DOI:
10.1039/C4RA04110K
(Paper)
RSC Adv., 2014,
4, 33923-33934
Solid-phase extraction, separation and preconcentration of titanium(IV) with SSG-V10 from some other toxic cations: a molecular interpretation supported by DFT†
Received
5th May 2014
, Accepted 24th June 2014
First published on 26th June 2014
Abstract
The present work reports the separation and preconcentration of titanium(IV) with functionalized silica gel (SSG-V10). A density functional theory (DFT) calculation has been performed to analyze the structure of both the extractor and the titanium(IV)-extractor complex to rationalize the sorption pathway. The systematic studies on the solid phase extraction of titanium(IV) ensured its quantitative sorption at solution pH: 5.0–6.0, influent volume: 1000 mL, analyte concentration: 23.95–35.92 μg mL−1, flow rate: 2.5 mL min−1, temperature: 27 °C, time of equilibration: 1.5 minutes and stress of foreign ions concentration (Cl−, SO42−, ClO4− and NO3−): 200 μg mL−1. The extractor, i.e., SSG-V10 (29
567.465 eV; η = 3.671 eV), has a high BET surface area (149.46 m2 g−1), a good value of exchange capacity (2.54 meq. of H+ g−1 of dry SSG-V10), break-through capacity (Q0 = 37.4–40.7 μg mg−1) and column efficiency (N: 108) with respect to titanium(IV). The +ve ΔH (0.048 kJ mol−1), ΔS (5 J K−1 mol−1) and −ve ΔG (−1.1488 kJ mol−1) indicate that the sorption process was endothermic, entropy-gaining and spontaneous in nature. The DFT calculations reveal that the guest, [(OH)(H2O)Ti(-μO)2(OH)(H2O)]+2 (15
531.185 eV; η = 3.39 eV), is stabilized as a syn isomer. This syn isomer was then placed at the exchange site and a second DFT calculation was performed. It was found that the hydrogen bonded anti complex gets stabilized by 0.286 eV over the syn isomer as the extracted species. The loading of titanium(IV) has been confirmed by EDX. The sorbed titanium(IV) was eluted as a distinct and detectable color with 1 M HCl containing H2O2. The preconcentration factor has been optimized at 60.8 ± 0.5. Titanium(IV) amid congeners and other metal ions, associated with it in ores and alloy samples, have been separated from synthetic mixtures. Moreover, the method was found effective for alloy samples.
1. Introduction
Titanium is a relatively rare element (0.6%), but because of its unusual corrosion resistance and self-healing properties, it is in great demand for special applications in turbine engines, aircrafts, marine equipment, and in medical applications like hip and knee replacements.1 The manufacture of the versatile white pigment TiO2 through the ‘chloride process’ generates large amounts of acidic waste chloride liquors,2 containing metal ions such as magnesium(II) (13.41 g dm−3), aluminum(III) (13.36 g dm−3), vanadium(V) (1.16 g dm−3), chromium(III) (0.78 g dm−3), manganese(II) (5.96 g dm−3), iron(II) (215.66 g dm−3) and iron(III) (48.67 g dm−3) and a comparatively low amount of titanium(IV) (0.22 g dm−3), which should be monitored to assess the efficiency of the titania production process. Thus, this chloride process waste poses a challenge to the analytical chemist. Sample clean up, through the preconcentration and separation of an analyte, is required before its monitoring with sophisticated instruments. During enrichment, the target species is selectively gathered in a small volume, from a large volume of sample of complex nature. Small amounts of analyte can therefore be quantified by coupling a preconcentration system to a sensitive, selective detection/estimation technique.3 The most widely used techniques for separation and pre-concentration of trace levels of titanium(IV) include liquid–liquid extraction,4 differential thermal analysis,5 reversed-phase high-performance liquid chromatographic separation,6 ion exchange,7 precipitation,8 and inductively coupled plasma optical emission spectrometry (ICPOES).9 Because of its unique features, ICPOES is able to work with trace level amounts of elements, but samples need severe digestion in 18 mol L−1 H2SO4 at 250 °C for 1 h in order to be analyzed. Moreover, prior to the operation a proper sample clean-up is required. Metal ion absorption on a solid polymer surface (solid phase extraction, SPE) is now considered as one of the most promising techniques for preconcentration, removal and recovery of metal ions from a wide variety of sources due to its eco-friendliness, fastness, simplicity and cost effectiveness. In our laboratory, Versatic-10 present on the surface of a hydrophobic silica support has been effectively used10–12 for the extraction, preconcentration and separation of Cu(II), Bi(III) and V(IV). However, the systematic extraction chromatographic investigation for preconcentration and separation of titanium(IV) using silica gel-Versatic-10 composite has not yet been reported. Versatic-10, a high molecular mass liquid cation-exchanger (HMMLCE), containing a mixture of C10-isomeric tertiary monocarboxylic acids, is soluble in several solvents like benzene, toluene, n-hexane, xylene, butanol, carbon tetrachloride, chloroform, nitrobenzene and diisopropyl ether.10,11 It has a good thermal and chemical stability and efficiently extracts metal ions from aqueous solution over a wide range of pH.12,13 The present work reports the separation and preconcentration of titanium(IV) with functionalized silica gel (SSG-V10) for the analysis. Global hardness (η) is a definite quantum mechanical descriptor and it is the cardinal index of chemical reactivity as well as stability of atoms and ions.14 Thus, a density functional theory (DFT) calculation has been performed not only to analyze the structure of the extractor and the titanium(IV)–extractor complex but also to rationalize the sorption pathway in terms of hardness.
2. Experimental
The pH measurements were carried out with a digital Elico L1-120 pH meter combined with a glass electrode. The scanning electronic microscopy (SEM) image was obtained at 5.0 kV by JEOL JSM-6700-FESEM. Fourier transform infrared (FT-IR) spectra of SSG-V10 in its titanium(IV) loaded and unloaded forms were recorded on a Shimadzu FT-IR spectrophotometer (Model no. FT-IR-8400S) using KBr pellets. EDX spectra were recorded on a JEOL 2010 FEG microscope equipped with an EDX analyzer. The X-ray diffraction patterns were recorded on a Rigaku (Ultima IV) H-12 Japan (Kurary Co. Ltd. N) diffractometer in the 2θ range of 18°–50° using Cu (Kα) as its scattering X-ray line source. UV-visible spectra for the metal–ligand complexation were studied with a Shimadzu UV-visible spectrophotometer (Model no. UV-1800). The thermal stability of the SSG-V10 has been determined by TGA/DTA analysis with PerkinElmer (STA 6000) Simultaneous Thermal Analyzer in temperature range from 40 to 950 °C. BET experiments were carried out by using a Beckman Coulter SA 3100 surface area analyzer at 77 K in high vacuum. A liquid cation exchanger, Versatic-10 (Shell Chemical, London, England), which is a high molecular mass synthetic C15–C16 monocarboxylic acid, was used without any further purification. Dimethyldichlorosilane (BDH, Bombay, India) was utilized as an end-capping reagent. A standard stock solution of titanium(IV) (5.5 mg mL−I) was prepared by dissolving Ti(SO4)2 (E. Merc, Bombay, India) in water and estimated spectrophotometrically.15 Buffer solutions of different pH values were prepared from acetic acid (0.2 M) and ammonium acetate (0.2) in proper ratios. For ion-exchange paper chromatography, 0.5 mL Versatic-10 was placed in 20 mL of diisopropyl ether solution and paper strips (Whatman no. 1) were immersed in it to achieve uniform coating, and then dried in air.10 The titanium(IV) solution (in μg) was taken on the paper strip containing Versatic10 and developed with acetate buffer (pH 5.5)–acetone (15
:
2 v/v) as the developing solvent (mobile phase).
2.1. Preparation of ion exchange material
Silica gel (60–120 mesh; 8.5 g) was rendered hydrophobic by exposing it to vapors of dimethyldichlorosilane (DMDCS) in N2 atmosphere. It was then washed with anhydrous methanol and dried at 100 °C. Versatic-10 (0.5 mL (density: 0.92 g mL−1) in 20 mL diisopropyl ether) was placed in an ultrasonic bath at 27 °C to achieve a homogenous solution. The silanized silica gel (SSG) was impregnated with Versatic-10 (SSG-V10; 5.411% w/w), diluted in diisopropyl ether and dried in a rotary vacuum evaporator to achieve uniform coating.11 Each column could be used for at least 60 cycles without any loss of its exchange capacity.
2.2. UV-visible spectral study
UV-visible spectra were recorded for the solutions (pH 5.5) containing titanium(IV) (10−4 M) and Versatic10 (10−4) at different molar ratios to obtain the composition of the extracted species.
2.3. Extraction procedure
An aliquot containing 5.5 μg mL−1 of titanium(IV) in acetate buffer was passed through the column at a flow rate of 2.0 mL−1 min at 25 °C. After extraction, titanium(IV) was stripped with 1 M HCl containing H2O2 and the amount of titanium(IV) from each fraction was determined spectrophotometrically.
2.4. Break-through capacity (BTC)
For the determination of break-through capacity (BTC), 200 mL fractions of metal ion solution (0.055 mg mL−1) were passed through the column and the effluent was collected till the amount of metal ion concentration was the same both in feed and effluent. A breakthrough curve (Fig. 1) was obtained by plotting the ratio
against the effluent volume, where C0 and Ce are the concentrations of the initial solution and of the effluent, respectively. BTC is calculated using the formula
, where C0 is the concentration of the metal ion in mg mL−1, V (10%) is the volume of the metal ion solution passed through the column when the exit amount reaches 10% of the initial amount (mg) and W is the weight of the ion-exchange material in grams.
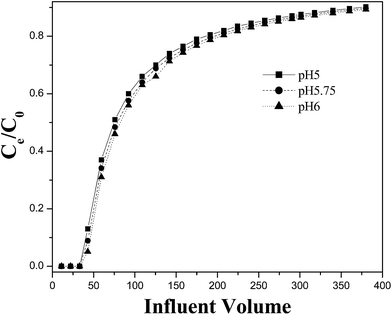 |
| Fig. 1 Break through curve (■ = pH 5; ● = pH 5.75 and ▲ = pH 6). | |
2.5. Computational methods
Standard density functional theory (DFT) calculations for all the structures were carried out with the Gaussian 09 package.16 The geometries of all the species were fully optimized by using B3LYP17,18 hybrid functional, LANL2DZ+ECP19–21 basis set for titanium (Ti) and 6-31g(d) basis set for the other elements. All the optimized structures have been characterized by frequency analysis as energy minima in the absence of imaginary frequencies. In addition, neutral bond order (NBO)22–24 calculations of the optimized structures were performed to determine the molecular orbital. The energies of the optimized structures were calculated after the ZPE (zero point energy) and thermal corrections.
3. Results and discussion
3.1 Silanization and the nature of the attachment of Versatic-10 leading to an ion-exchange material
The surface of the silica gel gets methylated (hydrophobic) during silanization (eqn (1)).25 The silanised silica gel (SSG) surface immobilizes the hydrophobic part of Versatic-10 through weak hydrophobic interaction (eqn (2)).10 The –COOH groups of Versatic-10 head towards the hydrophilic mobile phase and exist as dimers26 with the formation of cavities through intermolecular hydrogen bonding. |
 | (1) |
|
SSG + (RCOO−H+)2 → SSG·(RCOO−H+)2(s)
| (2) |
3.2 Physicochemical characteristics of the ion-exchange material
The SEM image of the ion-exchanger (SSG-V10) (Fig. 2) shows that the size of the particles lies between 60 and 70 μm and their shapes are irregular in nature. BET (Fig. 3) surface area (SA), pore volume and average pore diameter were found to be 149.46 m2 g−1, 0.2001 mL g−1 and 4.1 nm, respectively. The nitrogen BET often does not access the internal surface areas; hence, the standard methylene blue method11 is used.27 The SA is found to be much higher (280 m2 g−1) for the SSG-V10 composite. This SA obtained by methylene blue method is comparable to Versatic-911.3 The surface area of the material increases with decrease in the concentration of Versatic-10 in diisopropyl ether as solvent. This indicates that the increased concentration of Versatic-10 is blocking the pores of the silica gel. Pore diameters were computed using eqn (3) (ref. 11) as 62.5 μm for a pore volume of 0.1 mL. |
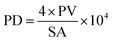 | (3) |
[PD = Pore diameter, PV = Pore volume, SA = Surface area]
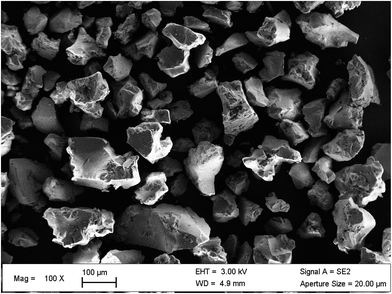 |
| Fig. 2 SEM image of SSG-V10. | |
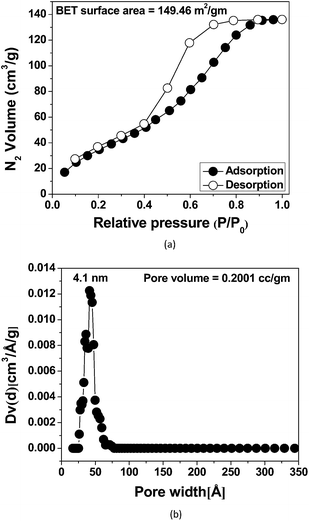 |
| Fig. 3 BET surface properties of SSG-V10 (a) BET surface area (149.46 m2 gm−1); (b) pore volume (0.2001 cm3 gm−1). | |
The BET study confirms that the adsorption hysteresis is H2 type, which is comprised of interconnectivity of pores in which the smaller pores act as a bottle-neck.28
3.3 TGA and DTA analyses
The TGA and DTA analyses (Fig. 4) exhibit two regions of weight loss. Up to 150 °C, the material (SSG-V10 composite) loses its trapped water and solvents (1.20%). The original weight reappears on restoring the material at room temperature. The second weight loss (5.404%) up to 850 °C, results entirely from the decomposition of Versatic-10 loaded on SSG (eqn (2)) for the preparation of the ion-exchanger. This is compatible with the TGA values (5.411% w/w); thus, the prepared ion-exchange material is thermally stable up to 150 °C.
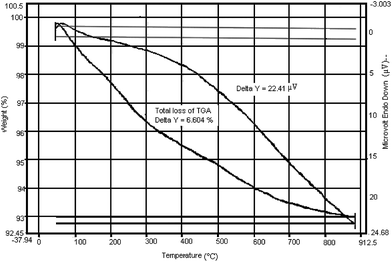 |
| Fig. 4 TGA-DTA of Versatic-10; flow rate of nitrogen: 20 mL min−1; temperature increment: 25 °C min−1. | |
3.4 FT-IR analysis
FT-IR spectra of the composite SSG-V10 containing –COOH groups29 showed a strong absorption band at 1688.4 cm−1. While at 2580 cm−1 a broad peak appears due to intermolecular hydrogen bonding (Fig. 5) by –COOH. Both these peaks indeed vanished, suggesting the conversion of the –COOH group to the –COO− ion and the rupture of the hydrogen bonding,29 thereby confirming the participation in the adsorption of titanium(IV). On the other hand, a sharp –OH peak at 3658 cm−1 appears as well as a broad peak at 3472 cm−1 in the loaded spectra due to intramolecular hydrogen bonding. The position of the peak at 1610 cm−1, related to the carboxylic –C
O group of SSG-V10, was found to be shifted to a somewhat higher frequency at 1616.2 cm−1 and confirmed the conversion of –COOH groups to –COO− ions with higher bond orders.29 EDX spectra of the loaded ion-exchange material also confirmed the presence of titanium(IV) in the sample (Fig. 6).
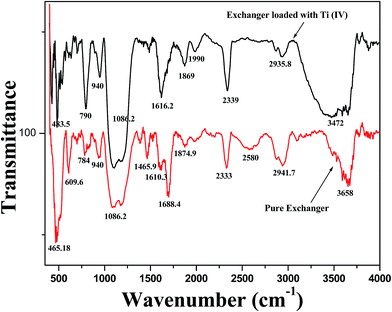 |
| Fig. 5 FT-IR spectra of (a) the ion exchanger and (b) Titanium(IV)-loaded ion exchanger. | |
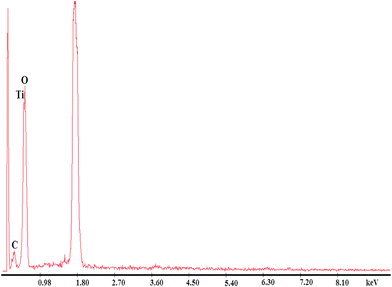 |
| Fig. 6 EDX spectra of Versatic-10 loaded with Titanium(IV). | |
3.5 Exchange capacity (EC), break-through capacity (maximum uptake capacity) and preconcentration factor
The exchange capacity of the prepared ion-exchange material (SSG-V10), in the range of pH 10–12, was determined (2.54 milliequivalent of H+/g at 25 °C), at different temperatures, by measuring the milliequivalent of sodium ions absorbed on 1 g of dry SSG-V10 in its H+ form.13 The ion-exchange material (1 g) becomes saturated at pH values of 5.0, 5.50 and 5.75 on equilibration with 680 mL (37.4 mg), 710 mL (39.1 mg) and 740 mL (40.7 mg) of titanium(IV) (0.055 mg mL−1) solution, respectively. However, the values (37.4–40.7 mg g−1) were much lower than the projected value of the maximum uptake capacity (1.27 mmol g−1 (60.8 mg g−1)) for dinuclear bipositive cationic species [(H2O)(OH)Ti(μ-O)2Ti(H2O)(OH)]2+ with respect to the exchange capacity (mono positive H+ ion). Here, the working pH (5.0–5.75) for the sorption of titanium(IV) is much lower than the required pH (10–12) for the full functioning of the exchange site containing the –COOH group. As a result of which the efficiency of the ion-exchange material stands at a somewhat reduced level of 61.5–66.9% with respect to titanium(IV). Exchangeable sites for titanium(IV) uptake increase along with an increase in the pH value.
3.6 Effect of pH on extraction
As carboxylic acid groups are the exchange sites on the investigated sorbent, the pH of the influent solution is an important controlling parameter in the sorption process.10 The systematic extraction chromatographic studies on titanium(IV) with SSG-V10, in the pH range 3.0 to 7.0, ensured its quantitative extraction in the optimum pH range 5.0–6.0 in 0.1 M acetate buffer. The retention of titanium increases with an increase in pH, reaches a maximum in the pH range 5.0–6.0, and then decreases with an increase in pH. Common anions like Cl−, SO42−, ClO4− and NO3− did not interfere. Quantitative retention of titanium was found up to a flow rate of 2.5 mL min−1.
At lower pH (<5.0), the otherwise difficult deprotonation of the carboxylic acid moiety of SSG-V10 (ref. 10) decreases the amount of –COO− ions and titanium extraction was poor. Moreover, the solubility of titanium(IV) increases in an aqueous solution along with the decrease in pH values. Both the effects lower the values of log
Kd (eqn S1 and Fig. S1; ESI† document), at low pH, and corroborate the suggested mechanistic path (eqn (4)).
The cationic dinuclear species, [(H2O)(OH)Ti(μ-O)2Ti(H2O)(OH)]2+, is present30 in the weak acidic pH range of 4.0–6.0. Its structure has been optimized by a DFT calculation (Fig. 7) and produced the syn compound (system energy: −15
531.124 eV; HOMO: −16.7623 eV; LUMO: −12.8711 eV and global hardness, η = 3.8912 eV). UV-visible spectra (Fig. 8) of the aqueous solutions containing metal–ligand (10−4 M) at different mole ratios were analyzed and the maximum absorbance at 1
:
1 mole ratio rationalizes the proper complex formation. A linear relationship (y = 1.029X + 1.6926; R2 = 0.9889) with the slope = 1.029 between log
Kd and log
C[Versatic-10] is also obtained in the range of pH 4.0–6.0 at a fixed concentrations of acetate and titanium(IV). Thus, the probable composition of the extracted species comes out to be 1
:
1 (metal–extractant). Within the pH range of 4.5–6.5, Versatic10 exists as a dimer through intermolecular hydrogen bonding.26 Bearing this in mind, it has been suggested that the dimer (anti compound), [(H2O)(OH)Ti(μ-O)2Ti(H2O)(OH)]+2, having the suitable size and charge, moves to the dimeric core of the exchange site and probably stabilized as anatase (pH: 2–11)31 with co-ordination number six, with one edge sharing (in this unit), at this recommended condition (pH: 4.0–6.0) as per the following proposed path:
|
SSG·(RCOO−H+)2(s) + [(H2O)(OH)Ti(μ-O)2Ti(H2O)(OH)]2+(aq.) ⇌ SSG·[Ti2(μ-O)2(OH)2(RCOO−)2](s) + 2H+(aq.)
| (4) |
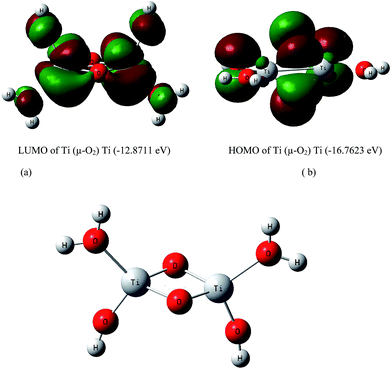 |
| Fig. 7 DFT-optimized structure of [(H2O)(OH)Ti(μ-O)2Ti(H2O)(OH)]+2 (η = 3.8912 eV); Ti–Ti: (bond distance) 265.99 pm; bond distance (Ti–O: 179.0 pm (bridge); Ti–O: 200.78 pm (terminal)); ∠Ti–O–Ti: 96.24°; ∠O–Ti–O: 106.4°; dipole moment: 4.2980 debye. | |
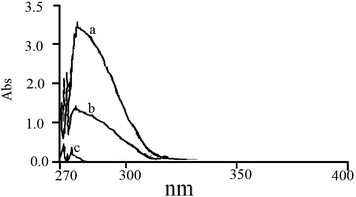 |
| Fig. 8 UV-visible spectra of organic phase (ligand–Titanium(IV) (a) 1 : 1 (b) 1 : 2 (c) 1 : 3). | |
Species such as Ti3O44+ appear at pH values higher than 6.5, and they lead to the eventual formation of colloidal or precipitated TiO2·nH2O (ref. 30) and do not participate in the exchange process.
3.7 Rationalization of titanium sorption in terms of DFT calculation
The COOH of Versatic-10, impregnated on SSG, which forms dimers and produces cavities (as is mentioned in Section 3.1) in the hydrophilic region of the ion-exchange material, was optimized by DFT calculations (Fig. 9). In the DFT optimized structure, the bond length of O⋯H⋯O is 244.9 pm, C–O bond length is 122.5 pm and ∠OCO is 124.02°.10 It generates a core radius of the dimer of 167.05 pm. The value is much lower than the calculated radius (from both the DFT optimized structure and global hardness (η) considering, r = 1/2η)32 of dinuclear anti compound of titanium(IV) (rDFT = 285.02 pm;
). To elucidate the hardness behavior, the hydrogen bonded dimeric structure of Versatic-10 (Fig. 9) was optimized by DFT calculations (−29
567.465 eV), and from the HOMO (−6.85732 eV)–LUMO (−1.714331 eV) gap, the global hardness was calculated32 (η = 5.143 eV). The dinuclear titanium(IV) complex is a soft acid (ηTi–syn–complex = 3.8912 eV) and has a suitable shape in comparison to the titanium(IV) ion itself (ηTi(IV) = 16.23 eV),33 which is trapped inside the co-ordination sphere with an anatase structure by one edge sharing at the recommended pH (2–11).31 Here, the stable dinuclear syn compound of titanium(IV), having comparable η values, is trapped inside the co-ordination sphere of a number of hard donor O-sites present in the ion-exchange core (inherent η = 5.143 eV) (Fig. 10). During the insertion of the larger-sized dinuclear syn compound of titanium(IV), the dimeric core of the ion-exchanger becomes ruptured, which is confirmed by the disappearance of the FT-IR peak at 2580 cm−1 in the titanium(IV)-loaded spectra (Fig. 5). Interestingly, this dinuclear syn isomer changed its conformation and stabilized as an anti-complex in the DFT optimized exchanger-metal complex entity. Lower steric crowding, comparable acid-base character (ηTi–Ti–complex = 3.8912 eV: ηV10–dimer = 5.143 eV)32 and intra-molecular hydrogen bonding (rO⋯H−O = 183.65 pm) rationalized the formation of the anti-complex (Fig. 10). The strong absorption band at 3658 cm−1 suffers a shift to 3472 cm−1 as a broad band in the loaded FT-IR spectrum (Fig. 5), thereby confirming the formation of intramolecular hydrogen bonding in the extracted species (Fig. 10). The plausible mechanistic path is depicted in Schemes 1 and 2.
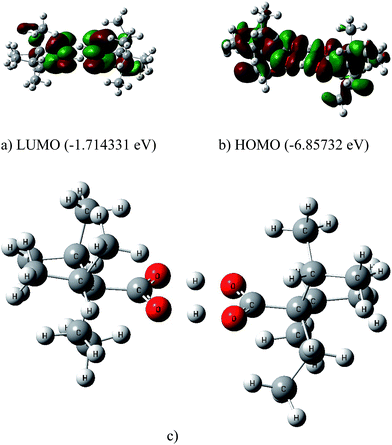 |
| Fig. 9 Hydrogen bonded dimer of Versatic-10 (ηdimer = 5.143 eV) (a) LUMO (b) HOMO and (c) optimized dimer Syn-Side-View Syn-Top-View. | |
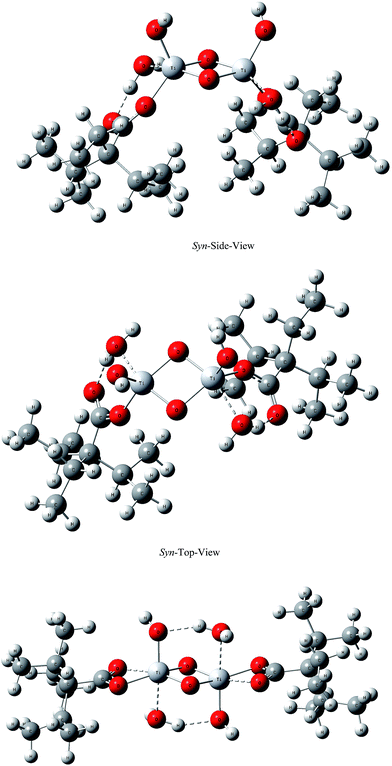 |
| Fig. 10 Ti-Versatic-10 anti-complex complex: bond distances (Ti–O: 179.0 pm (bridge); Ti–O: 260.05 pm (terminal); Ti–O: 213.61 pm (axial)); ∠Ti–O–Ti: 94.56°; ∠ –Ti–O: 63.02°. | |
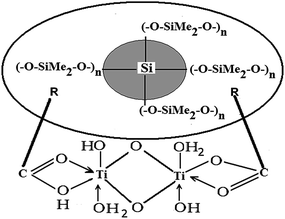 |
| Scheme 1 Probable attachment of titanium(IV) at the hydrophilic region of the ion-exchange material; R = –C{CH(CH3)2}2CH2CH3: silica gel (shaded area); methylated surface: (open area). | |
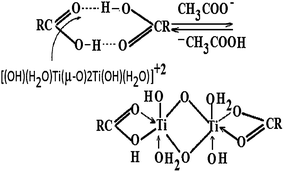 |
| Scheme 2 Probable mechanism of titanium(IV) trapping in dimeric cavity of the ion-exchange material; R = –C{CH(CH3)2}2CH2CH3. | |
The core diameter (Fig. 10) (i.e., the distance between two carboxylic carbon atoms) is 746.2 pm and in 1 g of dry SSG-V10 having the BET surface area of 149.46 m2 g−1 generates 3.42 × 1020 such cores. In each core, titanium is present as a dinuclear complex [(H2O)(OH)Ti(μ-O)2Ti(H2O)(OH)]+2 and it corresponds to 1135.64 μM (54.36 mg) of titanium(IV) g−1 of dry SSG-V10. The experimental value (37.4–40.7 mg g−1) is effectively 69–75% of this theoretically calculated value.
3.8 XRD analysis
The XRD patterns of silanized silica gel (SSG), SSG-V10 composite and SSG-V10-titanium(IV) are shown in Fig. 11. Each of them has two reflections: a broad, strong reflection (2θ = 21.83°; 2θ = 22.16°; 2θ = 21.82°) and a weak and sharp reflection (2θ = 48.56°; 2θ = 48.55°; 2θ = 48.52°) and the corresponding reflections of SSG, SSG-V10 and SSG-V10-titanium(IV) were found to be almost in the same position in their XRD patterns. The SSG network does not get influenced by impregnation with Vesatic-10 or by the sorption of titanium(IV) on the surface of the SSG-Versatic-10 composite. Here, the binding between SSG and Versatic-10 is caused by a hydrophobic interaction only (Scheme 1), and it takes place at the surface of the hydrophobic SSG. After that, the metal ion, titanium(IV), is placed at the exchange site of Versatic-10.
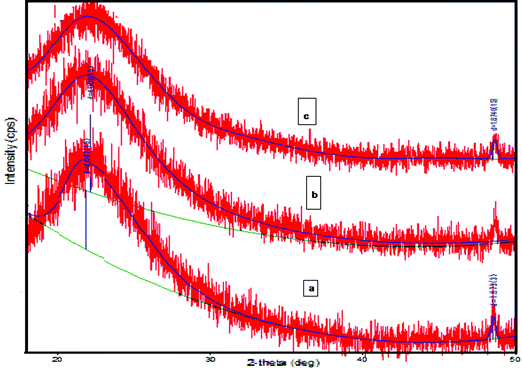 |
| Fig. 11 XRD pattern of (a) SSG (b) SSG V10 and (c) SSG V10 Titanium(IV). | |
3.9 Selection of stripping agents
At low pH, TiO2+ is in equilibrium with [Ti(OH)2]2+ and [Ti(aq.)]4+. Thus, in the elution process (back extraction) extracted titanium elutes as TiO2+, which is in equilibrium with [Ti(OH)2]2+ and [Ti(aq.)]4+ in such strong acid media, and H+ acts as an eluent. After extraction, titanium(IV) was stripped from the column using various acids of different concentrations (Fig. S2†). Quantitative elution of titanium(IV) has been achieved with HNO3 (0.5–1 M), HCl + H2O2 (0.5–1 M) and H2SO4 (1–1.5 M) (Table S1†). Acetic acid weakly dissociates in aqueous solution; thus, it performs poorly in the recovery of titanium from the extractant compared to the strong inorganic acids like HCl, H2SO4 or HNO3. Moreover, the strongly basic Ti
O group is very much susceptible to an electrophilic attack by protons and titanium(IV) leaves the cavity of the ion-exchange core (Scheme 2) through the formation of large polymeric species like [Ti
O → Ti–O–Ti ← O
Ti]n or [–O–Ti–O–Ti–OH]n (ref. 30) in mineral acids. Column efficiency (N) for these eluents has been calculated10 with respect to titanium(IV) (NH2SO4 = 88; NHNO3 = 60; NHCl+H2O2 = 108) (eqn (5)). It was found that 1 M HCl containing H2O2 as an eluent gave a symmetric elution profile (Fig. S2†). It requires less volume (15 mL) and provides the highest column efficiency. Moreover, 1 M HCl along with H2O2 gave a distinct and detectable bright yellow color with titanium(IV)15 during its elution. This colored complex has been utilized for the spectrophotometric estimation of titanium(IV). These features justify the use of 1 M HCl containing H2O2 as a stripping agent for the further steps of experiments. |
 | (5) |
where y = distance for peak elution,
and W0.6065 = width of the peak at 0.6065 times the peak height.
3.10 Optimization of column flow rate and time
Systematic studies on flow rate show that with the increase of flow rate (mL min−1) the retention of titanium(IV) decreases linearly. This was quantitative (<96%) up to the flow rate of 4.0 mL min−1. Since the reaction kinetic was found to be very fast, the effect of equilibration was investigated at a high flow rate (6–8 mL min−1), and it was observed that 1.5 min was sufficient for its extraction from aqueous solution at pH 5.0–5.75.
3.11 Effect of pH, volume and concentration of influent on recovery and preconcentration factor (PF)
During the extraction of titanium(IV) (23.95–35.92 μg mL−1) in the range of pH 5.0–5.75, it was found that an influent volume up to 1000 mL, PF (ratio of effluent concentration, Cf to influent concentration, Cs) increased with the increase in effluent concentration (Table 1). Although at a particular volume, PF was not depending on either the concentration or the pH of the influent. Recovery decreased with the increase in influent volume, and for an influent volume up to 1000 mL recoveries were quantitative (>90%). To attain the break-through values (as 37
400 μg g−1 at pH 5.0, 39
100 μg g−1 at pH 5.5 and 40
700 μg g−1 at pH 5.75), respectively, 1561, 1306 and 1133 mL of titanium(IV) solution were passed through the column. However, due to the volume effect10 recoveries were much poorer (<80%); consequently, PF did not attend its maximum possible values (104.1, 87.1 and 75.5 for 100% recovery). It was optimized at a value of 60.8 ± 0.5. Plot of VS (sample volume) versus RTi(IV) (recovery of titanium(IV)) produced two linear segments (y = −0.0094x + 99.4; R2 = 0.963 and y = −0.0637x + 153.8; R2 = 0.999) intersecting at a volume of 890 mL (Fig. S2†). The slopes of the corresponding segments were the measures of desorption efficiency and yielded the desorption constants8 Kdesorption1(9.4 × 10−3) and Kdesorption2(6.37 × 10−2), respectively. For influent volumes higher than 1000 mL, the rate of desorption was much higher, and the recovery would become zero at an influent volume of 1628.5 mL (extrapolated value). The cationic components of the solvents and the metal ions took part in the sorption and desorption process by replacing each other during the movement of the mobile phase. With the increase in the volume of the solvent, the extent of desorption of the metal ion becomes predominant and it becomes complete at a volume of 1628.5 mL. This indicates that at this very condition the influent solvent itself will act as an eluent.
Table 1 Effect of volume, sample concentration and pH on the preconcentration factor. [Flow rate: 3.5 mL min −1; pH: 5.0–6.0; Titanium(IV): 23.95–35.92 μg mL−1; # average of three determinations; standard deviation <0.04]
pH |
Sample conc. (Cs) (μg mL−1) |
Sample volume, VS (mL) |
Amount of Ti(IV) (μg) |
Effluent conc. (Cf) (μg mL−1) |
Recoverya (%) |
PF (Cf/Cs) |
Added |
Recovered |
Average of five determinations; PF = ratio of effluent conc. to sample conc. (Cf/Cs). |
5.0 |
23.95 |
200 |
4790 |
4732.5 |
315.5 |
98.8 |
13.2 |
400 |
9580 |
9311.8 |
620.8 |
97.2 |
25.9 |
800 |
19 160 |
18 393.6 |
1226.2 |
96.0 |
51.2 |
1000 |
23 950 |
21 674.7 |
1445.0 |
90.5 |
60.3 |
1300 |
31 135 |
21 888.0 |
1459.2 |
70.3 |
60.9 |
1561 |
37 386 |
20 487.5 |
1365.8 |
54.8 |
57.0 |
5.5 |
29.93 |
200 |
5986 |
5938.1 |
395.9 |
99.2 |
13.2 |
400 |
11 972 |
11 684.6 |
778.9 |
97.6 |
26.0 |
800 |
23 944 |
23 201.7 |
1546.8 |
96.9 |
51.7 |
1000 |
29 930 |
21 595.5 |
1839.7 |
92.2 |
61.4 |
1200 |
35 916 |
27 511.7 |
1834.1 |
76.6 |
61.2 |
1306 |
39 088 |
27 010.0 |
1800.7 |
69.1 |
60.2 |
5.75 |
35.92 |
200 |
7184 |
7083.4 |
472.2 |
98.6 |
13.1 |
400 |
14 368 |
14 080.6 |
938.7 |
98.0 |
26.1 |
800 |
28 736 |
27 988.8 |
1865.9 |
97.4 |
51.9 |
1000 |
35 920 |
32 759.0 |
2183.9 |
91.2 |
60.8 |
1100 |
39 512 |
32 874.0 |
2191.6 |
83.2 |
61.0 |
1133 |
40 698 |
23 884.0 |
2192.2 |
80.8 |
61.0 |
3.12 Effect of temperature on extraction
The extraction equilibrium constant (Kex) has been computed at different temperatures using the following equation (eqn (6))10 and plot of log
Kex vs. 1000/T gives a linear relationship (y = −2.523x + 8.66; R2 = 0.996) (Fig. S4†). It was observed that the separation efficiency, C/C0 (Fig. S5†) increased with sharply increasing temperature in the temperature range of 293–310 K. The equilibration time decreased with increasing temperature, in the mentioned interval and became nearly constant at the final equilibrium state. |
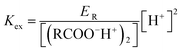 | (6) |
where [(RCOO−H+)2(s)] denotes the concentration of SSG-V10 in its dimeric form, which participated in the ion-exchange process, and ER is the ratio of extracted metal ion to its un-extracted portion.
The enthalpy change (ΔH) was evaluated from the plot of log
Kex versus 1000/T (eqn (7)). The effect of temperature on the extraction of titanium(IV), in the range of pH 5.5–5.75, has been performed for the determination34 of different thermodynamic parameters (ΔG and ΔS at 300 K) using the standard van't Hoff equation (eqn (8) and (9)).
|
ΔH = −(slope) × 2.303 × R
| (7) |
|
ΔG = −2.303RT log Kex
| (8) |
|
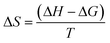 | (9) |
where
R (8.314 J K
−1 mol
−1) is the gas constant.
The positive ΔH (0.048 kJ mol−1) and smaller ΔS (5 J K−1 mol−1) rationalize the endothermic nature of the extraction process. During the adsorption of titanium(IV), H+ is released in the solution phase and increases the number of neutral CH3COOH. This effect correlates with the positive value of ΔS. The higher negative value of ΔG (−1.1488 kJ mol−1) suggests the spontaneity and tendency for chemisorptions of the equilibrium,34 and this is in agreement with the Langmuir isotherm.
3.13 Ion-exchange paper chromatography
At pH 5.75, the Rf values and selectivity factors (α) (ratio of the Rf values) of metal ions were determined in an acetate buffer–acetone mixture (15
:
2 v/v) using chromatograms (Table S2†). The results show that, under this recommended condition, Sn(II), Cr(III), Ca(II), Mg(II), Co(II), Zn(II), Cd(II) are very weakly bound (Rf value ∼0.9), metal ions like titanium(IV), Pb(II), Al(III), Cu(II), Ga(III) are moderately bound (Rf value 0.3–0.6) while Hg(II), Bi(III), Tl(III) is tightly bound (Rf value < 0.2) with the stationary phase. At pH 2.5, binding strength of Zr(IV) (Rf value = 0.06) is much higher than that of Fe(III) (Rf value = 0.41) and under this condition, large polymeric species like [Ti
O–Ti–O–Ti–O
Ti]n do not participate in the exchange process (Rf value = 0.93). Selectivity factors for most of the studied metal ions were appreciably higher (α ≥ 2.17), and this suggests their easy separation from titanium(IV).
3.14 Effect of pH on selectivity factors (α)
Systematic studies on Rf values of metal ions (except Fe(III) and Zr(IV)) in the range of pH 5.0–5.75 have been performed. The selectivity factors (α) (separation efficiency) with respect to titanium(IV) have been computed, and they were appreciably higher (≥2.17) at pH 5.75 (Table 2). The values of separation factors for Fe(III) and Zr(IV) were found to be the best at pH 2.5.
Table 2 Effect of pH on Rf values and selectivity factors (α = (Rf)i/(Rf)Ti(IV)) of different metal ions obtained on Whatman no. 1 impregnated with Versatic-10; [time = 2.5 hours; developing solvent = acetate buffer: acetone (25
:
1) v/v]
pH |
Metal ion |
Rf values |
Selectivity factors (α) |
5.0 |
Zn(II) |
0.82 |
10.25 |
Cd(II) |
0.86 |
10.75 |
V(IV) |
0.38 |
4.75 |
U(VI) |
0.09 |
1.13 |
Ti(IV) |
0.08 |
1.00 |
Cr(III) |
0.96 |
2.34 |
Th(IV) |
0.05 |
1.20 |
5.75 |
Zn(II) |
0.88 |
14.66 |
Cd(II) |
0.84 |
14.00 |
V(IV) |
0.34 |
5.67 |
U(VI) |
0.14 |
2.33 |
Ti(IV) |
0.06 |
1.00 |
Cr(III) |
0.86 |
14.33 |
Th(IV) |
0.02 |
3.00 |
3.15 Separation of titanium(IV) from binary and multicomponent mixtures
In order to access the possible analytical applications, different binary and multicomponent mixtures (Table 3; Table 4) of titanium(IV) containing diverse metal ions (elements present in the acidic waste of “chloride process for titania production”: Ti(IV), Fe(III), Cr(III), V(V), Al(III); elements of Gr III in analytical table: Ti(IV), Zn(II), Ce(IV), Th(IV), U(VI); elements of periodic table: Ti(IV) and Zr(IV)) having widely different Rf values (appreciably higher (α ≥ 1.5))11 have been formed. Then, from these important synthetic mixtures, viz., mixture number 4 containing Zr(IV), V(IV), Al(III), Ti(IV); mixture number 1 containing Fe(III), Cr(III), Ti(IV) etc. (Table 4) metal ions from one to another have effectively been separated. The binary and ternary separations from diverse metal ions were achieved either by exploiting the differences in the pH for extraction or by using suitable eluting agent utilizing the selectivity factors (α ≥ 1.5)9 (Tables 3 and 4) (separation procedure is given as ESI file (f. 1)†). After recovery, except Ti(IV) the different metal ions (diverse ions) which have been selectively separated from the synthetic mixtures (Table 3 and Table 4) were determined complexometrically.9 In almost all the cases, fairly good reproducibility with respect to quantitative extraction, separation and recovery have been achieved.
Table 3 Separation of Titanium(IV) from synthetic metal ion mixtures [Titanium(IV) taken: 2.2 mg mL−1, column: 0.8 × 8 cm, flow rate: 1 mL min−1, pH-2.5*, 5.0; temp: 25 °C; RSD = Relative Standard Deviation]
Mixture |
Cations |
Added (mg mL−1) |
Recovered (mg mL−1) |
R.S.D. (%) |
Eluent vol. (mL) |
1 |
*Fe(III) |
2.85 |
2.87 |
1.74 |
0.1 M HCl (30) |
Titanium(IV) |
2.20 |
2.22 |
3.73 |
1 M HCl + H2O2 (15) |
2 |
Th(IV) |
1.8 |
1.88 |
3.80 |
2.5 M HNO3 (25) |
Titanium(IV) |
2.20 |
2.16 |
1.53 |
1 M HCl |
3 |
Pb(II) |
2.20 |
2.24 |
2.41 |
0.005 M CH3COOH (60) |
Titanium(IV) |
2.15 |
2.17 |
3.22 |
1 M HCl + H2O2 (15) |
4 |
Ni(II) |
2.15 |
2.12 |
1.50 |
Mobile phase |
Titanium(IV) |
2.20 |
2.24 |
2.41 |
1 M HCl + H2O2 (15) |
5 |
Zn(II) |
2.63 |
2.62 |
2.17 |
Mobile phase |
Titanium(IV) |
2.20 |
2.22 |
3.73 |
1 M HCl + H2O2 (15) |
6 |
*Zr(IV) |
2.08 |
2.06 |
2.12 |
4 M HNO3 (20) |
Titanium(IV) |
2.20 |
2.21 |
3.48 |
1 M HCl + H2O2 (15) |
7 |
Cd(II) |
1.80 |
1.83 |
3.87 |
Mobile phase |
Titanium(IV) |
2.20 |
2.22 |
3.73 |
1 M HCl + H2O2 (15) |
Table 4 Separation of Titanium(IV) from synthetic metal ion mixtures [Titanium(IV) taken: 2.2 mg mL−1, column: 0.8 × 8 cm, flow rate: 1 mL min−1, pH: 2.5*, 5.0; temp: 25 °C; RSD = Relative Standard Deviation]
Mixture |
Cations |
Added (mg mL−1) |
Recovered (mg mL−1) |
R.S.D. (%) |
Eluent vol. (mL) |
1 |
*Fe(III) |
2.85 |
2.87 |
1.74 |
0.1 M H2SO4 (20) |
Cr(III) |
2.15 |
2.12 |
1.50 |
Mobile phase (25) |
Titanium(IV) |
2.20 |
2.24 |
2.41 |
1 M HCl + H2O2 (15) |
2 |
U(VI) |
2.90 |
2.98 |
2.21 |
0.25 M HNO3 (30) |
V(IV) |
2.20 |
2.14 |
3.48 |
0.1 M CH3COOH (50) |
Titanium(IV) |
2.20 |
2.26 |
3.60 |
1 M HCl + H2O2 (15) |
3 |
*Zr(IV) |
2.98 |
3.04 |
2.45 |
4 M HNO3 (20) |
Cr(III) |
2.15 |
2.20 |
1.66 |
Mobile phase (25) |
Titanium(IV) |
2.20 |
2.18 |
2.13 |
1 M HCl + H2O2 (15) |
4 |
*Zr(IV) |
2.98 |
3.02 |
1.68 |
4 M HNO3 (20) |
V(IV) |
2.20 |
2.15 |
1.68 |
0.1 M HNO3 (05) |
Titanium(IV) |
2.20 |
2.26 |
2.54 |
1 M HCl + H2O2 (15) |
5 |
*Zr(IV) |
2.98 |
3.08 |
3.12 |
4 M HNO3 (20) |
U(VI) |
2.90 |
2.96 |
1.98 |
0.25 M HNO3 (30) |
Titanium(IV) |
2.20 |
2.12 |
2.78 |
1 M HCl + H2O2 (15) |
6 |
*Zr(IV) |
2.98 |
3.08 |
3.08 |
4 M HNO3 (20) |
V(IV) |
2.20 |
2.13 |
3.11 |
0.1 M HNO3 (05) |
Al(III) |
1.80 |
2.00 |
2.69 |
1 M CH3COOH (20) |
Titanium(IV) |
2.20 |
2.12 |
3.22 |
1 M HCl + H2O2 (15) |
3.16 Application of the method of extraction in an alloy sample of titanium
A titanium, Ti6Al–4V alloy (V, Al, Ti and Fe) sample was treated15 with 10 mL 1
:
1 nitric acid and 10 mL of 70% perchloric acid. The analyte solution (80 mL) was evaporated to dryness. The mass was dissolved in 25 mL distilled water. Any residue that remained was filtered (Whatman no. 42) and diluted to 100 mL with distilled water and extracted at pH 2.5, whereas Fe(III) was extracted and eluted with 0.5 M H2SO4. The effluent containing V, Al and Ti was passed through the column at pH 5.75 and sequentially eluted by the developed method (Table 4), and the recovery was quantitative (Table 5).
Table 5 Separation of Titanium(IV) from real sample
Sample |
Ti6Al–4V |
Metal ion |
V(IV) |
Al(III) |
Titanium(IV) |
Recovered |
3.79 |
7.26 |
86.18 |
Certified |
4.50 |
6.75 |
88.42 |
3.17 Estimation of an unknown concentration
For an unknown solution (200 mL), the recovered amount was estimated in the effluent. The recovered amounts of titanium(IV) were equated with the average recovery of 98.8%, for a sample volume of 200 mL the concentration for 100% recovery was calculated. The predicted concentration agreed well with observed values obtained by AAS and spectrophotometry (relative error <7.02%) (Table 6). The preconcentration process gave slightly lower recovery with respect to 98.8%, probably because of the loss of analyte in both the extraction and elution steps, during its recovery, and all the recovery values were found to be about 4.0% ± 0.5% lower than AAS.
Table 6 Comparison of the estimated conc. (AAS and Spectrophotometry) with the preconcentration technique, in the concentration range of 20.4–40.8 μg mL−1; sample volume: 200 mLa
Amounts of Titanium(IV) (μg) |
Standard |
Estimated AAS |
Spectrophotometry |
Predicted (preconcentration technique) |
Values in the parentheses represent the relative errors (%). |
4080 |
4026 (1.32) |
3929 (3.70) |
3848 (5.69) |
6120 |
5982 (2.25) |
5892 (3.73) |
5726 (6.44) |
8160 |
7948 (2.60) |
7822 (4.14) |
7668 (6.03) |
The separation of titanium(IV) and Cr(III) from water (pond and well water) samples was performed by applying the proposed method (PF: 59.1 ± 0.3) (Table S3†). The amounts of recovered titanium(IV) were equated with the average recovery of 95% for a sample volume of 800 mL−1, and the projected concentrations were calculated to get an idea about the concentrations of the mother samples. The calculated values were highly compatible with the observed values obtained by spectrophotometry (relative error <2.8%) (Table S4†).
4. Conclusions
According to the DFT optimized structure, the dimer complex, [(OH)(H2O)Ti(-μO)2(OH)(H2O)]+2, exists as a syn isomer, but in the extracted species it is the anti-form, which is stabilized by 0.286 eV over the syn isomer due to intra-molecular hydrogen bonding. DFT-optimized extracted complex turned out to be anatase. The loading of titanium(IV) on to SSG-V10 has been confirmed by EDX. The ion exchange material, SSG-V10 has a high BET surface area of 149.46 m2 g−1. The positive values of ΔH (0.048 kJ mol−1) and ΔS (5 J K−1 mol−1) and negative value of ΔG (−1.48 kJ mol−1) indicated that the interaction process is endothermic, entropy-gaining and spontaneous in nature. A clean separation of titanium(IV) has been achieved from several toxic and heavy metal ions like Pb(II), Fe(III), Cr(III), Zn(II), Cd(II), Hg(II), Tl(III), Zr(IV), Ce(IV), and Th(IV) having very high selectivity factor (α ≥ 1.24) values. Very minute amounts (∼0.2 mL) of liquid extractant in the developed ion-exchange material can selectively separate titanium(IV) from wide varieties of multicomponent mixtures containing cations (Table 4), present in the acidic waste of “chloride process for titania production” (Ti(IV), Fe(III), Cr(III), V(V), Al(III)) and also from Gr III in the analytical table (Ti(IV), Zn(II), Ce(IV), Th(IV), U(VI)). Regarding the estimation of an unknown sample of titanium(IV) in its microgram level, the method was highly compatible with other sophisticated techniques. Estimation of the components of a mixture in the conventional spectrophotometric method fails if the absorption of the metal ions in presence of ligands occurs in the overlapping region. However, the present method does not face any such inconveniences as interfering ions are already separated. The ion-exchange material developed is chemically stable (up to 6 M HNO3, 4 M CH3COOH, 4 M HCl and 2 M H2SO4) and effective over a wide range of pH. It can be used for more than 30–40 cycles without any loss in its exchange capacity.
Acknowledgements
One of the authors (M. K. Barman) gratefully acknowledges the facilities provided by the Department of Chemistry, Visva-Bharati, Santiniketan. The authors thankfully acknowledge experimental assistance from Laboratories CRISMAT, UMR 6508, CNRS/ENSICAEN, Caen Cedex, France and are also grateful to the Department of Physics, Visva-Bharati for providing instrumental support (XRD).
References
- F. A. Cotton, G. Wilkinson, C. A. Murillo and M. Bochmann, Adv. Inorg. Chem., John Wiley and Sons, 5th edn, 1998, pp. 695–696 Search PubMed.
- P. R. Narayanan and M. R. Lakshmipathy, Solvent extraction separation of titanium(IV), vanadium(V) and iron(III) from simulated waste chloride liquors of titanium minerals processing industry by the trialkylphosphine oxide Cyanex 923, J. Chem. Technol. Biotechnol., 2004, 79, 734–741 CrossRef PubMed.
- B. Mandal and N. Ghosh, Extraction chromatographic method of preconcentration and separation of lead(II) with high molecular mass liquid cation exchanger, Desalination, 2010, 250, 506–514 CrossRef CAS PubMed.
- N. M. Sundaramurthi and V. M. Shinde, Solvent extraction of titanium(IV), zirconium(IV) and hafnium(IV) salicylates using liquid ion exchangers, Analyst, 1989, 114, 201–205 RSC.
- W. R. Bandi and G. Krapf, Identification and determination of titanium sulphide and carbosulphide compounds in steel, Analyst, 1979, 104, 812–821 RSC.
- S. Oszwałdowski, Reversed-phase high-performance liquid chromatographic separation and determination of titanium, vanadium, niobium and tantalum ternary complexes with hydrogen peroxide and 2-(5-bromo-2-pyridylazo)-5-diethylaminophenol, Analyst, 1995, 120, 1751–1758 RSC.
- K. Sobhana Menon and Y. K. Agrawal, Micro-determination and separation of titanium in environmental samples by spectrophotometry using a liquid ion exchanger, Analyst, 1984, 109, 27–30 RSC.
- L. G. Gerasimova and M. V. Maslova, Precipitation of Titanium(IV) and Iron(III) Phosphates from Sulfuric Acid Solutions Russ, J. Appl. Chem., 2003, 76(11), 1855–1857 CAS.
- M. C. E. Lomer, R. P. H. Thompson, J. Commisso, C. L. Keen and J. J. Powell, Determination of titanium dioxide in foods using inductively coupled plasma optical emission spectrometry, Analyst, 2000, 125, 2339–2343 RSC.
- B. Mandal, U. S. Roy, D. Datta and N. Ghosh, Combined cation-exchange and extraction chromatographic method of pre-concentration and concomitant separation of Cu(II) with high molecular mass liquid cation exchanger after its online detection, J. Chromatogr. A, 2011, 1218, 5644–5652 CrossRef CAS PubMed.
- B. Mandal and N. Ghosh, Extraction chromatographic method of preconcentration and separation of lead(II) with high molecular mass liquid cation exchange, J. Hazard. Mater., 2010, 182, 363–370 CrossRef CAS PubMed.
- B. Mandal, M. K. Barman and B. Srivastava, Extraction Chromatographic Method of Preconcentration, Estimation and Concomitant Separation of Vanadium(IV) with Silica Gel-Versatic-10 Composite, J. Chromatogr. Sci., 2013 DOI:10.1093/chromsci/bmt160 , in press.
- B. Mandal and N. Ghosh, Extraction chromatographic method of preconcentration and separation of cadmium(II) with high molecular mass liquid cation exchanger, Versatic-10, Indian J. Chem., Sect. A:, 2009, 48, 668–671 Search PubMed.
- R. G. Parr and W. Yang, Density-functional Theory of Atoms and molecules, Oxford University Press, New York, 1989 Search PubMed.
- E. B. Sandall, Colorimetric Determination of Traces of Metals, Interscience Publications INC., New York, 1965, pp. 870–880 Search PubMed.
- M. Caricato, A. Frisch and M. J. Frisch, Gaussian 09, Revision D.01, Gaussian Inc., 340 Quinnipiac St., Bldg. 40 Wallingford, CT 06492, USA, 2009 Search PubMed.
- A. D. Becke, Density-functional thermochemistry. III. The role of exact exchange, J. Chem. Phys., 1993, 98, 5648–5652 CrossRef CAS PubMed.
- C. Lee, W. Yang and R. G. Parr, Development of the Colle-Salvetti correlation-energy formula into a functional of the electron density, Phys. Rev. B:, 1988, 37, 785–789 CrossRef CAS.
- P. J. Hay and W. R. Wadt, Ab initio effective core potentials for molecular calculations. Potentials for the transition metal atoms Sc to Hg, J. Chem. Phys., 1985, 82, 270–283 CrossRef CAS PubMed.
- W. R. Wadt and P. J. Hay, Ab initio effective core potentials for molecular calculations. Potentials for main group elements Na to Bi, J. Chem. Phys., 1985, 82, 284–297 CrossRef CAS PubMed.
- P. J. Hay and W. R. Wadt, Ab initio effective core potentials for molecular calculations. Potentials for K to Au including the outermost core orbitals, J. Chem. Phys., 1985, 82, 299–310 CrossRef CAS PubMed.
- J. E. Carpenter and F. Weinhold, Analysis of the geometry of the hydroxymethyl radical by the “different hybrids for different spins” natural bond orbital procedure, J.
Mol. Struct.: (THEOCHEM), 1988, 169, 41–62 CrossRef.
- A. E. Reed, L. A. Curtiss and F. Weinhold, Intermolecular Interactions from a Natural Bond Orbital, Donor-Acceptor Viewpoint, Chem. Rev., 1988, 88, 899–926 CrossRef CAS.
- J. P. Foster and F. Weinhold, Natural Hybrid Orbitals, J. Am. Chem. Soc., 1980, 102, 7211–7218 CrossRef CAS.
- P. Chowdhury, S. K. Pandit and B. Mandal, Preparation and characterization of silanised silica gel-supported poly(acrylic acid) network polymer and study of its analytical application as selective extractor for lead ion, J. Appl. Poly. Sci., 2008, 110, 475–480 CrossRef CAS PubMed.
- T. Shigematsu, S. Nishimura, T. Tanabe and Y. Kondo, Solvent extraction of europium by versatic acid 911, Nippon Kinzoku Gakkaishi, 1972, 36, 5445–5448 Search PubMed.
- Y. Yukselen and A. Kaya, Suitability of the methylene blue test for surface area, cation exchange capacity and swell potential determination of clayey soils, Eng. Geol., 2008, 102, 38–45 CrossRef PubMed.
- G. Manson, The effect of pore space connectivity on hysteresis of capillary condensation in adsorption – desorption isotherms, J. Colloid Interface Sci., 1982, 88(1), 36–46 CrossRef.
- K. Nakamoto, Infrared and Raman Spectra of Inorganic and Coordination Compounds, Part B, John Wiley & Sons, Inc., 5th edn, 1977, pp. 59–61 Search PubMed.
- F. A. Cotton, G. Wilkinson, C. A. Murillo and M. Bochmann, Adv. Inorg. Chem., John Wiley and Sons, 5th edn, 1998, vol. 698–699, pp. 718–721 Search PubMed.
- M. P. Finnegan, H. Zhang and J. F. Banfield, Anatase Coarsening Kinetics under Hydrothermal Conditions As a Function of pH and Temperature, Chem. Mater., 2008, 20, 3443–3449 CrossRef CAS.
- R. G. Pearson, Absolute electronegativity and hardness: application to inorganic chemistry, Inorg. Chem., 1988, 27, 734–740 CrossRef CAS.
- D. C. Ghosh and R. Biswas, Theoretical Calculation of Absolute Radii of Atoms and Ions. Part 2. The Ionic Radi, Int. J. Mol. Sci., 2003, 4, 379–407 CrossRef CAS PubMed.
- P. Atkins and J. Paula, Physical Chemistry, Oxford University press, 8th edn, 2006, pp. 77–117 Search PubMed.
Footnote |
† Electronic supplementary information (ESI) available. See DOI: 10.1039/c4ra04110k |
|
This journal is © The Royal Society of Chemistry 2014 |