DOI:
10.1039/C4RA02776K
(Paper)
RSC Adv., 2014,
4, 22463-22469
A single colorimetric sensor for multiple target ions: the simultaneous detection of Fe2+ and Cu2+ in aqueous media†
Received
29th March 2014
, Accepted 12th May 2014
First published on 13th May 2014
Abstract
This study demonstrates the design, synthesis and sensing properties of a simple and efficient chemosensor 1 (1 = 2,6-bis((2-(((pyridine-2-yl)methylamino)methyl)phenol)ethylamido)pyridine) to rapidly detect Fe2+ and Cu2+ in aqueous solution (bis-tris buffer/DMF (8/2, v/v)), exploiting UV-vis spectral analysis, naked-eye and paper devices. The sensor 1 showed significant absorption spectra at 455 nm for Fe2+ and 660 nm for Cu2+, which are responsible for color changes due to the metal-to-ligand charge-transfer. The binding modes of 1 with Fe2+ or Cu2+ have been investigated by Job plot and ESI-mass analysis. In addition, the sensor 1 could be recyclable simply through treatment with a proper reagent such as EDTA. Moreover, the sensor has been used in the development of practically viable colorimetric kits.
Introduction
Biologically relevant metal cations play crucial roles in many physiological and pathological processes.1,2 Therefore, the development of chemosensors for the detection of the metal cations is a vibrant area of investigation due to the potential applications of these chemosensors as diagnostic tools in medical, physiological and environmental applications.3 Among metal cations, iron and copper ions have bio-importance and are the first and third most abundant transition elements, respectively, in human body.
In well-nourished people the total iron content is ∼4 g (70% in Hgb, 25% in storage).4 In proportion to the amounts of iron in human body, iron is the most essential transition element responsible for carrying the oxygen in heme and acts as cofactor in enzymatic reactions of mitochondrial respiratory chain.5–8 However, iron deficiency leads to anemia, liver and kidney damages, diabetes, and heart diseases.9–13 Accretion of iron in the central nervous system has been involved in a number of diseases such as Parkinson's, Huntington's and Alzheimer's disease, associated with an increased quantity of iron.14–19 In addition, two oxidation states (Fe2+ and Fe3+) of iron are one of the important redox pairs in biological systems.
Copper plays an important role as a redox catalyst in biological processes such as electron transfer reactions involving oxidation of various organic substrates.20–31 The adult human body contains 1.4–2.1 mg of copper per kilogram of body weight under normal conditions.30 At high concentrations, copper becomes toxic and causes oxidative stress and disorders associated with neurodegenerative diseases including Alzheimer's, Parkins's, Menke's, Wilson's and prion diseases.31–34 The World Health Organization (WHO) has recommended the maximum limit of copper in drinking water to be at 2 ppm (30 μM).20 In view of the significance of iron and copper ions, therefore, it is very necessary to efficiently monitor the presence of iron and copper metal ions. As a result, the development of simple and rapid sensors for iron and copper ions has attracted wide research interest. The most attractive approach for iron and copper detection is a colorimetric method, because they can be easily observed and determined by eye, rather than by using large, sophisticated, and expensive analytical instruments.35–43 Therefore, there is a strong demand for new colorimetric chemosensors that can achieve naked-eye detection in the visible wavelength region without the requirement of equipment.43–45 In addition, paper-based assays using the colorimetric method are attractive for portable point-of-measurement (POM) monitoring and on-site detection due to advantages which include low cost, portability, ease of use, high speed, and low reagent and sample consumption.46,47
Recently, we demonstrated that the chemosensor obtained from the displacement of dipicolylamine by PAP (2-(((pyridin-3-yl)methylamino)methyl)phenol) showed a change of the sensing property from a fluorogenic sensing for Zn2+ to a colorimetric sensing for Co2+ ions.48 These results led us to design a new chemosensor that two dipicolylamines attached to the skeleton of the pyridyl amide49 are displaced with the two PAP groups (Scheme 1). To this end, we synthesized the new chemosensor 1 and tested its sensing properties to a variety of metal ions. Surprisingly, the 1 showed colorimetrically the simultaneous detection of Fe2+ and Cu2+.
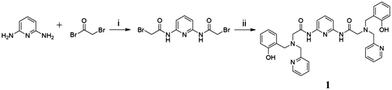 |
| Scheme 1 Synthesis of 1. (i) pyridine, 1 h. (ii) PAP, TEA, 4 h. | |
Herein, we report on the development of a colorimetric sensor based on PAP platform for rapid determination of Fe2+ and Cu2+ in aqueous solution (bis-tris buffer/DMF = 8/2, v/v, pH 7.0). The solution colors of 1 with Fe2+ and Cu2+ changed from colorless to light orange and to green, respectively. To our best knowledge, it is the second report that the simultaneous detection of Fe2+ and Cu2+ could be carried out calorimetrically by 1 in aqueous solution.11 In particular, it is very valuable to detect Fe2+ and Cu2+ with naked-eye, because Fe2+ and Cu2+ are well known fluorescent quenchers due to their paramagnetic nature which makes it practically difficult to develop fluorescent sensors for their detection.
Experimental section
General information
All the solvents and reagents (analytical grade and spectroscopic grade) were obtained commercially and used as received. 2,6-Bis(chloroethylamido)pyridine49 and 2-(((pyridin-3-yl)methylamino)methyl)phenol50 were prepared according to the procedure reported in the literature. 1H and 13C NMR spectra were recorded on a Varian 400 MHz spectrometer and chemical shifts are recorded in ppm. Absorption spectra were recorded at room temperature using a Perkin Elmer model Lambda 2S UV/Vis spectrometer. Electrospray ionization mass spectra (ESI-MS) were collected on a Thermo Finnigan (San Jose, CA, USA) LCQTM Advantage MAX quadrupole ion trap instrument. Elemental analysis for carbon, nitrogen, and hydrogen was carried out using a Flash EA 1112 elemental analyzer (thermo) at the Organic Chemistry Research Center of Sogang University, Korea.
Synthesis of receptor 1
2,6-Bis(chloroethylamido)pyridine (0.7370 g, 2.1 mmol), 2-(((pyridin-3-yl)methylamino)methyl)phenol (0.8570 g, 4 mmol) and triethylamine (588 μL, 4.2 mmol) were dissolved in acetonitrile (30 mL). After the reaction solution was stirred for 4 h at room temperature, the liquid was removed under reduced pressure to obtain brown oil, which was purified by silica gel column chromatography (1/8, v/v, THF–EA). Yield: 0.78 g (63.5%). 1H NMR (DMSO-d6, 400 MHz): δ 10.32 (s, 2H), 10.02 (s, 2H), 7.77 (m, 5H), 7.41 (d, 2H), 7.26 (t, 2H), 7.21 (d, 2H), 7.12 (t, 2H), 6.82 (d, 2H), 6.76 (t, 2H), 3.91 (s, 4H), 3.81 (s, 4H), 3.43 (s, 4H); HRMS (ESI) m/z calcd for C35H35N7O4 + H+: 618.28 [M + H]+; found: 618.13; anal. calcd for C35H35N7O4: C, 68.06; H, 5.71; N, 15.87. Found: C, 67.69; H, 5.85; N, 16.17.
UV-vis titrations
Dissolved in DMF (2 mL) and 20 μL of the receptor 1 (3 mM) were diluted with 2.980 mL bis-tris buffer/DMF (8/2) to make the final concentration of 20 μM. Fe(ClO4)2 (20.79 mg, 0.08 mmol) was dissolved in bis-tris buffer/DMF (8/2, 4 mL) and 0.6–6 μL of the Fe2+ solution (20 mM) were transferred to receptor 1 solution (20 μM) prepared above. After mixing them for a few second, UV-vis spectra were taken at room temperature.
For Cu2+ ion; receptor 1 (3.7 mg, 0.006 mmol) was dissolved in DMF (2 mL) and 20 μL of the receptor 1 (3 mM) were diluted with 2.980 mL bis-tris buffer/DMF (8/2) to make the final concentration of 20 μM. Cu(NO3)2 (18.9 mg, 0.08 mmol) was dissolved in bis-tris buffer/DMF (8/2, 4 mL) and 0.6–6 μL of the Cu2+ solution (20 mM) were transferred to receptor 1 solution (20 μM) prepared above. After mixing them for a few second, UV-vis spectra were taken at room temperature.
Job plot measurements
For Fe2+ ion; receptor 1 (1.48 mg, 0.0024 mmol) was dissolved in DMF (40 mL). 5, 4.5, 4, 3.5, 3, 2.5, 2, 1.5, 1 and 0.5 mL of the receptor 1 solution were taken and transferred to vials. Fe(ClO4)2 (0.98 mg, 0.0024 mmol) was dissolved in bis-tris buffer/DMF (8/2, 4 mL). 0.5, 1, 1.5, 2, 2.5, 3, 3.5, 4, 4.5 and 5 mL of the Fe2+ ion were taken and transferred to each receptor 1 solution prepared above. Each vial had a total volume of 3 mL. After shaking the vials for a few minutes, UV-vis spectra were taken at room temperature.
For Cu2+ ion; receptor 1 (1.48 mg, 0.0024 mmol) was dissolved in DMF (40 mL). 5, 4.5, 4, 3.5, 3, 2.5, 2, 1.5, 1, and 0.5 mL of the receptor 1 solution were taken and transferred to vials. Cu(NO3)2 (0.98 mg, 0.0024 mmol) was dissolved in bis-tris buffer/DMF (8/2, 4 mL). 0.5, 1, 1.5, 2, 2.5, 3, 3.5, 4, 4.5 and 5 mL of the Cu2+ ion were taken and transferred to each receptor 1 solution prepared above. Each vial had a total volume of 3 mL. After shaking the vials for a few minutes, UV-vis spectra were taken at room temperature.
Competition with other metal ions
For Fe2+ ion; receptor 1 (3.7 mg, 0.006 mmol) was dissolved in DMF (1 mL) and 20 μL of the receptor 1 (3 mM) were diluted with 2.980 mL bis-tris buffer/DMF (8/2) to make the final concentration of 20 μM. MNO3 (M = Na, K, 0.08 mmol) or M(NO3)2 (M = Mg, Ca, Mn, Co, Ni, Cu, Zn, Cd, Pb, 0.08 mmol) or M(NO3)3 (M = Al, Cr, Fe 0.08 mmol) or M(ClO4)2 (M = Fe, 0.08 mmol) were dissolved in bis-tris buffer/DMF (8/2, 4 mL), respectively. 6 μL of each metal solution (20 mM) were taken and added into 3 mL of each receptor 1 solution (20 μM) prepared above to make 2 equiv. Then, 6 μL of the Fe2+ solution (20 mM) were added into the mixed solution of each metal ion and receptor 1 to make 2 equiv. After mixing them for a few second, UV-vis spectra were taken at room temperature.
For Cu2+ ion; receptor 1 (3.7 mg, 0.006 mmol) was dissolved in DMF (1 mL) and 20 μL of the receptor 1 (3 mM) were diluted with 2.980 mL bis-tris buffer/DMF (8/2) to make the final concentration of 20 μM. MNO3 (M = Na, K, 0.08 mmol) or M(NO3)2 (M = Mg, Ca, Mn, Co, Ni, Cu, Zn, Cd, Pb, 0.08 mmol) or M(NO3)3 (M = Al, Cr, Fe 0.08 mmol) or M(ClO4)2 (M = Fe, 0.08 mmol) were dissolved in bis-tris buffer/DMF (8/2, 4 mL), respectively. 6 μL of each metal solution (20 mM) were taken and added into 3 mL of each receptor 1 solution (20 μM) prepared above to make 2 equiv. Then, 6 μL of the Cu2+ solution (20 mM) were added into the mixed solution of each metal ion and receptor 1 to make 2 equiv. After mixing them for a few second, UV-vis spectra were taken at room temperature.
Results and discussion
Synthesis and characterization of receptor 1
The receptor 1 was prepared through a straightforward synthetic route. 2,6-Bis(chloroethylamido)pyridine was treated with 2-(((pyridin-3-yl)methylamino)methyl)phenol in acetonitrile (Scheme 1). The synthesized receptor 1 was characterized by 1H and 13C NMR, ESI-mass spectrometry analysis, and elemental analysis.
Colorimetric signaling of Fe2+ and Cu2+ ions
The cation binding properties of 1 were observed by employing aqueous solution (bis-tris buffer/DMF = 8/2, v/v, pH 7.0) of different cations (Na+, Mg2+, Al3+, K+, Ca2+, Cr3+, Mn2+, Fe2+, Fe3+, Co2+, Ni2+, Cu2+, Zn2+, Cd2+ and Pb2+). The receptor 1 did not exhibit any absorption band in visible region, as shown in Fig. 1(a). The addition of Fe2+ and Cu2+ into 1 showed the spectral changes, which were accompanied with visual color change from colorless to light orange and green, respectively (Fig. 1(b)). The presence of other metal ions (Na+, Mg2+, Al3+, K+, Ca2+, Cr3+, Mn2+, Fe3+, Co2+, Ni2+, Zn2+, Cd2+ and Pb2+) showed no change in the absorption spectrum relative to the free receptor except for Fe3+. The addition of Fe3+ into 1 also showed the change of color, but it was proved that the pale yellow color was due to the color of Fe3+ itself (Fig. S1†). In particular, these results are very valuable, because Fe2+ and Cu2+ are well known fluorescent quenchers due to their paramagnetic nature which makes it practically difficult to develop fluorescent sensors for their detection.
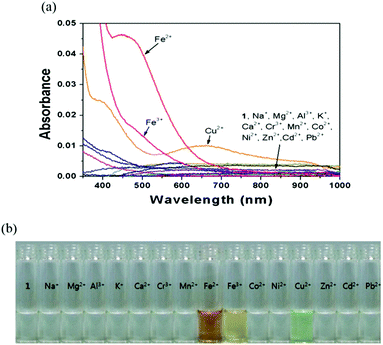 |
| Fig. 1 (a) UV-vis spectra of 1 (20 μM) in the presence of various metal ions (60 μM) in bis-tris buffer/DMF (8/2, v/v, pH 7.0). (b) Color changes of 1 in the presence of various metal ions. | |
To further elicit the recognition behaviors of 1 towards Fe2+ and Cu2+, UV-vis titrations of 1 with Fe2+ and Cu2+ were measured, respectively. With an increasing amount of Fe2+, the absorbance at 455 nm gradually increased (Fig. 2). The molar extinction coefficient of this band is ε = 3.2 × 103 M−1 cm−1 which is too large to be Fe-based d–d transitions and thus must be metal-based transitions. These results indicate that a colorimetric method could be used for Fe2+ assay. To determine the stoichiometry of the 1–Fe2+ complex, Job method for the absorbance was applied (Fig. 3).51 It showed that the absorbance exhibited a maximum at a molar fraction of 0.7, indicating a 2
:
1 stoichiometry of Fe2+ to 1 in the complex. This was further confirmed by the ESI-mass analysis (Fig. S2†). The [1 + 2Fe + H2O]2+ complex was calculated to be m/z 372.57 and measured to be m/z 372.80. Based on the Job plot and ESI-mass spectrometry analysis, we propose the structure of a 2
:
1 complex of Fe2+ and 1 as shown in Scheme 2. We presume that each iron in the proposed structure would have penta-coordinate environment with a solvent or ClO4−, because iron(II) ion usually forms penta- or hexa-coordinate complexes. From the 2
:
1 binding mode, the association constant (log
K) was calculated to be 9.22 on the basis of Li's equation (Fig. S3†).
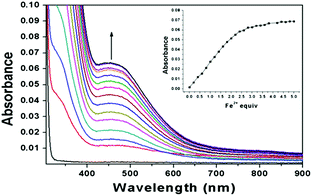 |
| Fig. 2 UV-vis spectral titration of 1 (20 μM) with Fe2+ (0–60 μM) in bis-tris buffer/DMF (8/2, v/v). Inset: absorbance of 1 at 455 nm as a function of equiv. of Fe2+. | |
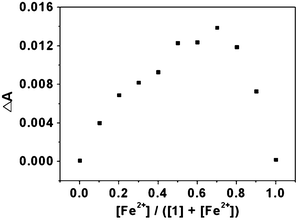 |
| Fig. 3 Job plot of Fe2+ complex formation. The total concentration of 1 with Fe2+ was 40 μM. | |
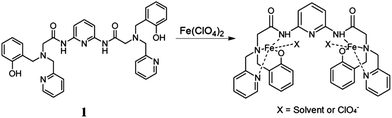 |
| Scheme 2 Proposed structure of a 1 : 2 complex of 1 with Fe2+. | |
This value is within the range of those (5.17–17) reported for Fe2+-binding chemosensors.4,52–54 The absorption titration profile of 1 with Fe2+ demonstrated that the detection limit of Fe2+ is 2.94 μM on the basis of 3σ/K (Fig. S4†).55 According to the drinking water standards and health advisories proposed by U.S. Environmental Protection Agency (EPA), the safety amount of iron is limited to 0.3 mg L−1 (5.36 μM).56 Hence, the receptor 1 could monitor trace of Fe2+ in industrial, environmental and biological analysis.
UV-vis titration of 1 with Cu2+ showed two absorption bands at 400 nm (ε = 1.3 × 103 M−1 cm−1) and 660 nm (ε = 0.5 × 103 M−1 cm−1), which might be due to a metal-to-ligand charge-transfer (MLCT) process (Fig. 4). The MLCT is responsible for the change of color from colorless to green, which is perceptible to the naked-eye. The stoichiometry of complexation of 1 and Cu2+ was studied using Job plot, which showed 2
:
1 ratio of Cu2+ and 1 (Fig. S5†). The stoichiometry of Cu2+ with 1 was also supported by ESI-mass spectrometry analysis (Fig. 5). The [1 + 2Cu]2+ complex was calculated to be m/z 740.11 and measured to be m/z 740.00. Based on the Job plot and ESI-mass spectrometry analysis, we propose the structure of a 2
:
1 complex of Cu2+ and 1 as shown in Scheme 3. We presume that each copper in the proposed structure would have tetra- or penta-coordinate environment without/with a NO3−, because copper(II) ion prefers to form tetra- or penta-coordinate complexes. From the 2
:
1 binding mode, the association constant (log
K) of 1–Cu2+ complex was calculated to be 9.22 from Li's equation (Fig. S6†). The detection limit of receptor 1 for the analysis of Cu2+ was calculated to be 2.29 μM (Fig. S7†), which is far below the WHO guideline (30 μM), making it capable of being a practical system for the monitoring of Cu2+ concentrations in aqueous samples.
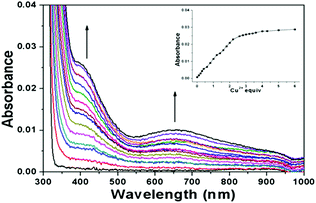 |
| Fig. 4 UV-vis spectral titration of 1 (20 μM) with Cu2+ (0–60 μM) in bis-tris buffer/DMF (8/2, v/v). Inset: absorbance of 1 at 400 nm as a function of equiv. of Cu2+. | |
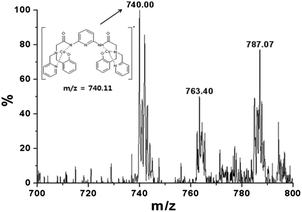 |
| Fig. 5 Positive-ion electrospray ionization mass spectrum of 1 (1.0 × 10−4 M) upon addition of 2 equiv. of Cu2+. | |
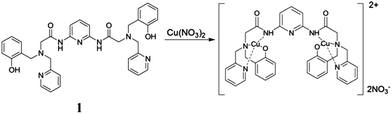 |
| Scheme 3 Proposed structure of a 1 : 2 complex of 1 with Cu2+. | |
The competitive binding studies
We studied the preferential selectivity of 1 as a colorimetric chemosensor for the detection of Fe2+ and Cu2+ in the presence of various competing metal ions. The receptor 1 was treated with 3 equiv. of Fe2+ in the presence of various metal ions such as Na+, Mg2+, Al3+, K+, Ca2+, Cr3+, Mn2+, Fe3+, Co2+, Ni2+, Cu2+, Zn2+, Cd2+ and Pb2+. As shown in Fig. 6(a), the presence of other background metal ions showed no or a little change of absorbance at 455 nm. Therefore, the receptor 1 showed an exclusive selectivity toward the detection of Fe2+ in the presence of various other metal ions. The change of absorption intensity of 1 was also measured in the presence of Cu2+ mixed with various metal ions. Compared to the absorption intensity obtained with Cu2+ alone (Fig. 6(b)), the absorbance showed no or a little change in the presence of Na+, Mg2+, Al3+, K+, Ca2+, Cr3+, Mn2+, Fe2+, Fe3+, Co2+, Ni2+, Zn2+, Cd2+ and Pb2+.
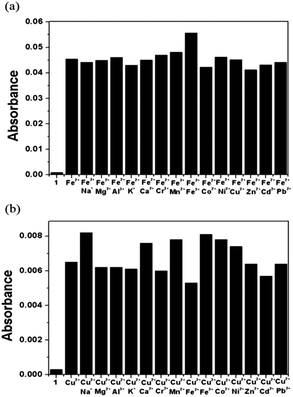 |
| Fig. 6 Effect of competitive metal ions (60 μM) on the interaction between receptor 1 (20 μM) and (a) Fe2+ (60 μM), and (b) Cu2+ (60 μM), respectively, in bis-tris buffer/DMF (8/2, v/v). | |
On the other hand, it is important to figure out how the color of 1 solution changes in the presence of both Fe2+ and Cu2+, because both of them showed the same binding constants toward 1. Therefore, we examined the color of 1 solution in the presence of blended Fe2+ and Cu2+, and observed that the receptor 1 showed the color change from colorless to yellow which is a mixed color of orange (for Fe2+) and green (for Cu2+) (Fig. S8†). These results indicate that one could also detect the presence of both Fe2+ and Cu2+ by the color change of 1 under the special conditions.
The effects of pH and EDTA
We investigated the effect of pH on the absorption response of receptor 1 to Fe2+ and Cu2+, respectively, in a series of buffers with pH values ranging from 2 to 11 (Fig. 7). The pH studies revealed that the receptor 1 itself did not undergo any significant absorbance enhancement within the pH range from 2–11, which suggested that it was stable over the pH range. The absorption of the 1–Fe2+ complex was very weak at low pH, whereas, at high pH, the complex showed a significant response between pH 6 and 11, which includes the biologically relevant range of pH 6.0–7.6. The absorbance of the 1–Cu2+ complex also displayed pH dependence over pH 2–11.
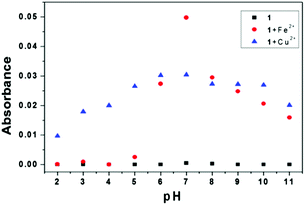 |
| Fig. 7 Effect of pH on the absorbance of 1 at 455 nm (circle for Fe2+) and 400 nm (triangle for Cu2+), respectively, in bis-tris buffer/DMF (8/2, v/v). | |
To validate the reversibility of complexation of 1 with Fe2+ and Cu2+, respectively, EDTA-addition experiments were performed. When EDTA was added to the solution of the 1–Fe2+ complex, the absorption intensity at 445 nm decreased (Fig. 8(a)). This is attributed to the stronger complexation of Fe2+ with EDTA than with 1. Further addition of excess Fe2+ recovered the spectrum and color of the solution (Fig. 8). This process could be repeated several times. The 1–Cu2+ complex also showed the same reversibility with EDTA (Fig. S9(a) and (b)†). The absorbance of the 1–Cu2+ complex at 400 nm decreased by addition of EDTA. Upon addition of Cu2+ to the system again, the spectrum of 1–Cu2+ complex was recovered to the original one accompanied by a color change from colorless to green. The absorbance at 740 nm is due to the coordination of Cu2+–EDTA, as shown in Fig. S9(c).†
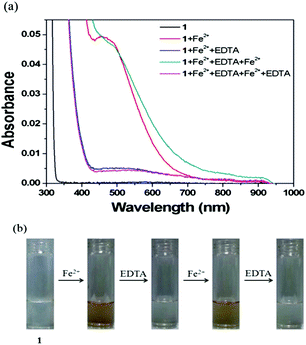 |
| Fig. 8 (a) Reversible changes in absorbance of 1 after the sequential addition of Fe2+ and EDTA in bis-tris buffer/DMF (8/2, v/v). (b) Reversible color changes of 1 after the sequential addition of Fe2+ and EDTA in bis-tris buffer/DMF (8/2, v/v). | |
These results indicate that receptor 1 could be recyclable simply through treatment with a proper reagent such as EDTA.
Detection of Fe2+ and Cu2+ on the paper devices
Most of sensors for detection of Fe2+ and Cu2+ operate only in the solution phase, and thus restrict their practical applications.7 Therefore, we have developed a colorimetric test kit, which was prepared by immersing filter papers into aqueous medium (bis-tris buffer/DMF = 8/2) of 1. The aqueous solutions of different metal ions were sprayed onto these strips. The receptor showed marked orange and green color only in the presence of Fe2+ and Cu2+, respectively, as shown in Fig. 9. Fe3+ exhibited its own color yellow, as already shown in Fig. S1.† These results indicate that receptor 1 could be a convenient sensor for Fe2+ and Cu2+ in environmental analyses.
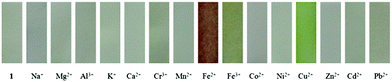 |
| Fig. 9 Colorimetric test kits. Photographs of various cations sprayed on the filter paper coated with 1. | |
Conclusion
We have developed a novel colorimetric chemosensor 1 for Fe2+ and Cu2+ based on PAP platform in aqueous solution. The sensor 1 showed unique color changes upon the binding with Fe2+ or Cu2+ in aqueous solution (bis-tris buffer/DMF = 8/2). In addition, the sensor showed good sensitivities with μM-level detection limits, and displayed high selectivity to Fe2+ or Cu2+ in the presence of other common interference cations. Importantly, the Fe2+ and Cu2+-induced chromogenic processes could be reversible by addition of EDTA. Moreover, the easy-to-prepare test kit provided a convenient and reliable detection of Fe2+ and Cu2+ ions in practical applications. Therefore, the results reported here provide a novel approach for the simultaneous colorimetric recognition of the two most abundant transition metal ions (Fe2+ and Cu2+) among the various metal ions.
Acknowledgements
Basic Science Research Program through the National Research Foundation of Korea (NRF) funded by the Ministry of Education, Science and Technology (2012001725 and 2012008875) are gratefully acknowledged.
Notes and references
- Q. Mei, C. Jiang, G. Guan, K. Zhang, B. Liu, R. Liu and Z. Zhang, Chem. Commun., 2012, 48, 7468–7470 RSC.
- E. J. Song, H. Kim, I. H. Hwang, K. B. Kim, A. R. Kim, I. Noh and C. Kim, Sens. Actuators, B, 2014, 195, 36–43 CrossRef CAS PubMed.
- P. Kaur, K. Sareen and K. Singh, Talanta, 2011, 83, 1695–1700 CrossRef CAS PubMed.
- S. Sen, S. Sarkar, B. Chattopadhyay, A. Moirangthem, A. Basu, K. Dhara and P. Chattopadhyay, Analyst, 2012, 137, 3335–3342 RSC.
- N. Narayanaswamy and T. Govindaraju, Sens. Actuators, B, 2012, 161, 304–310 CrossRef CAS PubMed.
- D. Touati, Arch. Biochem. Biophys., 2000, 373, 1–6 CrossRef CAS PubMed.
- G. Cairo and A. Pietrangelo, Biochem. J., 2000, 352, 241–250 CrossRef CAS.
- Y. W. Choi, G. J. Park, Y. J. Na, H. Y. Jo, S. A. Lee, G. R. You and C. Kim, Sens. Actuators, B, 2014, 194, 343–352 CrossRef CAS PubMed.
- N. R. Chereddy, S. Thennarasu and A. B. Mandal, Analyst, 2013, 138, 1334–1337 RSC.
- Y. Hong, S. Chen, C. W. T. Leung, J. W. Y. Lam, J. Liu, N. W. Tseng, R. T. K. Kwok, Y. Yu, Z. Wang and B. Z. Tang, ACS Appl. Mater. Interfaces, 2011, 3, 3411–3418 CAS.
- K. B. Kim, H. Kim, E. J. Song, S. Kim, I. Noh and C. Kim, Dalton Trans., 2013, 42, 16569–16577 RSC.
- R. Joeseph, J. P. Chinta and C. P. Rao, Inorg. Chem., 2011, 50, 7050–7058 CrossRef PubMed.
- J. A. Lee, G. H. Eom, H. M. Park, J. H. Lee, H. Song, C. S. Hong, S. Yoon and C. Kim, Bull. Korean Chem. Soc., 2012, 33, 3625–3628 CrossRef CAS.
- S. Goswami, S. Das, K. Aich, D. Sarkar, T. K. Mondal, C. Kheng and H. K. Fun, Dalton Trans., 2013, 42, 15113–15119 RSC.
- R. Crichton, Inorganic Biochemistry and Iron Metabolism: From Molecular Mechanisms to Clinical Consequences, John Wiley & Sons Ltd, Chichester, 2nd edn, 2001 Search PubMed.
- M. J. Behrenfeld, A. J. Bale, Z. S. Kolber, J. Aiken and P. G. Falkowski, Nature, 1996, 383, 508–511 CrossRef CAS.
- M. A. Smith, P. L. R. Harris, L. M. Sayre and G. Perry, Proc. Natl. Acad. Sci. U. S. A., 1997, 94, 9866–9868 CrossRef CAS.
- X. Liu and E. C. Theil, Acc. Chem. Res., 2005, 38, 167–175 CrossRef CAS PubMed.
- F. A. Tezcan, J. T. Kaiser, D. Mustafi, M. Y. Walton, J. B. Howard and D. C. Rees, Science, 2005, 309, 1377–1380 CrossRef CAS PubMed.
- N. R. Chereddy, S. Janakipriya, P. S. Korrapati, S. Thennarasu and A. B. Mandal, Analyst, 2013, 138, 1130–1136 RSC.
- I. A. Koval, P. Gamez, C. Belle, K. Selmeczi and J. Reedijk, Chem. Soc.
Rev., 2006, 35, 814–840 RSC.
- N. R. Chereddy and S. Thennarasu, Dyes Pigm., 2011, 91, 378–382 CrossRef CAS PubMed.
- H. Wang, L. Yang, W. Zhang, Y. Zhou, B. Zhao and X. Li, Inorg. Chim. Acta, 2012, 381, 111–116 CrossRef CAS PubMed.
- E. L. Que, D. W. Domaille and C. J. Chang, Chem. Rev., 2008, 108, 1517–1549 CrossRef CAS PubMed.
- W. F. Fitzgerald, C. H. Lamgorg and C. R. Hammerschmidt, Chem. Rev., 2007, 107, 641–662 CrossRef CAS PubMed.
- E. M. Nolan and S. J. Lippard, Chem. Rev., 2008, 108, 3443–3480 CrossRef CAS PubMed.
- X. He, J. Zhang, X. Liu, L. Dong, D. Li, H. Qiu and S. Yin, Sens. Actuators, B, 2014, 192, 29–35 CrossRef CAS PubMed.
- M. Dong, T. H. Ma, A. J. Zhang, Y. M. Dong, Y. W. Wang and Y. Peng, Dyes Pigm., 2010, 87, 164–172 CrossRef CAS PubMed.
- X. Chen, T. Pradhan, F. Wang, J. S. Kim and J. Yoon, Chem. Rev., 2012, 112, 1910–1956 CrossRef CAS PubMed.
- J. Y. Noh, G. J. Park, Y. J. Na, H. Y. Jo, S. A. Lee and C. Kim, Dalton Trans., 2014, 43, 5652–5656 RSC.
- D. Maity, A. K. Manna, D. Karthigeyan, T. K. Kundu, S. K. Pati and T. Govindaraju, Chem.–Eur. J., 2011, 17, 11152–11161 CrossRef CAS PubMed.
- H. Zhou, J. Wang, Y. Chen, W. Xi, Z. Zheng, D. Xu, Y. Cao, G. Liu, W. hu, J. Wu and Y. Tian, Dyes Pigm., 2013, 98, 1–10 CrossRef CAS PubMed.
- Y. H. Hung, A. I. Bush and R. A. Cherny, JBIC, J. Biol. Inorg. Chem., 2010, 15, 61–76 CrossRef CAS PubMed.
- E. Gaggelli, H. Kozlowski, D. Valensin and G. Valensin, Chem. Rev., 2006, 106, 1995–2044 CrossRef CAS PubMed.
- S. Wang, S. Y. Gwon and S. H. Kim, Spectrochim. Acta, Part A, 2010, 76, 293–296 CrossRef PubMed.
- J. Huang, Y. Xu and X. Qian, Dalton Trans., 2009, 1761–1766 RSC.
- Y. Hao, D. Xiong, L. Wang, W. Chen, B. Zhou and Y. N. Liu, Talanta, 2013, 115, 253–257 CrossRef CAS PubMed.
- X. Xue, F. Wang and X. Liu, J. Am. Chem. Soc., 2008, 130, 3244–3245 CrossRef CAS PubMed.
- R. Sheng, P. Wang, Y. Gao, Y. Wu, W. Liu, J. Ma, H. Li and S. Wu, Org. Lett., 2008, 10, 529–533 CrossRef PubMed.
- G. He, L. Zhao, K. Chem, Y. Liu and H. Zhu, Talanta, 2013, 106, 73–78 CrossRef CAS PubMed.
- J. Liu, D. Wu, X. Yan and Y. Guan, Talanta, 2013, 116, 563–568 CrossRef CAS PubMed.
- S. A. Lee, G. R. You, Y. W. Choi, H. Y. Jo, A. R. Kim, I. Noh, S. Kim, Y. Kim and C. Kim, Dalton Trans., 2014, 43, 6650–6659 RSC.
- J. F. Xu, H. H. Chen, Y. Z. Chen, Z. J. Li, L. Z. Wu, C. H. Tung and Q. Z. Yang, Sens. Actuators, B, 2012, 168, 14–19 CrossRef CAS PubMed.
- J. V. R. Lis, R. M. Manez, A. Benito and J. Soto, Polyhedron, 2006, 25, 1585–1591 CrossRef PubMed.
- D. Maity and T. Govindaraju, Chem.–Eur. J., 2011, 17, 1410–1414 CrossRef CAS PubMed.
- N. Ratnarathorn, O. Chailapakul, C. S. Henry and W. Dungchai, Talanta, 2012, 99, 552–557 CrossRef CAS PubMed.
- G. J. Park, Y. J. Na, H. Y. Jo, S. A. Lee and C. Kim, Dalton Trans., 2014, 43, 6618–6622 RSC.
- E. J. Song, J. Kang, G. R. You, G. J. Park, Y. Kim, S. J. Kim, C. Kim and R. G. Harrison, Dalton Trans., 2013, 42, 15514–15520 RSC.
- H. G. Lee, J. H. Lee, S. P. Jang, H. M. Park, S. J. Kim, Y. Kim, C. Kim and R. G. Harrison, Tetrahedron, 2011, 67, 8073–8078 CrossRef CAS PubMed.
- J. Lee, H. Kim, S. Kim, J. Y. Noh, E. J. Song, C. Kim and J. Kim, Dyes Pigm., 2013, 96, 590 CrossRef CAS PubMed.
- N. Shao, Y. Zhang, S. Cheung, R. Yang, W. Chan, T. Mo, K. Li and F. Liu, Anal. Chem., 2005, 77, 7294–7303 CrossRef CAS PubMed.
- T. C. Fox, J. E. Shaff, M. A. Grusak, W. A. Norvell, Y. Chen, R. L. Chaney and L. V. Kochian, Plant Physiol., 1996, 111, 93–100 CAS.
- F. B. Abdallah, P. Arosio, P. Santambrogio, X. Yang, C. J.-. Chandler and N. D. Chasteen, Biochemistry, 2002, 41, 11184–11191 CrossRef PubMed.
- M. Guo, C. Perez, Y. Wei, E. Rapoza, G. Su, F. B.-. Abdallah and N. D. Chasteen, Dalton Trans., 2007, 4951–4961 RSC.
- Y. K. Tsui, S. Devaraj and Y. P. Yen, Sens. Actuators, B, 2012, 161, 510–519 CrossRef CAS PubMed.
- S. Huang, P. Du, C. Min, Y. Liao, H. Sun and Y. Jiang, J. Fluoresc., 2013, 23, 621–627 CrossRef CAS PubMed.
Footnote |
† Electronic supplementary Information (ESI) available. See DOI: 10.1039/c4ra02776k |
|
This journal is © The Royal Society of Chemistry 2014 |