1745 CrossRef CAS PubMed;
(h) P. S. Mainkar, K. Johny, T. P. Rao and S. Chandrasekhar, J. Org. Chem., 2012, 77, 2519–2525 CrossRef CAS PubMed.
-
(a) J. A. Pearson and S. Panda, Org. Lett., 2011, 13, 5548 CrossRef PubMed;
(b) H. Wennemrs, Chem. Commun., 2011, 47, 12036–12041 RSC;
(c) F. Kolundzic, M. N. Noshi, M. Tjandra, M. Movassaghi and S. J. Miller, J. Am. Chem. Soc., 2011, 133, 9104–9111 CrossRef CAS PubMed;
(d) K. W. Fiori, A. I. Puchlopek and S. J. Miller, Nat. Chem., 2009, 1, 630–634 CrossRef CAS PubMed;
(e) S. B. Tsogoeva, S. B. Jagtap and Z. A. Ardemasova, Tetrahedron: Asymmetry, 2006, 17, 989–992 CrossRef CAS PubMed;
(f) M. Lei, S. Xia, J. Wang, T. Cheng and R. Li, Chirality, 2010, 22, 580–586 CAS.
-
(a) S. Chandrasekhar, M. S. Reddy, B. N. Babu, B. Jagadeesh, A. Prabhakar and B. Jagannadh, J. Am. Chem. Soc., 2005, 127, 9664–9665 CrossRef CAS PubMed;
(b) S. Chandrasekhar, M. S. Reddy, B. Jagadeesh, A. Prabhakar, M. H. V. Ramana Rao and B. Jagannadh, J. Am. Chem. Soc., 2004, 126, 13586–13587 CrossRef CAS PubMed;
(c) S. Chandrasekhar, N. Kiranmai, M. U. Kiran, A. S. Devi, G. P. K. Reddy, M. Idris and B. Jagadeesh, Chem. Commun., 2010, 46, 6962–6964 RSC;
(d) S. Chandrasekhar, A. Sudhakar, M. U. Kiran, B. N. Babu and B. Jagadeesh, Tetrahedron Lett., 2008, 49, 7368–7371 CrossRef CAS PubMed;
(e) S. Chandrasekhar, B. N. Babu, A. Prabhakar, A. Sudhakar, M. S. Reddy, M. U. Kiran and B. Jagadeesh, Chem. Commun., 2006, 1548 RSC;
(f) B. Jagadeesh, M. Udayakiran, A. Sudhakar and S. Chandrasekhar, Chem.–Eur. J., 2009, 15, 12592–12595 CrossRef CAS PubMed;
(g) S. Chandrasekhar, K. V. M. Rao, M. Seenaiah, P. Naresh, A. S. Devi and B. Jagadeesh, Chem.–Asian J., 2014, 9, 457–461 CrossRef CAS PubMed.
-
(a) V. D. Bock, D. S. Peijer, H. Hiemstra and J. H. van Maarseveen, Org. Biomol. Chem., 2007, 5, 971–975 RSC;
(b) M. Tischler, D. Nasu, M. Empting, S. Schmelz, D. W. Heinz, P. Rottmann, H. Kolmar, G. Buntkowsky, D. Tietze and O. Avrutina, Angew. Chem., Int. Ed., 2012, 51, 3708–3712 CrossRef CAS PubMed;
(c) I. E. Valverde, A. Bauman, C. A. Kluba, S. Vomstein, M. A. Walter and T. L. Mindt, Angew. Chem., Int. Ed., 2013, 52, 8957–8960 CrossRef CAS PubMed;
(d) V. D. Bock, R. Perciaccante, T. P. Jansern, H. Hiemstra and J. H. van Maarseveen, Org. Lett., 2006, 8, 919–922 CrossRef CAS PubMed;
(e) A. Choudhary and R. T. Raines, ChemBioChem, 2011, 12, 1801–1807 CrossRef CAS PubMed and reference cited therein.
-
(a) M. Wiesner, J. D. Revell and H. Wennemers, Angew. Chem., Int. Ed., 2008, 47, 1871–1874 CrossRef CAS PubMed;
(b) M. Wiesner, M. Neuburger and H. Wennemers, Chem.–Eur. J., 2009, 15, 10103–10109 CrossRef CAS PubMed;
(c) J. D. Revell and H. Wennemers, Adv. Synth. Catal., 2008, 350, 1046–1052 CrossRef CAS;
(d) J. Revell and H. Wennemers, Tetrahedron, 2007, 63, 8420–8424 CrossRef CAS PubMed;
(e) J. Duschmalé, J. Wiest, M. Wiesner and H. Wennemers, Chem. Sci., 2013, 4, 1312–1318 RSC.
-
(a) M. J. Mc Kennon and A. I. Meyers, J. Org. Chem., 1993, 58, 3568 CrossRef CAS;
(b) D. K. Mohapatra, P. K. Maity, R. G. Gonnade, M. S. Chorghade and M. K. Gurjar, Synlett, 2007, 12, 1893–1896 CrossRef.
-
(a) R. Rai, S. Aravinda, K. Kanagarajadurai, S. Raghothama, N. Shamala and P. Balaram, J. Am. Chem. Soc., 2006, 128, 7916–7926 CrossRef CAS PubMed;
(b) S. Hanessian and M. Angiolini, Chem.–Eur. J., 2002, 8, 111–117 CrossRef CAS.
-
(a) A. K. Sharma and R. B. Sunoj, J. Org. Chem., 2012, 77, 10516–10524 CrossRef CAS PubMed;
(b) M. P. Patil and R. B. Sunoj, Chem.–Eur. J., 2008, 14, 10472–10485 CrossRef CAS PubMed;
(c) D. Seebach and J. Golinski, Helv. Chim. Acta, 1981, 64, 1413–1423 CrossRef CAS;
(d) J. M. Betancort, K. Sakthivel, R. Thayumanavan, F. Tanaka and C. F. Barbas III, Synthesis, 2004, 1509–1521 CAS.
- Please see ESI.†.
-
(a) L. Zhang, X. Chen, P. Xue, H. H. Y. Sun, I. D. Williams, K. B. Sharpless, V. V. Fokin and G. Jia, J. Am. Chem. Soc., 2005, 127, 15998–15999 CrossRef CAS PubMed;
(b) B. C. Boren, S. Narayan, L. K. Rasmussen, L. Zhang, H. Zhao, Z. Lin, G. Jia and V. V. Fokin, J. Am. Chem. Soc., 2008, 130, 8923–8930 CrossRef CAS PubMed.
- F. Himo, T. Lovell, R. Hilgraf, V. V. Rostovtsev, L. Noodleman, K. B. Sharpless and V. V. Fokin, J. Am. Chem. Soc., 2005, 127, 210–216 CrossRef CAS PubMed.
-
(a) Previously Kokotos, et al. have reported similar transformation using pyrrolidine-thiohydantoins/thioxotetrahydropyrimidines with 2.5 mol% loading of catalyst, however additive (nitrobenzoic acid) 15 mol% was required for the reaction to proceed, C. G. Kokotos, D. Limnios, D. Triggidou, M. Trifonidou and G. Kokotos, Org. Biomol. Chem., 2011, 9, 3386 RSC;
(b) Reports of addition of nitroolefins to aldehydes are reported with lower loeading of catalysts, M. Wiesner, G. Upert, G. Angelici and H. Wennemers, J. Am. Chem. Soc., 2010, 132, 6–7 CrossRef CAS PubMed and references cited therein;
(c) A. Paul and H. Bittermann, Tetrahedron, 2006, 62, 8919–8927 CrossRef CAS PubMed;
(d) J. Eldo and J. P. Cardia, J. Med. Chem., 2006, 49, 5932–5938 CrossRef CAS PubMed;
(e) F. Giacalone, M. Gruttadauria, P. Agrigentoa and R. Notoa, Chem. Soc. Rev., 2012, 41, 2406–2447 RSC.
-
(a) S. R. Ban, X. X. Zhu, Z. P. Zhang, H. Y. Xie and Q. S. Li, Eur. J. Org. Chem., 2013, 2977–2980 CrossRef CAS;
(b) A. Lu, R. Wu, Y. Wang, Z. Zhou, G. Wu, J. Fang and C. Tang, Eur. J. Org. Chem., 2010, 2057–2061 CrossRef CAS;
(c) P. Li, L. Wang, Y. Zhang and G. Wang, Tetrahedron, 2008, 64, 7633–7638 CrossRef CAS PubMed;
(d) V. Maya and V. K. Singh, Org. Lett., 2007, 9, 1117–1119 CrossRef PubMed.
Footnote |
† Electronic supplementary information (ESI) available: Experimental procedures, characterization, NMR spectra of compounds, HPLC chromatograms, details of NMR and DFT studies. See DOI: 10.1039/c4ra04165h |
|
This journal is © The Royal Society of Chemistry 2014 |