DOI:
10.1039/C4RA02829E
(Paper)
RSC Adv., 2014,
4, 23191-23201
Poly(arylene ether sulfone) containing thioether units: synthesis, oxidation and properties
Received
31st March 2014
, Accepted 15th May 2014
First published on 15th May 2014
Abstract
Two kinds of high glass temperature poly(arylene ether sulfone)s containing thioether and biphenyl units (PASSs) have been developed. The polymers were prepared by a polycondensation reaction of 4,4′-bis(4-chlorophenylsulfone)biphenyl (BCPSB) and 4,4′-dimercaptodiphenyl sulfone (DMDPS) (or 4,4′-bis(4-mercaptophenylsulfone)biphenyl (BMPSB)). They showed good thermal properties such as a relatively high glass transition temperature of 263–282 °C and a 5% weight-loss temperature (T5%) of 441–445 °C. The water flux and retention rate of the resultant membranes were 0.61–2.48 L m−2 h−1 and 89.47–94.91%, respectively. Interestingly, we found that the corrosion resistance of the membranes had been largely improved with the oxidization treatment. They were even insoluble in NMP, DMSO, concentrated H2SO4, aqua regia and so on, while the polymers without oxidation were soluble or destroyed in above solvents. In addition, we found the water flux of the oxidized membrane was also improved but the retention rate only decreased a little.
Introduction
Membrane separation processes have become gradually important in the last three decades for their applications in high technology areas such as biotechnology and membrane-based energy devices. As a class of high performance engineering thermoplastic materials, poly(arylene ether sulfone)s (PAES) are found to have high glass transition temperature, thermal stability, good mechanical properties, solubility and excellent resistance to hydrolysis.1–11 So they have been widely used in industrial, especially in membrane separation such as microfiltration and ultrafiltration process in the fields of biomedicine, food, hemodialysis, water purification12,13 and so on. However, the performance of traditional PAES is not good enough for some special applications. With respect to improve or endow the polymer novel properties, the chemical structure modification or molecular design is frequently required. Zhao et al. modified polyether sulfone (PES) with poly(acrylic acid),14 carboxyl15 and aniline16 endow PES to be pH-sensitive or photoresponsive, respectively. M. D. Guiver and B. D. Freeman developed a series of high performance separation membranes of poly(arylene ether sulfone).17–22 In order to make the membrane can be used in much more rigorous environments. Our interest is focused on the improvement of corrosion resistance and thermal properties of PAES. In recent decades, these approaches had been reported, Jian developed a kind of membrane materials containing aromatic heterocycle,23–25 Shin and Bottino synthesized a new kind of materials with polycyclic,26,27 Park etc. developed a kind of poly(arylene ether sulfone) containing amide group.28–30 They are all high glass transition temperature membrane materials and have good corrosion resistance. Poly(aryl thioether)s, such as poly(phenylene sulfide) and poly(phenylene sulfide sulfone)31–34 are of well-known high performance engineering thermoplastics. They all have excellent thermal and corrosion resistance properties. On the basis of these considerations, we designed a kind of polymer that containing thioether, sulfonyl and diphenyl units to achieve high glass transition temperature, good corrosion resistance and film-forming properties. This article reports the synthesis and properties of PASSs containing thioether and biphenyl ring, which prepared from 4,4′-bis(4-chlorophenylsulfone)biphenyl (BCPSB) and 4,4′-dimercaptodiphenyl sulfone (DMDPS) (or 4,4′-bis(4-mercaptophenylsulfone)biphenyl (BMPSB)). The resultant PASSs exhibited high glass transition temperature in the range of 263–282 °C. They were 40–60 °C higher than that of polyether sulfone (Tg: ∼230 °C) and PVDF (Tg: ∼40 °C). They can be used in higher temperature environment. Interestingly, we found that the corrosion resistance of the membranes had been largely improved with moderate oxidation treatment. They were even insoluble in NMP, DMSO, concentrated H2SO4, aqua regia and so on, while the polymers without oxidation were soluble or destroyed in above solvents. We studied the water flux and retention rates of the membranes. And the mechanism of the oxidization reaction was also studied in detail.
Experimental
Materials
Commercially available 4,4′-bis(4-chlorophenylsulfone)biphenyl (BCPSB) (99%, ShenZhen, Advanced Technology & Industrial Company Limited), 4,4′-dichlorodiphenylsulfone (DCDPS) (AR, Suzhou Yinsheng Chemical Industry Company), sodium hydroxide (NaOH) (AR, SiChuan ChengDu ChangLian Chemical Reagent Company), sodium hydrosulfide (NaHS·xH2O, NaHS% ≈ 70%) (Nafine Chemical Industry Group Co., Ltd.), N-methyl-2-pyrrolidone (NMP) (JiangSu NanJing JinLong Chemical Industry Company), sodium benzoate, sodium 4-methylbenzenesulfonate (AR, Aladdin Reagent Company) and other reagents and solvents were commercially obtained.
Monomer synthesis
4,4′-Bis(4-mercaptophenylsulfone)biphenyl (BMPSB) (shown in Scheme 1). DMF (600 mL), sodium hydrosulfide (48 g, 0.6 mol), BCPSB (100.6 g, 0.2 mol), sodium benzoate (2.8 g, 0.02 mol) and sodium 4-methylbenzenesulfonate (3.8 g, 0.02 mol) were added into a 1000 mL three-necked flask fitted with a water segregator, reflux condenser, mechanical stirrer and thermometer. The mixture was stirred at 140 °C for 1 h; during this time, 33.6 mL of liquid was removed. The reaction flask was stirred at 150 °C for another 6 h (the little amount H2S produced during the reaction must be trapped and oxidized by passing through a solution of NaOH and NaOCl.). The reaction solution was then poured into the mixture of ice and water. Then the solution was filtered, the filter liquor was acidified with 1 mol L−1 HCl to precipitate a cottony crude product under stirring. The crude product was washed several times with deionized water to remove possible residual salts. Next, the purified product was filtered, dissolved in a solution of NaOH, filtered and precipitated in hydrochloric acid. After filtration and washing twice by the precipitant, the product was vacuum-dried at 30 °C for 36 h to yield a pale-yellow powder.
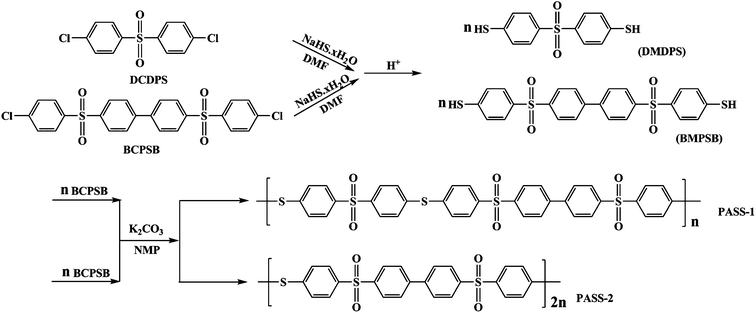 |
| Scheme 1 Synthesis routes of monomers and PASSs. | |
Yield: 65.5 g, 65.8%. Elemental analysis (%): found: C, 57.46 (57.81); H, 3.49 (3.64); S, 25.83 (25.72) (data in brackets are calculated); FT-IR (KBr, cm−1): 3081 (C–H aromatic ring), 2562 (–SH), 1578, 1477 (C
C aromatic ring), 1315, 1156 (–SO2–), 1072 (C–S–C), 821 (para-substituent of aromatic ring); 1H-NMR (600 MHz, DMSO-d6/TMS, ppm): 3.391 (s, 2H, H1), 7.500–7.514 (d, 4H, H2), 7.797–7.810 (d, 4H, H3), 7.910–7.924 (d, 4H, H4), 8.005–8.019 (d, 4H, H5).
4,4′-Dimercaptodiphenyl sulfone (DMDPS) was synthesized with a similar method as our earlier reports.11
Polymer synthesis (PASS-1) (shown in Scheme 1). A typical polymerization was performed as shown in Scheme 1. In a 250 mL three-necked flask fitted with a mechanical stirrer and thermometer, DMDPS (14.1 g, 0.05 mol), BCPSB (25.2 g, 0.05 mol), potassium carbonate (10.4 g, 0.075 mol), toluene (30 mL) and NMP (120 mL) were added. The mixture was stirred at 130–180 °C for 1 h to remove water; then stirred at 190–200 °C for 6 h to yield a viscous pale-yellow solution. Next, the reaction solution was poured into water to obtain a white fibrous precipitate. Then the precipitate was washed with water and ethanol, and dried under vacuum. At last the fibrous precipitate was pulverized to powder, and washed with water and ethanol again. After drying under vacuum at 100 °C for 12 h, 33.4 g (93.7%) of PASS-1 was obtained.PASS-1: elemental analysis (%), found: C: 60.23 (60.57); H: 3.50 (3.53); S: 22.74 (22.46) (data in brackets are calculated). FT-IR (casting film, cm−1): 3087 (C–H aromatic ring), 1590, 1572, 1473 (C
C aromatic ring), 1315, 1153 (–SO2–), 1071 (C–S–C), 817 (para-substituent of aromatic ring). 1H-NMR (600 MHz, DMSO-d6/TMS, ppm): 7.407–7.586 (m, 8H, H1–2), 7.801–7.885 (m, 8H, H3–4), 7.931–7.983 (d, 4H, H5), 8.016–8.086 (d, 4H, H6). 13C-NMR (Ca–Cl): 116.12, 121.93, 126.11, 128.15, 128.65, 130.34, 131.30, 131.41, 132.02, 139.73, 139.86, 140.57.
PASS-2 was prepared following a similar procedure as that of PASS-1.
PASS-2: 16.3 g, yield: 91%, elemental analysis (%), found: C: 61.78 (61.91); H: 3.55 (3.68); S: 21.02 (20.66) (data in brackets are calculated). FT-IR (casting film, cm−1): 3063 (C–H aromatic ring), 1591, 1573, 1475 (C
C aromatic ring), 1317, 1156 (–SO2–), 1076 (C–S–C), 820 (para-substituent of aromatic ring). 1H-NMR (600 MHz, DMSO-d6/TMS, ppm): 7.539–7.561 (d, 4H, H1), 7.841–7.861 (d, 4H, H2), 7.940–7.960 (d, 4H, H3), 8.045–8.066 (d, 4H, H4). 13C-NMR (Ca–Ck): 116.67, 126.23, 128.16, 128.69, 130.94, 131.35, 139.89, 140.59.
We also synthesized comparative samples poly(phenylene sulfide sulfone) (PPSS) to compare their thermal properties with PASS-1 and PASS-2.
Preparation of membranes
The PASSs solution (16–22 wt%) were cast onto glass plates after degassing. The membranes were immediately immersed into the deionized water at room temperature for phase separation. Then the resultant membranes were stored in deionized water bath to remove the residual solvent NMP. The thickness of the membranes was in the range of 110–126 μm.
Oxidation of membranes
These PASSs membranes were oxidized in a solution of glacial acetic acid with 30% hydrogen peroxide and about 1–5% content of concentrated sulfuric acid for about 24–36 h at room temperature. After that the membranes were immersed into the deionized water.
Characterization
Limiting viscosity and molecular weights analysis: the limiting viscosities
of PASSs were obtained by one-point method at 30 ± 0.1 °C with 0.500 g of polymer dissolved in 100 mL of NMP, using a Cannon-Ubbelhode viscometer:
where ηr = η/η0, ηsp = η/η0 − 1.
The number average molecular weights (Mn) and weight average molecular weight (Mw) were obtained via GPC performed with a Waters 1515 performance liquid chromatography pump, a Waters 2414 differential refractometer (Waters Co., Milford, MA) and a combination of Styragel HT-3 and HT-4 columns (Waters Co., Milford, MA), the effective molecular weight ranges of which were 500–30
000 and 5000–800
000, respectively. N,N-Dimethyl formamide (DMF) was used as an eluent at a flow rate of 1.0 mL min−1 at 35 °C. Polystyrene standards were used for calibration. Elemental analysis: the samples of monomer and polymers were measured with an elemental analyzer (EURO EA-3000). Chemical structure analysis: FT-IR spectroscopic measurements were performed on a NEXUS670 FT-IR instrument. Nuclear magnetic resonance (1H-NMR and 13C-NMR) spectra were determined on a BRUKER-600 NMR spectrometer in deuterated chloroform or deuterated dimethyl sulfoxide. Thermal analysis: differential scanning calorimetry (DSC) was performed on a NETZSCH DSC 200 PC thermal analysis instrument. The heating rate for the DSC measurements was 10 °C min−1. Thermogravimetric analysis (TGA) measurements were performed on a TGA Q500 V6.4 Build 193 thermal analysis instrument with a heating rate of 10 °C min under nitrogen atmosphere. Mechanical testing: an Instron Corporation 4302 was used to study the stress–strain behavior of the films (according to GB 13022-91). Membrane cross-sections were prepared by mechanically fractured in liquid nitrogen and sputtering-coated with a thin layer of gold, and then examined under a JSM 6701 field emission scanning electron microscope (SEM) operated at 10 keV. The samples were also characterized by atomic force microscopy (AFM-SPA-400 SPM UNIT). XPS analysis was used to evaluate the element valence and quantity changes on the membrane surfaces. It was carried out on a Krais ULFRADLD spectrometer employing a MgKα (1253.6 eV) achro-matic X-ray source operated at 14 keV with a total power of 300 W. The retention rates of the membranes were evaluated by deionized water and 100 mg L−1 bovine serum albumin solutions in a dead-end ultrafiltration (UF) cell with an effective membrane. The pure water flux (J) through the membrane could be calculated by the following equation:
where
V is the volume of permeate,
A is the membrane area, Δ
t is the permeation time and Δ
p is
trans-membrane pressure.
The solutes (bovine serum albumin) retention rate (R) was defined as the following equation:
where
Cp and
Cf (mg mL
−1) were the concentration of permeate and feed solutions, respectively. The concentrations of the feed and permeate solutions were examined by UV-Vis spectroscopy. Experiment of solubility: the solubility of polymers in various solvents was tested at room temperature and the boiling point of the solvent.
Results and discussion
Synthesis of monomers
In this study, the monomer (BMPSB) containing sulfone and thiol groups was prepared with the nucleophilic substitution reaction in the presence of sodium benzoate and sodium 4-methylbenzenesulfonate. This reaction generated little amount byproduct such as hydrogen sulfide, so the exhaust must be absorbed by the solution of NaOH and NaOCl. It is better to control the temperature of vacuum-drying below 35 °C, or BMPSB may be oxidized to disulfide compounds. The structure of BMPSB was characterized by elemental analysis, FT-IR and 1H-NMR spectrum. The FT-IR spectra of BMPSB showed characteristic absorptions near 2560, 1320, 1150 and 1075 cm−1 attributed to the thiol, sulfone and thio–ether groups, respectively. Fig. 1 shows the 1H-NMR spectrum of BMPSB consisting of one single peak at 3.391 ppm and four double signals at 7.500–8.019 ppm which due to the thiol and benzene protons, respectively. The ratio of the corresponding integral curves was also about 1
:
2
:
2
:
2
:
2. That is consistent with the calculated results.
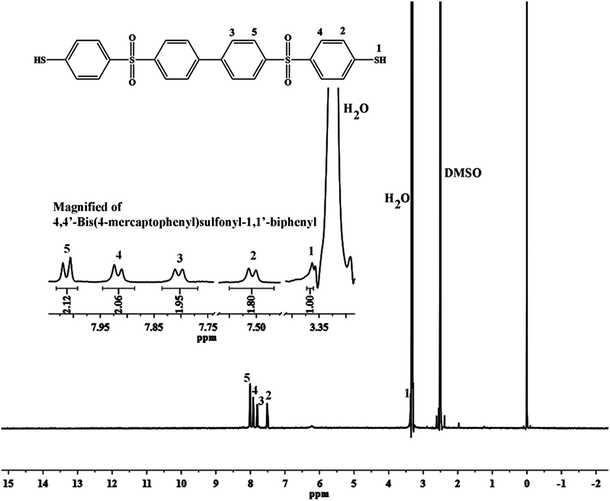 |
| Fig. 1 1H-NMR spectrum of BMPSB. | |
Synthesis and characterization of polymers
The polycondensation reaction was carried out by nucleophilic substitution polymerization using potassium carbonate as the catalyst. The reaction temperature was about 180–200 °C. The molecular weights of the polymers were measured by limiting viscosity and GPC. As shown in Table 1, the [η] values of PASS-1 and PASS-2 were 0.56 and 0.49 dL g−1, the Mn values were 5.2 × 104 and 4.3 × 104, Mw values were 9.7 × 104 and 8.9 × 104, respectively. The polydispersity indices (PDIs) of polymers PASS-1 and PASS-2 were 1.86 and 2.07, respectively.
Table 1 Limiting viscosity ([η]), molecular weights and thermal properties of PASSs
Polymers |
[η] (dL g−1) |
Mn (g mol−1) |
Mw (g mol−1) |
PDI (Mw/Mn) |
PASS-1 |
0.56 |
5.2 × 104 |
9.7 × 104 |
1.86 |
PASS-2 |
0.49 |
4.3 × 104 |
8.9 × 104 |
2.07 |
The structures of the resultant polymer were characterized by elemental analysis, FT-IR and NMR spectrum. The FT-IR spectra (Fig. 2) of polymers exhibited the characteristic stretching absorption of sulfone and thioether near 1315, 1150 and 1070 cm−1, respectively. Comparing with the spectrum of monomer (BMPSB), the characteristic absorption around 2560 cm−1 (–SH) disappeared. Fig. 3 shows the 1H-NMR spectrum of PASS-1. The signals of protons on benzene ring were in the range of 7.407–8.086 ppm. Four groups of peaks appeared in the 1H-NMR spectra. The calculated result should have six group peaks and the ratio of corresponding integral curves was 1
:
1
:
1
:
1
:
1
:
1, but the chemical shift of H1 and H2, H3 and H4 was so approximate that it could not be separated. The ratio of corresponding integral curves was (H1 + H2) :
(H3 + H4)
:
H5
:
H6 = 2
:
2
:
1
:
1. The 13C-NMR spectrum of PASS-1 showed twelve groups of peaks Ca–Cl ranged from 116.12 to 140.57 ppm. Combined with the FT-IR and elemental analysis results suggest that the polymerization proceeds as descript in Scheme 1. The structures of PASS-2 were also characterized by NMR (Fig. 4), FT-IR spectra and elemental analysis.
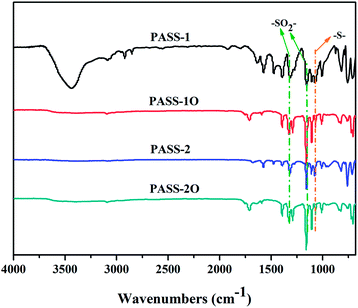 |
| Fig. 2 FT-IR spectra of PASS-1, PASS-1O (oxidation sample), PASS-2 and PASS-2O (oxidation sample). | |
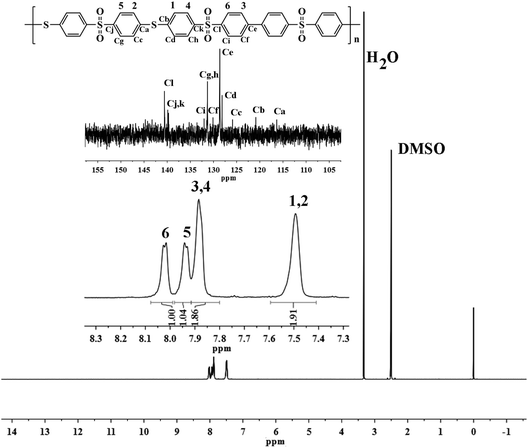 |
| Fig. 3 NMR spectrum of PASS-1. | |
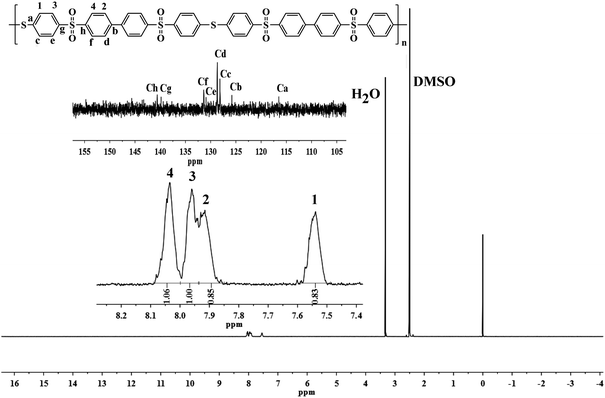 |
| Fig. 4 NMR spectrum of PASS-2. | |
Thermal properties of polymers
The thermal properties of polymers were evaluated by TGA and DSC measurements. The results of weight loss temperatures (T5%) and glass transition temperatures are summarized in Table 2. The glass transition temperature (Tgs) of the polymers was estimated by DSC. As shown in Fig. 5, the Tg of the resultant polymers were 263 and 282 °C, respectively. They were 40–60 °C higher than that of poly(phenylene sulfide sulfone) (Tg: 207–222 °C),32 polyether sulfone (Tg: ∼230 °C) and PVDF (Tg: ∼40 °C). Compared with PES and PVDF, they can be used in higher temperature environment. In particular, PASS-2 has about 19 °C higher Tg than that of PASS-1 because of the much more content rigid structure of biphenyl unit. Also from the DSC curves we had not find the endothermic melting peak of polymers. That suggests the amorphous nature of the polymers. As shown in Fig. 6, the initial degradation temperatures of PPSS, PASS-1 and PASS-2 in nitrogen (T5%) were 431, 441 and 445 °C, respectively. They provided about 40.8%, 42.6%, 43.9% char yield at 800 °C in nitrogen (Table 2). The TGA data indicated that these polymers had good thermo-stability. From Table 2 we found that PASS-2 had the highest thermal stability among these three kinds of polymers. The main reason was that PASS-2 had much higher content of sulfone and biphenyl units in the polymer main chain than that of PPSS and PASS-1. The thermal decomposition curves also revealed the degradation process of PPSS, PASS-1 and PASS-2 was one step.
Table 2 Thermal and tensile properties of PASSs
Polymers |
Tg (°C) |
T5% (°C) |
Char yield (%) |
Tensile strength (MPa) |
Young's modulus (GPa) |
Elongation at break (%) |
PPSS |
223 |
431 |
40.8 |
72 |
0.8 |
18 |
PASS-1 |
263 |
441 |
42.6 |
94 |
1.1 |
16 |
PASS-2 |
282 |
445 |
43.9 |
78 |
1.4 |
12 |
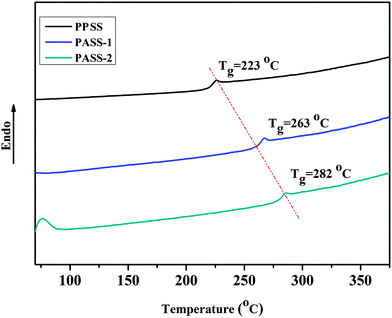 |
| Fig. 5 DSC curves of PPSS and PASSs at a heating rate of 10 °C per min in N2. | |
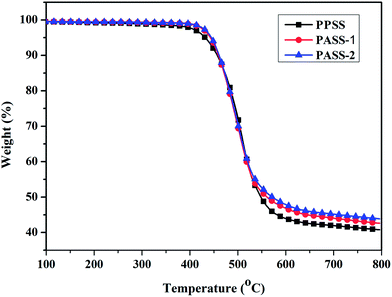 |
| Fig. 6 TGA curves of PPSS and PASSs at a heating rate of 10 °C per min in N2. | |
Tensile properties
The average tensile strengths of the casting films of polymers (treated at 130 °C for 10 h, then at 180 °C for 12 h) are given in Table 2. As shown in Table 2, the average tensile strengths and Young's modulus values of the films were 72–94 MPa and 0.8–1.4 GPa, respectively. It suggests the resultant polymers have good mechanical properties. While the elongation at break was more than 10%, suggesting the films have better toughness than that of earlier reported by our group.11
Oxidation of PASSs membranes
FT-IR analysis of oxidized PASSs. FT-IR analysis was used to study the chemical group changes of the oxidation reaction. As shown in Fig. 2, Comparing with the spectra of untreated PASS-1 membrane, the spectra of oxidized PASS-1 and PASS-2 membranes (PASS-1O and PASS-2O) showed significantly absorption increasement near 1320 cm−1 and 1150 cm−l (attributed to the vibration of sulfone group: –SO2–), at the same time we found the absorption decreasement near 1073 cm−l (attributed to the vibration of thioether unit: –S–). The FT-IR result suggests that the content of sulfone group has increased, while the thioether content has decreased with the oxidation reaction. It indicates that the thioether group on the membrane surface of PASS-1 has been converted to sulfone (–SO2–) units with the oxidation treatment.
XPS analysis of oxidized PASSs membrane surface. X-ray photoelectron spectroscopy (XPS) was used to characterize the valence and content changes of the chemical elements with the oxidation treatment. As shown in Fig. 7, un-oxidized PASSs exhibited two sulfur peaks, which suggested that the S atom in the polymer main chain had two kinds of valence states (–SO2–: ∼167.5 eV and –S–: 163.5 eV).32 We found that the sulfur peak near 163.5 eV attributed to thioether moiety almost disappeared after oxidation treatment, while the peak near 167.5 eV got more large than that of un-oxidized sample. We also fond a new peak near 165–166 eV with the oxidation reaction. It may be attributed to the little amount of sulfoxide (–SO–) group. It revealed the thioether was converted into sulfoxide and sulfone group with the oxidation process. As shown in Table 3, with the treatment of oxidation, the content of oxygen was raised from 18.81–16.16% to 22.82–18.20%. It also indicated the thioether was converted to sulfone unit with the treatment. That was agreed with the results of FT-IR analysis.
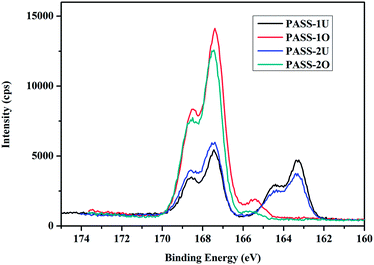 |
| Fig. 7 X-ray photoelectron spectroscopy (XPS) of sulfur in PASSs (unoxidized and oxidized). | |
Table 3 XPS surface elements contents (C, O, S) for the PASSs before (PASS-U) and after oxidation (PASS-O)
Element (%) |
C |
O |
S |
PASS-1U |
75.13 |
18.81 |
6.05 |
PASS-1O |
67.72 |
22.82 |
9.47 |
PASS-2U |
77.59 |
16.16 |
6.26 |
PASS-2O |
73.51 |
18.20 |
8.29 |
Properties of oxidized PASSs membranes
Corrosion resistance properties of oxidized PASSs membranes. The corrosion resistance of PASS-1U (untreated), PASS-1O (oxidized) PASS-2U (untreated) and PASS-2O (oxidized) are summarized in Table 4. Comparing with PASS-1U and PASS-2U, PASS-1O and PASS-2O showed a relatively excellent corrosion resistance. PASS-1U and PASS-2U dissolved in some strong polar solvents such as DMF, DMAC, NMP, concentrated sulfuric acid and so on. While PASS-1O and PASS-2O absolutely had no changes after keeping for about 6 months in NaOH (2 M), NMP, concentrated sulfuric acid and even aqua regia (as shown in Fig. 8). The main reason was that the thioether bonds in the polymer backbone were almost oxidized to sulfone groups35,36 (as described in Fig. 9) with the mixture of H2O2–CH3COOH–H2SO4 (small amount) under moderate condition. The aromatic ring planes lie essentially orthogonal to a plane defined by the bridging C–S–C groups in
, and the diaryl sulfone units adopt near-perfect open-book conformations and the molecules crystallize with the aromatic rings of laterally adjacent chains essentially parallel as shown in Fig. 9. This results was similar with the earlier reports about that the poly(1,4-phenylenesulfone) is highly crystalline and insoluble.37
Table 4 Corrosion resistance behavior of PASSs
Solvents |
Polymers |
PASS-1U |
PASS-1O |
PASS-2U |
PASS-2O |
++: soluble at room temperature; +: soluble at solvents boiling point; +−: swelling with heating; −: insoluble with heating. |
concentrated sulfuric acid |
++a |
− |
++ |
− |
Formic acid |
− |
− |
− |
− |
NMP |
++ |
− |
++ |
− |
DMF |
++ |
− |
++ |
− |
DMAC |
++ |
− |
++ |
− |
Pyridine |
+− |
− |
− |
− |
Acetone |
− |
− |
− |
− |
Chloroform |
+− |
− |
+− |
− |
DMSO |
++ |
− |
+ |
− |
1,4-Dioxane |
− |
− |
− |
− |
Toluene |
− |
− |
− |
− |
Cyclohexanone |
− |
− |
− |
− |
Phenol + tetrachloroethane |
++ |
− |
++ |
− |
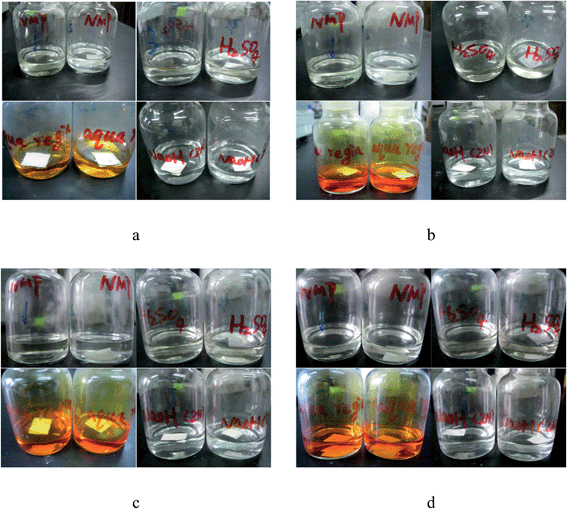 |
| Fig. 8 The images of PASS-1 membrane (unoxidized-the left sample, oxidized-the right sample) kept for different time (a. 5 min, b. 24 h, c. 15 days, d. 180 days) in solvents (NMP, concentrated sulfuric acid, aqua regia, NaOH). | |
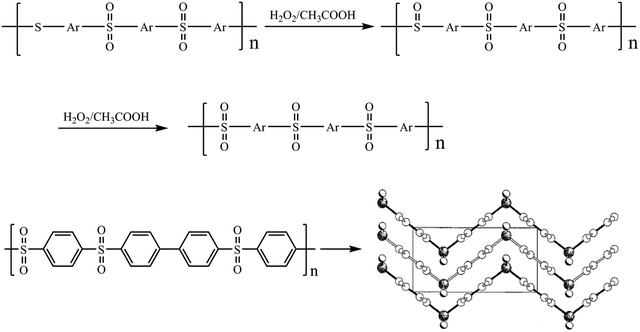 |
| Fig. 9 The oxidation reaction mechanism and three-dimensional diagrammatic sketch. | |
Flux and permeation of oxidized PASSs membranes. The flux of PASSs was tested with deionized water in a dead-end ultrafiltration (UF) cell under 0.1 MPa. Each membrane was initially pre-pressurized with deionized water for 2 h at 0.2 MPa. As shown in Fig. 10, The flux of PASS-1 and PASS-2 were 0.61–2.02 and 0.74–2.48 L m−2, respectively. We found that the flux value decreased with the resin concentration increased. It was consistent with the regularity of the water flux of separation membrane. Also we found the pure PASSs had minor flux than commercial products such as PVDF, PES and so on, we will improve its water flux property next. Interestingly, we found that the flux of PASS-1 and PASS-2 became more large than that of untreated samples (PASS-1O: 1.06–2.52 L m−2, PASS-2O: 1.25–3.56 L m−2) after oxidation treatment. Whereas the retention rate (bovine serum albumin) decreased a little in a reasonable range as shown in Fig. 11. In order to investigate this phenomenon, the SEM and AFM was carried to observe the section and surface of the membranes. As shown in Fig. 12, the oxidized and un-oxidized membranes exhibited similar asymmetric structure: a dense top layer, a porous finger-like and sponge-like sub layer. From the AFM images (Fig. 13), we found that the microporous on the oxidized membranes surface became much more obvious than that of un-oxidized samples. The main reason is that the polymer chain is tend to appear local order structure with the oxidation treatment as demonstrated in Fig. 9. The surface of the membrane begun to shrink, then the microporous structure got more and more clear. We also further studied the changes of flux and retention rate before and after oxidization with the contact angle experiment. As shown in Fig. 14, the contact angle became smaller with the oxidation treatment. It suggested the hydrophilicity of the membrane got better after oxidation treatment. And that was beneficial for the contamination resistance of the membranes. It could be concluded that both the surface structure and hydrophilicity of the membrane before and after oxidation affected the flux and retention rate results.
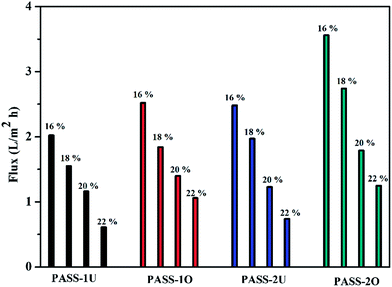 |
| Fig. 10 Flux of PASSs membrane before and after oxidation. | |
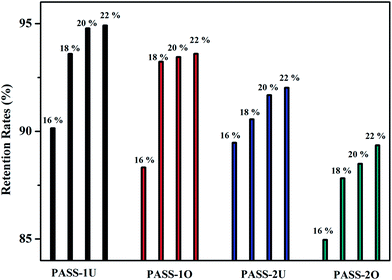 |
| Fig. 11 Retention rate (bovine serum albumin) of PASSs membrane before and after oxidation. | |
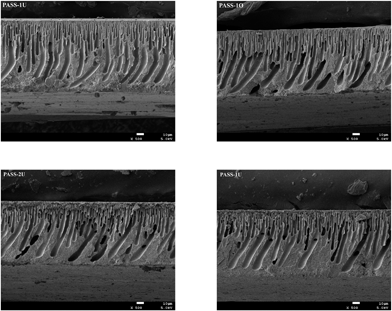 |
| Fig. 12 SEM images of cross-section of PASSs membrane (18 wt%) before and after oxidation. | |
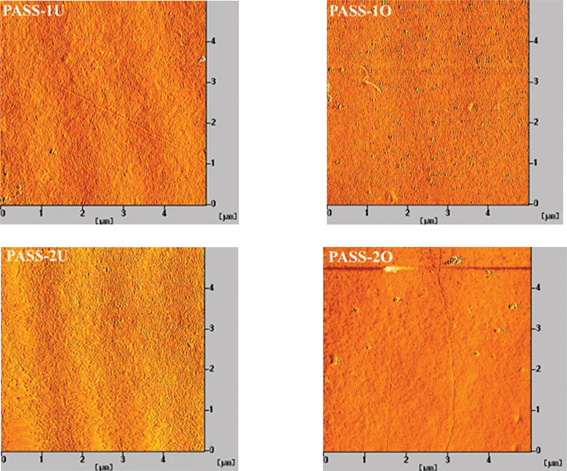 |
| Fig. 13 AFM images of surface of PASSs membrane before and after oxidation. | |
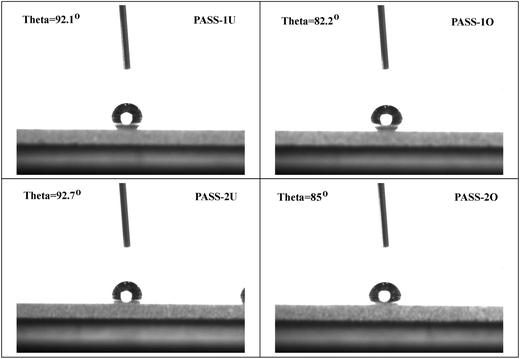 |
| Fig. 14 Contact angle of PASSs membrane (18 wt%) before and after oxidation. | |
Conclusions
Two kinds of aromatic dithiol (BMPSB and DMDPS) monomers containing sulfone unit and high sulfur content were synthesized in one step. They were conducted to react with BCPSB by nucleophilic polycondensation to prepare poly(arylene ether sulfone)s containing thioether units (PASSs) with higher glass transition temperature and excellent thermal stability than that of commercial products such as PVDF and PES. The resultant polymers can dissolve in strong polar solvents such as NMP and supply a separation membrane with flux of 0.61–2.48 L m−2 h−1, retention rate of 88.32–94.91%. Interestingly, we found that the thioether in the polymer main chain can be oxidized with a moderate condition. The corrosion resistance of these membranes after oxidation is largely improved. The oxidized samples are even not insoluble in concentrated sulfuric acid, NMP, aqua regia and so on. This approach provides a convenient method for preparation of chemically and thermally stable poly(arylene ether sulfone)s polymers with excellent corrosion resistance. Thus, the PASSs can be good candidates as membrane materials for industrial filtration especially in some harsh environments (high operating temperature and corrosion condition), although the pure water flux need improvement.
Acknowledgements
This work was supported by research grants from the Youth Fund Natural Science Foundation of China (21304060) and Youth Fund of Sichuan University (2012SCU11009).
References
- M. E. Rogers and T. E. Long, Synthetic Methods in Step-Growth Polymers, Wiley-Interscience, New York, 2003, pp. 327–375 Search PubMed.
- J. B. Rose, Polymer, 1974, 15, 456–465 CrossRef CAS.
- B. Zhang, S. Justin, Y. Chen, T. Richard and W. Sungsool, J. Phys. Chem. B, 2012, 116, 7970–7980 CrossRef CAS PubMed.
- G. P. Yu, C. Liu, H. X. Zhou, J. Y. Wang, E. C. Lin and X. G. Jian, Polymer, 2009, 50, 4520–4528 CrossRef CAS PubMed.
- D. M. Tigelaar, E. Palkera, C. M. Jackson, K. M. Anderson, J. S. Wainrigh and F. Robert, Macromolecules, 2009, 42, 1888–1896 CrossRef CAS.
- M. Kumar and M. Ulbricht, RSC Adv., 2013, 3, 12190–12203 RSC.
- B. Zhang and S. T. Richard, Polymer, 2013, 54, 4493–4500 CrossRef CAS PubMed.
- Y. Liu, S. L. Zhang and G. B. Wang, Desalination, 2013, 316, 127–136 CrossRef CAS PubMed.
- Z. G. Wang, T. L. Chen and J. P. Xu, Macromolecules, 2001, 34, 9015–9022 CrossRef CAS.
- Q. F. Zhang, S. B. Zhang, L. Dai and X. S. Chen, J. Membr. Sci., 2010, 349, 217–224 CrossRef CAS PubMed.
- G. Zhang, H. H. Ren, D. S. Li, S. R. Long and J. Yang, Polymer, 2013, 54, 601–606 CrossRef CAS PubMed.
- G. M. Geise, D. R. Paul and B. D. Freeman, Prog. Polym. Sci., 2014, 39, 1–42 CrossRef CAS PubMed.
- G. M. Geise, H. S. Lee, D. J. Miller, B. D. Freeman, J. E. McGrath and D. R. Paul, J. Polym. Sci., Part B: Polym. Phys., 2010, 48, 1685–1718 CrossRef CAS.
- B. S. Qian, J. Li, Q. Wei, P. L. Bai, B. H. Fang and C. S. Zhao, J. Membr. Sci., 2009, 344, 297–303 CrossRef CAS PubMed.
- D. S. Wang, W. Zou, L. L. Li, Q. Wei, S. D. Sun and C. S. Zhao, J. Membr. Sci., 2011, 374, 93–101 CrossRef CAS PubMed.
- D. S. Wang, X. X. Zhang, S. Q. Nie, W. F. Zhao, Y. Lu, S. D. Sun and C. S. Zhao, Langmuir, 2012, 28, 13284–13293 CrossRef CAS PubMed.
- Z. Li, J. F. Ding, G. P. Robertson and M. D. Guiver, Macromolecules, 2006, 39, 6990–6996 CrossRef CAS.
- Y. Dai, X. G. Jian, S. H. Zhang and M. D. Guiver, J. Membr. Sci., 2001, 188, 195–203 CrossRef CAS.
- N. Y. Du, G. P. Robertson, I. Pinnau and M. D. Guiver, Macromolecules, 2009, 42, 6023–6030 CrossRef CAS.
- M. Paul, H. B. Park, B. D. Freeman, A. Roy, J. E. McGrath and J. S. Riffle, Polymer, 2008, 49, 2243–2252 CrossRef CAS PubMed.
- W. Xie, H. Ju, G. M. Geise, B. D. Freeman, J. I. Mardel, A. J. Hill and J. E. McGrath, Macromolecules, 2011, 44, 4428–4438 CrossRef CAS.
- W. Xie, J. Cook, H. B. Park, B. D. Freeman, C. H. Lee and J. E. McGrath, Polymer, 2011, 52, 2032–2043 CrossRef CAS PubMed.
- Y. Song, J. Y. Wang, G. H. Li, Q. M. Sun, X. G. Jian, J. Teng and H. B. Zhang, Polymer, 2008, 49, 724–731 CrossRef CAS PubMed.
- G. P. Yu, C. Liu, B. Li, L. W. Wang, J. Y. Wang and X. G. Jian, J. Polym. Res., 2012 DOI:10.1007/s10965-012-9829-1.
- C. Yan, S. H. Zhang, C. Liu, D. L. Yang, F. J. Yang and X. G. Jian, J. Appl. Polym. Sci., 2009, 113, 1389–1397 CrossRef CAS.
- D. W. Shin, S. Y. Lee, N. R. Kang, K. H. Lee, M. D. Guiver and Y. M. Lee, Macromolecules, 2013, 46, 3452–3460 CrossRef CAS.
- F. A. Bottino, A. R. Cinquegrani, G. D. Pasquale, L. Lucrezia and A. Pollicino, Eur. Polym. J., 2003, 39, 2203–2208 CrossRef CAS.
- J. Park, J. Kim, M. Seo, J. Lee and S. Y. Kim, Chem. Commun., 2012, 48, 10556–10558 RSC.
- M. Barikani and S. Mehdipour-Ataei, J. Polym. Sci., Part A-1: Polym. Chem., 2000, 38, 1487–1492 CrossRef CAS.
- G. Zhang, J. Liu, M. L. Zhang, S. L. Liu, S. R. Long and J. Yang, J. Macromol. Sci., Part A: Pure Appl. Chem., 2009, 46, 1015–1023 CrossRef CAS.
- S. X. Xu, J. Yang, S. R. Long, Y. R. Chen and G. X. Li, Polym. Bull., 2005, 54, 251–261 CrossRef CAS PubMed.
- Y. Liu, A. Bhatnagar, Q. Ji, J. S. Riffile, J. E. McGrath, J. F. Geibei and T. Kashiwagi, Polymer, 2000, 41, 5137–5146 CrossRef CAS.
- H. D. Wang, J. Yang, S. R. Long, X. J. Wang, Z. Yang and G. X. Li, Polym. Degrad. Stab., 2004, 83, 229–235 CrossRef CAS.
- J. Yang, H. D. Wang, S. X. Xu, G. X. Li and Y. J. Huang, J. Polym. Res., 2005, 12, 317–323 CrossRef CAS.
- R. Noyori, M. Aoki and K. Sato, Chem. Commun., 2003, 39, 1977–1986 RSC.
- M. Schuster, K. D. Kreuer, H. T. Andersen and J. Maier, Macromolecules, 2007, 40, 598–607 CrossRef CAS.
- O. M. Colquhoun, P. L. Aldred, F. H. Kohnke, P. L. Herbertson, I. Baxter and D. J. Williams, Macromolecules, 2002, 35, 1685–1690 CrossRef.
|
This journal is © The Royal Society of Chemistry 2014 |
Click here to see how this site uses Cookies. View our privacy policy here.