DOI:
10.1039/C4RA03419H
(Paper)
RSC Adv., 2014,
4, 28623-28627
One dimensional CdS nanostructures: heterogeneous catalyst for synthesis of aryl-3,3′-bis(indol-3-yl)methanes†
Received
15th April 2014
, Accepted 16th June 2014
First published on 16th June 2014
Abstract
Herein, we have developed a novel heterogeneous catalytic system for synthesis of bis(indol-3-yl)methanes using one dimensional (1D) CdS nanorods. CdS nanorods were synthesized by a solvothermal reaction technique at 200 °C over 12 h in the presence of ethylenediamine as a solvent. The prepared 1D CdS was characterized using various spectroscopic methods. X-ray diffraction (XRD) revealed the formation of highly crystalline CdS having a wurtzite structure. FESEM analysis confirms the formation of a rod like morphology with length around 150 to 200 nm and diameter ∼15 nm. TEM also validates the formation of uniform size one dimensional (1D) CdS nanostructures. The catalytic activity of 1D CdS as a Lewis acid was investigated for the synthesis of bis(indol-3-yl)methanes (BIMs) with various substituted aldehydes and indoles within shorter reaction time affording the corresponding product in excellent yield.
1. Introduction
Indole and its derivatives have received significant attention from organic chemists and researchers due to its wide range of biological activities1 such as antibacterial, cytotoxic, antioxidant, insecticidal,2,3 antimicrobial, and antifungal.4 Bis(indol-3-yl)methanes (BIMs) are used as bioactive intermediates in pharmaceuticals,5 agrochemicals6 and material science.7 They are also used as tranquilizers8 in the treatment of cancer, AIDS, fibromyalgia, chronic fatigue, and irritable bowel syndrome. Various methods have been reported for the synthesis of these derivatives which involves condensation of indole with various aldehydes or ketones employing Lewis acids9 or protic acids10 as a catalyst. The synthesis of bis(indol-3-yl)methanes has been also reported using diverse range of catalysts like, Ln(OTf)3,11 FeCl3,12 KHSO4,13 In(OTf)3,14 Dy(OTf)3,15 LiClO4,16 I2,17 NBS,18 CAN,19 NaHSO4·SiO2,20 H–Y zeolite,21 ion exchange resin,22 H3PMo12O40·xH2O,23 clay24 or using different ionic liquids in association with In(OTf)3 or FeCl3·6H2O.25 Recently, Mulla et al. reported synthesis of bis(indol-3-yl)methanes using ionic liquid (EAN) as a catalyst.26 Most of the reported methods suffer from various disadvantages such as prolonged reaction time, harsh reaction conditions, use of expensive Lewis acids, toxic metal ions and tedious work-up procedures, lack of reusability and loss of expensive metals/ligands.27 All the above reaction provides high reaction rate with good selectivity and yield. But majority of these reactions are carried out using homogeneous catalysis. Recently, the use of heterogeneous catalysis in organic synthesis has augmented appreciable interest due to higher reaction yield, recyclability and milder reaction conditions.28,29 Several researchers have reported the use of oxide based catalyst for the synthesis of bis(indol-3-yl)methanes. Moreover, carbon–carbon coupling reactions have been reported using noble metal loaded on graphene oxide,30 carbon nanotubes,31 cellulose32 etc. Of late the use of metal sulphide nanostructures for organic transformation have been reported in presence of light.33 In this regards here in, we have synthesized one dimensional cadmium sulfide (CdS) nanostructures and performed the synthesis of bis(indol-3-yl)methanes under ambient reaction conditions. To the best of our knowledge this is the first report exploring the activity of CdS nanorods as heterogeneous catalyst for synthesis of bis(indolyl)methanes having excellent yield under mild reaction conditions. In the present work we have reported inexpensive and effective use of heterogeneous catalyst for the synthesis of bis(indol-3-yl)methanes avoiding use of toxic reagents or solvents.
2. Experimental section
2.1. Materials and reagent
Cadmium nitrate (Cd(NO3)2·4H2O), thiourea, ethylenediamine (EDA, C2H4(NH2)2), of Sisco Research Laboratory (SRL) make were used for the synthesis of CdS nanostructures. All other chemicals solvents were of analytical grade and used as received without further purification.
2.2. Synthesis of CdS nanorods
The cadmium sulfide (CdS) nanorods were synthesized using solvothermal technique by dissolving Cd(NO3)2·4H2O (10 mmol) and thiourea (20 mmol) separately in 30 mL of ethylenediamine (EDA) respectively and mixed together with constant stirring. The resultant mixture was stirred for 10 min, transferred into Teflon lined stainless steel autoclave and was further heated at 200 °C for 12 h. The resultant mass was centrifuged, washed with distilled water several times and finally with ethanol. It was subsequently, dried at 80 °C for 4 h in an oven, well grinded in mortar and pestle and used for further physico-chemical analysis and catalytic study.
2.3. Characterisation techniques
X-ray diffraction (XRD) analysis of the synthesized material was carried out using Bruker AXS model D-8, (10 to 70° range, scan rate = 1° min−1) equipped with a monochromator and Ni-filtered Cu Kα radiation. UV-Visible absorbance spectrum was recorded using Shimadzu UV-Vis-NIR spectrophotometer (Model UV-3600) over a wavelength range of 300 to 900 nm. The morphological characterization of the samples was accomplished using HITACHI S-4800. Microstructure analysis was carried out using transmission electron microscopy performed by Technai 20 G2 (FEI, Netherlands) microscope operating at 200 kV.
2.4. General procedure for synthesis of bis(indol-3-yl)methanes
In a 25 mL round bottom flask, aldehyde (1.0 mmol), indole (2.0 mmol), cadmium sulphide (5 mol%, catalytic amount) and methanol (5 mL) was added, reaction mixture was refluxed for 15 min. The progress of the reaction was monitored by TLC (30% hexane–ethyl acetate). After completion of the reaction, the catalyst was filtered and resulting crude product was extracted with ethyl acetate, dried over anhydrous sodium sulfate and the solvent was evaporated under reduced pressure. The crude product was purified by silica column chromatography using hexane–ethyl acetate (90
:
10 v/v) as eluent. 1H NMR spectra were recorded at Varian 400 MHz NMR spectrophotometer with CDCl3 or DMSO-d6 as a solvent. Proton chemical shifts (δ) are relative to TMS (δ = 0) as internal standard and expressed in ppm. Coupling constants (J) are given in Hertz. FTIR spectra were recorded using Perkin-Elmer 1600 A in the range of 400–4000 cm−1. UV-Visible absorption spectrum of BIMs was recorded by double beam spectrophotometer (Shimadzu, UV-3600) in the range of 300–900 nm. Melting points were determined on Buchi M-560. Mass spectral data was obtained from LC-MS (Shimadzu 2010). Reaction was monitored by thin layer chromatography (TLC) on Merck's silica gel plates (60 F254). The characterization data MP, NMR, IR of these compounds were found to be identical with those reported in the literature.
3. Results and discussion
3.1. X-ray diffraction analysis
The synthesized CdS nanostructure was analyzed by powder X-ray diffractometry to identify the crystalline phase. XRD pattern of CdS prepared at 12 h is depicted in Fig. 1. The diffraction peaks at 2θ = 24.8, 26.5, 28.1, 36.6, 43.6, 47.8 and 51.8° can be indexed to (100), (002), (101), (102), (110), (103) and (112) crystal planes of hexagonal CdS (PDF card 41-1049 JCPDS) respectively. The intensity of (002) peak is higher than the other diffraction peaks of (100) and (101), this revealed the high crystalline order along c-axis and poor crystalline order along x–y plane resulting one dimensional growth. Additional peaks due to other impurities were not observed indicating formation of highly pure CdS nanostructure.
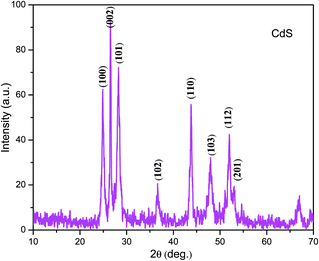 |
| Fig. 1 X-ray diffraction pattern of CdS nanostructures. | |
3.2. FE-SEM analysis
The surface morphology of CdS sample was examined by FESEM, high and low resolution FESEM images are reproduced in Fig. 2. CdS prepared at 12 h of reaction time reveals the formation of rod like morphology with uniform size and smooth surface, the observed thickness of the rods are ∼15 nm and length in the range of 150 to 200 nm (Fig. 2a and b).
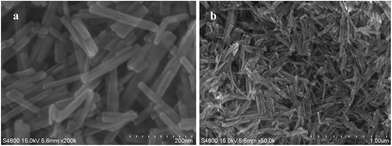 |
| Fig. 2 FESEM micrographs of CdS nanostructures with (a) high and (b) low resolution. | |
3.3. TEM analysis
The microstructure of synthesized CdS was investigated by TEM analysis. TEM images along with selected area electron diffraction (SAED) pattern are depicted in Fig. 3. TEM images of CdS nanostructure indicate the formation of uniform size nano rods having diameter 10 to 15 nm and length 100 to 150 nm (Fig. 3a). SAED pattern revealed the formation of polycrystalline CdS and confirm the hexagonal phase of CdS (inset of Fig. 3b). TEM analysis supports the XRD observations and confirms the formation of nanorods.
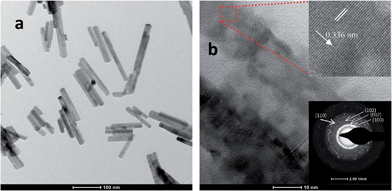 |
| Fig. 3 (a and b) TEM analysis of CdS nanostructure, inset of (b) is SAED pattern and high resolution TEM image. | |
3.4. Synthesis of bis(indol-3-yl)methanes using CdS nanorods as a catalyst
To establish the general reaction conditions for the synthesis of bis(indol-3-yl)methanes a model reaction was carried out with p-nitrobenzaldehyde and indole using CdS nanorods as a catalyst.
In order to find the appropriate concentration of CdS for BIM synthesis, the catalyst amount varied from 0.5 mol% to 10 mol%, the results are depicted in Table 1.
Table 1 Optimization of amount of catalystb
Entry |
CdS (x mmol) |
Yielda |
Isolated yield after chromatographic separation. Reaction conditions: aldehyde (1 mmol), indole (0.117 g, 2 mmol), catalyst (x mol%) were stirred in 5 mL methanol at reflux temperature, time 15 min. |
1 |
0.5 |
72% |
2 |
1.0 |
83% |
3 |
2.5 |
90% |
4 |
5.0 |
95% |
5 |
7.5 |
96% |
6 |
10.0 |
96% |
With increase in catalyst amount the yield of the reaction increases from 72% to 96% respectively. Based on these results for further study the 5 mol% catalyst was used.
With higher catalyst amount (>5 mol%) the yield of the product was around 96% this indicates that further increase in catalyst amount did not show any appreciable enhancement in the yield of desired product. Hence, for further study 5 mol% catalyst was found to be effective. The influence of various protic, polar aprotic and non polar solvents on the yield of BIMs was also investigated using 5 mol% CdS nanorods and results are shown in Table 2. The reaction in water gave only 10% yield after prolonged reaction time; the lower yield was due to the inhomogeneity of reactants. Yield of BIMs in methanol was almost more than 95% whereas, in ethanol it was 65%. Other polar aprotic solvents (CH3CN, CH2Cl2, THF, CHCl3, DMF) affords moderate yield (Table 2 entry 4 to 7 and 9), while in non-polar solvents (1,4-dioxane, toluene) the yield of corresponding product is low which require longer reaction time (Table 2 entry 8 and 10). Among the studied solvents methanol gave the excellent yield of bis(indol-3-yl)methanes within 15 min under reflux reaction condition. For further investigation we employed 5 mol% catalyst and methanol as a solvent. To explore the further applicability of this reaction, we extended the methodology for the synthesis of variety of bis(indol-3-yl)methanes using various aromatic aldehydes with either electron-releasing or electron-withdrawing substituent's in the ortho, meta and para positions (Scheme 1), the results are summarized in Table 3. The benzaldehyde and halogenated benzaldehydes gave almost more than 87% yield (Table 3, entries 1–4).
Table 2 Influence of solvent on bis(indol-3-yl)methanes synthesisc
Entry |
Solvent |
Time |
Yieldb |
Reaction at room temperature. Isolated yield after chromatographic separation. Reaction conditions: aldehyde (1 mmol), indole (2 mmol), catalyst (5 mol%) were stirred in 5 mL methanol at reflux temperature. |
1 |
H2O |
4.5 h |
>10% |
2 |
MeOH |
15 min |
95% |
2a |
MeOH |
120 min |
7% |
3 |
EtOH |
15 min |
65% |
4 |
Acetonitrile |
40 min |
89% |
5 |
DCM |
55 min |
82% |
6 |
THF |
50 min |
80% |
7 |
CHCl3 |
45 min |
88% |
8 |
1,4-dioxane |
60 min |
76% |
9 |
DMF |
90 min |
60% |
10 |
Toluene |
40 min |
40% |
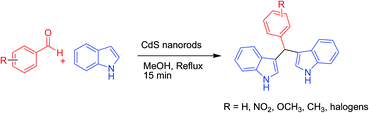 |
| Scheme 1 | |
Table 3 Synthesis of bis(indol-3-yl)methanes using CdS nanorodsb
Entry |
Aldehye |
Product yielda (%) |
Isolated yield after chromatographic separation. Reaction conditions: aldehyde (1 mmol), indole (2 mmol), catalyst (5 mol%) were stirred in 5 mL methanol at reflux temperature for 15 min. |
1 |
Benzaldehye |
87 |
2 |
4-Fluorobenzaldehye |
89 |
3 |
4-Trifluorobenzaldehye |
93 |
4 |
4-Bromobenzaldehye |
92 |
5 |
4-Chlorobenzaldehye |
90 |
6 |
5-Bromo-2-fluorobenzaldehye |
93 |
7 |
4-Chloro-2-fluorobenzaldehye |
92 |
8 |
4-Nitrobenzaldehye |
95 |
9 |
4-Methoxybenzaldehye |
90 |
10 |
4-Hydroxybenzaldehye |
90 |
11 |
3-Hydroxybenzaldehye |
95 |
12 |
4-Methoxy-3-hydroxybenzaldehye |
90 |
13 |
3,4-Dimethoxybenzaldehye |
90 |
14 |
4-N,N-Dimethylbenzaldehye |
85 |
15 |
4-Formylbenzoic acid |
87 |
16 |
5-Bromo-piconilaldehyde |
92 |
17 |
2-Bromoisonicotinaldehyde |
92 |
18 |
2-Bromonicotinaldehyde |
94 |
Trifluorobenzaldehyde afforded 93% yield of corresponding BIM (Table 3, entry 3). Di substituted halogenated benzaldehyde under similar reaction condition leads to ∼92% yield of BIM (Table 3, entry 6 and 7). It was observed that the reaction proceeds at faster rate with all the aldehydes possessing electron-withdrawing groups on the aromatic ring (Table 3, entry 8) than aldehydes possessing electron-donating substituent's (Table 3, entries 9–13) giving good to excellent yield of corresponding products. Additionally, the methodology was further extended for heterocyclic aldehydes, affording more than 90% yield (Table 3 entries 16–18). The reusability of catalyst was studied by the reaction of 4-nitrobenzaldehyde with indole in presence of CdS as a catalyst for desired time under reflux reaction condition. After completion of reaction the catalyst was recovered, filtered, washed repeatedly with ethyl acetate and dried. It was reused under optimized reaction conditions and was found that yield of bis(indol-3-yl)methanes was almost comparable. The reusability of catalyst checked for four cycles and the corresponding yields obtained in each cycle is summarized in (Table 4). The decrease in the catalytic activity of CdS was might be due to the deactivation of active sites of catalyst. To confirm the deactivation of catalyst we heated the CdS recovered after fourth run at 150 °C in N2 atmosphere for 60 min and again reused for the reaction which gave 94% yield. This confirmed reusability of the catalyst without loss in activity.
Table 4 Recyclability of catalystc
Entry |
Number of cycles |
Yielda |
Isolated yield after chromatographic separation. Used CdS catalyst from fourth recycle, heated at 150 °C for 60 min in N2 atmosphere. Reaction conditions: aldehyde (1 mmol), indole (2 mmol), catalyst (5 mol%) were stirred in 5 mL methanol at reflux temperature, time 15 min. |
1 |
Fresh |
95% |
2 |
First |
92% |
3 |
Second |
89% |
4 |
Third |
85% |
5 |
Fourth |
80% |
6b |
Fifth |
94% |
Overall, the synthesis of BIMs using CdS nanorods with higher yield was the first report. In this reaction CdS acts as Lewis acid, the plausible mechanism is shown in Scheme 2. The electrophilic condensation reaction most likely proceeds through activation of a carbonyl group (intermediate i) followed by attack of indole to give azafulvenium salt (intermediate ii).
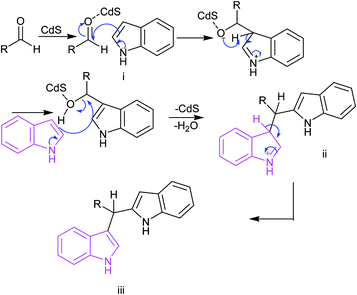 |
| Scheme 2 | |
Further, another molecule of indole reacts with intermediate (ii) followed by subsequent loss of H2O and CdS to give corresponding bis(indol-3-yl)methane (iii).
We compared our data with the reported results in the literature and details are given in Table 5. In comparison with these reagents our approach gives higher yield under ambient reaction conditions.
Table 5 Comparison between CdS nanorods and other catalysts used to synthesis bis(indol-3-yl)methanes
Sr. no. |
Reagent and solvent |
Time |
Yield (%) |
Ref. |
1 |
In(OTf)3/CH3CN |
25 min |
71 |
14 |
2 |
[bmim]BF4 |
4.5 h |
87 |
16b |
3 |
AlPW12O40/CH3CN |
40 min |
92 |
34 |
4 |
Acidic ionic liquid immobilized on silica (ILIS)/CH3CN, rt |
5.5 h |
97 |
35 |
5 |
CdS nanostructure/MeOH, reflux |
15 min |
95 |
Present work |
3.5. Characterization data
3,3′-((4-Fluorophenyl) methylene bis(1H indole)). (Table 3, entry 2); 1H NMR δ (400 Hz, CDCl3, Me4Si) 5.8 (1H, s, CH), 6.6 (2H, s, Ph), 6.93–7.02 (4H, m, Ph), 7.15–7.2 (2H, m, Ph), 7.27–7.30 (3H, m, Ph), 7.36 (4H, dd, J = 2 and 7.60 Hz, Ph), 7.9(2H, br s, NH) mp 160–162 °C.
3,3′-((4-Bromophenyl) methylene bis(1H indole)). (Table 3, entry 4);1H NMR δ (400 Hz, CDCl3, Me4Si) 5.8 (s, 1H, CH), 6.67 (4H, d, Ph), 6.82 (2H, m, Ph), 7.1–7.21 (4H, m, Ph), 7.3 (4H, d, J = 8.3 Hz, Ph), 7.8 (2H, bs, NH) mp 120–125 °C.
3,3′-((4-Nitrophenyl) methylene bis(1H indole)). (Table 3, entry 8); δ (400 Hz, DMSO-d6, Me4Si) 6 (1H, s, CH), 6.8 (2H, d, J = 8.4, CH), 6.7 (2H, d), 6.84 (2H, t, J = 8.0 and 15.2 Hz, Ph), 7.01 (2H, t, J = 7.60 and 14.8 Hz, Ph), 7.1 (2H, d, J = 8.4 Hz, Ph), 7.2 (2H, d, J = 8.0 Hz, Ph), 7.3 (2H, d, J = 8.4 Hz, Ph), 10.8 (1H, bs, NH); mp 170–174 °C.
3,3′-((3-Hydroxyphenyl) methylene bis(1H indole)). (Table 3, entry 11); brown solid (from 20% EtOAc–hexane); 1H NMR δ (400 Hz, DMSO-d6, Me4Si) 5.69 (1H, s, CH), 6.5 (2H, d, Ph), 6.78 (2H, m, Ph), 6.86 (2H, t, J = 7.6 and 15.2 Hz, Ph), 7.0 (4H, t, J = 8.0 and 16 Hz, Ph), 7.2 (2H, d, J = 8.0 Hz, Ph), 7.3 (2H, d, J = 8.4 Hz, Ph), 9.1 (1H, bs, OH), 10.7 (2H, bs, NH), mp 158–162 °C.
3,3′-((3,4-Dimethoxyphenyl) methylene bis(1H indole)). (Table 3, entry 13); 1H NMR δ (400 Hz, CDCl3, Me4Si) 3.7 (3H, s, OMe), 3.8 (3H, s, OMe), 5.8 (1H, s, CH), 6.6 (2H, s, Ph), 6.77 (1H, d, J = 8.4 Hz, Ph), 6.83 (1H, m, Ph), 6.9 (1H, s, Ph), 7.0 (2H, t, J = 7.6 and 16 Hz, Ph), 7.1 (2H, t, J = 8.0 and 14.8 Hz, Ph), 7.3 (4H, dd, J = 8.0 Hz, Ph), 7.9 (2H, bs, Ph) mp 180–183 °C.
3,3′-((4-N,N-Dimethylphenyl) methylene bis(1H indole)). (Table 3, entry 14); brown solid (from 20% EtOAc–hexane); 1H NMR δ (400 Hz, CDCl3, Me4Si) 2.9 (6H, s), 5.8 (s, 1H, CH), 6.67 (4H, d, Ph), 6.9 (2H, m, Ph), 7.2–1.12 (4H, m, Ph), 7.3 (2H, d, J = 8.4 Hz, Ph), 7.4 (2H, d, J = 8.0, Ph), 7.8 (2H, bs, NH), mp 181–183 °C.
4. Conclusions
A simple methodology for an efficient synthesis of a variety of biologically active bis(indol-3-yl)methanes using one dimensional CdS nanostructures as a heterogeneous catalyst is reported. It offers short reaction time, high conversions and simple and easy workup procedure compared to the traditional methods of synthesis.
Acknowledgements
Authors sincerely acknowledge University Grants Commission, New Delhi, ISRO University of Pune for financial assistance and C-MET, Pune for characterization. We also thank Nowrosjee Wadia College Pune for providing lab facility.
Notes and references
- R. Bell, S. Carmeli and N. Sar, J. Nat. Prod., 1994, 11, 1587 CrossRef.
-
(a) P. Kaur, S. Koura and K. Singh, Org. Biomol. Chem., 2012, 10, 1497 RSC;
(b) P. Bose and P. Ghosh, Chem. Commun., 2010, 46, 2962 RSC;
(c) R. Martinez, A. Espinosa, A. Tarraga and P. Molina, Tetrahedron, 2008, 64, 2184 CrossRef CAS PubMed.
- S. Hibino and T. Choshi, Nat. Prod. Rep., 2001, 18, 66 RSC.
- G. Sivaprasad, P. T. Perumal, V. R. Prabavathy and N. Mathivanan, Bioorg. Med. Chem. Lett., 2006, 24, 6302 CrossRef PubMed.
-
(a) R. J. Sundberg, The Chemistry of Indoles, Academic Press New York, USA, 1970 Search PubMed;
(b) R. J. Sundberg, Indoles, Academic Press, London, 1996 Search PubMed.
- J. R. Plimmer, D. W. Gammon and N. N. Ragsdale, Encyclopedia of Agrochemicals, John Wiley and Sons, New York, 2003, p. 3 Search PubMed.
- S. C. Minne, S. R. Manalis and C. F. Quate, Appl. Phys. Lett., 1995, 67, 3918 CrossRef CAS PubMed.
- K. W. Lo, K. H. K. Tsang, W. K. Hui and N. Zhu, Chem. Commun., 2003, 2704 RSC.
- G. Babu, N. Sridhar and P. T. Perumal, Synth. Commun., 2000, 30, 1609 CrossRef CAS.
- B. Gregorovich, K. Liang, D. Clugston and S. F. Canand MacDonald, J. Chem., 1968, 46, 3291 CAS.
- D. Chen, L. Yu and P. G. Wang, Tetrahedron Lett., 1996, 37, 4467 CrossRef CAS.
- M. Xia, S. B. Wang and W. B. Yuan, Synth. Commun., 2004, 34, 3175 CrossRef CAS PubMed.
- R. Nagarajan and P. T. Perumal, Chem. Lett., 2004, 33, 288 CrossRef CAS.
- R. Nagarajan and P. T. Perumal, Tetrahedron, 2002, 58, 1229 CrossRef CAS.
- X. L. Mi, S. Z. Luo, J. Q. He and J. P. Cheng, Tetrahedron Lett., 2004, 45, 4567 CrossRef CAS PubMed.
-
(a) J. S. Yadav, B. V. S. Reddy, V. S. R. Murthy, G. M. Kumar and C. Madan, Synthesis, 2001, 5, 783 CrossRef PubMed;
(b) J. S. Yadav, B. V. S. Reddy and S. Sunita, Adv. Synth. Catal., 2003, 345, 349 CrossRef CAS.
-
(a) S. J. Ji, S. Y. Wang, Y. Zhang and T. P. Loh, Tetrahedron, 2004, 60, 2051 CrossRef CAS PubMed;
(b) B. P. Bandgar and K. A. Shaikh, Tetrahedron Lett., 2003, 44, 1959 CrossRef CAS.
- H. Koshima and W. Matsuaka, J. Heterocycl. Chem., 2002, 39, 1089 CrossRef CAS.
- C. Ramesh, N. Ravindranath and B. Das, J. Chem. Res., Synop., 2003, 72 CrossRef CAS.
- C. Ramesh, J. Banerjee, R. Pal and B. Das, Adv. Synth. Catal., 2003, 345, 557 CrossRef CAS.
-
(a) M. Karthik, A. K. Tripathi, N. M. Gupta, M. Palanichamy and V. Murugesan, Catal. Commun., 2004, 5, 371 CrossRef CAS PubMed;
(b) A. V. Reddy, R. Ravinder, V. L. N. Reddy, T. V. Goud, V. Ravikanth and Y. Venkateseswarlu, Synth. Commun., 2003, 33, 3687 CrossRef PubMed.
- X. L. Feng, C. J. Guan and C. X. Zhao, Synth. Commun., 2004, 34, 487 CrossRef CAS PubMed.
- M. A. Zolfigol, P. Salehi and M. Shirl, Phosphorus, Sulfur Silicon Relat. Elem., 2004, 179, 2273 CrossRef CAS.
-
(a) M. Chakrabarty, N. Gosh, R. Basak and Y. Harigaya, Tetrahedron Lett., 2002, 43, 4075 CrossRef CAS;
(b) G. Penieres-Carrillo, J. G. Garcia-Estrada, J. L. Gutierrez-Ramirez and C. Alvarez-Toledano, Green Chem., 2003, 5, 337 RSC.
-
(a) S. J. Ji, M. F. Zhou, D. G. Gu, S. Y. Wang and T. P. Loh, Synlett, 2003, 2077 CrossRef CAS PubMed;
(b) S. J. Ji, M. F. Zhou, D. G. Gu, Z. Q. Jiang and T. P. Loh, Eur. J. Org. Chem., 2004, 7, 1584 CrossRef.
- S. A. R. Mulla, A. Sudalai, M. Y. Pathan, S. A. Siddique, S. M. Inamdar, S. S. Chavan and R. S. Reddy, RSC Adv., 2012, 2, 3525 RSC.
- C. E. Garrett and K. Prasad, Adv. Synth. Catal., 2004, 346, 889 CrossRef CAS.
- L. Yin and J. Liebscher, Chem. Rev., 2007, 107, 133 CrossRef CAS PubMed.
- A. Molnar, Chem. Rev, 2011, 111, 2251 CrossRef CAS PubMed.
- R. Nie, J. Shi, W. Du and Z. Hou, Appl. Catal., A, 2014, 473, 1 CrossRef CAS PubMed.
- B. Yoon and C. M. Wai, J. Am. Chem. Soc., 2005, 127, 17174 CrossRef CAS PubMed.
- W. Chen, L. Zhong, X. Peng, K. Wang, Z. Chena and R. Sun, Catal. Sci. Technol., 2014, 4, 1426 CAS.
-
(a) O. Bunsho, K. Satoshi, O. Katsumi, T. Shigeto, N. Sei-ichi, A. Yusuke, I. Kunisuke, N. Yoshihiro, M. Michio and N. Yasuhiro, J. Chem. Soc., Perkin Trans. 2, 2001, 2, 201 Search PubMed;
(b) R. Arne, V. E. Rudi and K. Horst, J. Phys. Chem. B, 2000, 104, 1014 CrossRef.
- H. Firouzabadi, N. Iranpoor and A. A. Jafari, J. Mol. Catal. A: Chem., 2006, 244, 168 CrossRef CAS PubMed.
- H. Hagiwara, M. Sekifuji, T. Hoshi, K. Qiao and C. Yokoyama, Synlett, 2007, 1320 CrossRef CAS PubMed.
Footnote |
† Electronic supplementary information (ESI) available: 1H and 13C NMR spectra of selected compounds are shown. See DOI: 10.1039/c4ra03419h |
|
This journal is © The Royal Society of Chemistry 2014 |
Click here to see how this site uses Cookies. View our privacy policy here.