DOI:
10.1039/C4RA02435D
(Paper)
RSC Adv., 2014,
4, 25747-25758
Synthesis and photochromic properties of benzofuran–phenanthrene and benzofuran–pyrene hybrids†
Received
20th March 2014
, Accepted 30th April 2014
First published on 30th April 2014
Abstract
Iron(III) chloride mediated condensation of phenanthrene-9,10-dione and pyrene-4,5-dione with cyclohexanone and its derivatives furnish benzofuran–phenanthrene and benzofuran–pyrene hybrids. Absorption and emission spectroscopic studies revealed the bathochromic effect of benzofuran annulation on the photophysical properties of the pyrene chromophore. Solvatochromic studies revealed that solvent polarity did not have a noticeable effect on the absorption or the emission spectra. While benzofuran annulation enhanced the quantum yield in most of the solvents, appending a methyl group, in fact, reduced the quantum yield. Thus, our studies delineated the synthesis and photophysical properties of selected PAHs with a furan ring.
1. Introduction
Polycyclic aromatic hydrocarbons (PAH) represented by common molecules like phenanthrene 1 and pyrene 2 (Fig. 1) are a major class of organic compounds.1 Many PAHs are released into the environment as pollutants primarily due to human intervention. It is well known that PAHs cause cancer, allergies and other ailments.2 While most PAHs have a negative impact on nature, some of them, particularly those having more than five rings, have emerged as technologically useful light harvesting materials owing to their unique optoelectronic properties.3 Although many PAHs are known and well characterized, PAHs incorporating heteroaromatic rings are rare.4 However, some heteroaromatic ring fused PAHs are naturally occurring compounds and exhibit interesting applications. For example, a few naturally occurring PAHs with fused furan and phenanthrene rings5 act as non-steroidal anti-inflammatory agents.6 Some heteroaromatic ring annulated phenanthrenes show useful photochromic properties.7 Therefore, the synthesis and structural elucidation of PAHs incorporating heterocyclic rings are of high interest.
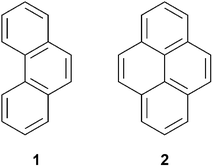 |
| Fig. 1 Structure of phenanthrene 1, pyrene 2. | |
We have recently disclosed an iron(III) chloride catalyzed synthesis of several furan annulated phenanthrenes from 9,10-phenanthrenequinone (PQ) 3 and aliphatic as well as alicyclic ketones.8 As an example, condensation of PQ 3 with cyclohexanone 4a in the presence of 6 mol% of iron(III) chloride under neat conditions provided furan annulated product 5 (Scheme 1). In contrast, when this reaction was conducted under aerobic conditions with one equivalent of iron(III) chloride, we obtained benzofuran annulated phenanthrene 6a (Scheme 1). Since the reaction provided easy and ready access to benzo[b]furan annulated phenanthrene, we explored the reaction further for the synthesis of various benzo[b]furans annulated to phenanthrene 6, the details of which we describe herein. We extended the synthetic studies to benzo[b]furans annulated to pyrene 8 (Scheme 4) and also evaluated the photochromic properties of the resulting pyrene–benzofuran hybrids. Furthermore, the spectroscopic properties of the pyrene–benzofuran hybrids were evaluated by theoretical studies.
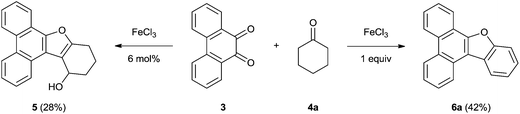 |
| Scheme 1 FeCl3 mediated reaction of PQ 3 with cyclohexanone to provide furan annulated products 5 or 6a. | |
2. Results and discussion
Condensation of PQ 3 with cyclohexanone 4a was employed as a test case to work out the optimal conditions for generation of the benzofuran–phenanthrene hybrid 6a (Scheme 1). The condensation worked well with a stoichiometric amount of iron(III) chloride to provide phenanthrene–benzofuran hybrid 6a in moderate yield (42%). Unfortunately, when we employed alternative Lewis acids like iron(III) acetylacetonate, iron(III) sulfate, copper(II) sulfate, copper(I) bromide, zinc(II) chloride, titanium(IV) chloride, aluminum(III) chloride, I2 and Et2OBF3 in catalytic or stoichiometric amounts, no useful product could be isolated. The reaction between PQ 3 and cyclohexanone 4a to provide 6a worked well under neat conditions in cyclohexanone (5 equivalents). The product 6a could be recovered from the reaction mixture by removing cyclohexanone under reduced pressure and isolating the product by chromatographic purification of the residue. Formation of benzofuran–phenanthrene hybrid 6a was deduced from its characteristic 20 line 13C NMR and DEPT spectra.
To evaluate the generality of the above transformation, we subjected PQ 3 to iron(III) chloride mediated condensation with 2-methylcyclohexanone 4b and the reaction provided phenanthrobenzofuran 6b as the only product in moderate yield (Scheme 2). The reaction of PQ with 3-methylcyclohexanone 4c could provide two isomeric phenanthrobenzofurans 6c and 6d. The reaction, however, furnished a single product, the structure of which was unambiguously assigned as 6c on the basis of 1H and 2D NMR spectral data and in comparison with compounds with the 4-alkyldibenzo[b,d]furan motif.9 The reaction of PQ with 4-methylcyclohexanone 4d provided phenanthrobenzofuran 6e uneventfully. The condensation of PQ with α-tetralone 4e and 6-methoxy-α-tetralone 4f also took place, albeit in lower yield, to provide the corresponding naphthofuran phenanthrene hybrids 6f and 6g respectively. It is to be noted that the last reaction, however, needed to be conducted in dichloroethane (DCE) as solvent as all the reacting components namely PQ, 6-methoxy-α-tetralone and iron(III) chloride are solids.
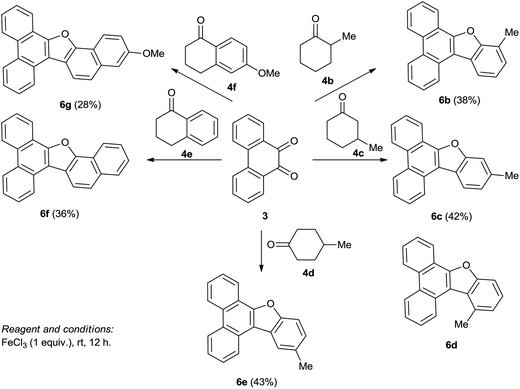 |
| Scheme 2 FeCl3 mediated condensation of PQ with substituted cyclohexanones. | |
Based on the above experimental results, a possible pathway for the formation of benzofuran–phenanthrene hybrids 6 is given in Scheme 3. The first major intermediate formed is the secondary alcohol 5 generated by cascade steps involving aldol condensation, cyclization and rearrangement.8 Subsequently, iron(III) chloride mediated dehydration followed by dehydrogenative aromatization furnished benzofuran–phenanthrene hybrid 6a. Indeed, when the intermediate 5, reported by us previously,8 was treated with iron(III) chloride under the present reaction conditions 6a was formed in quantitative yield. The role of iron(III) chloride appears to be that of being the most appropriate Lewis acid facilitating each of the cascade steps like aldol condensation, cyclization, rearrangement of the hydroxyl group, dehydration and dehydrogenative aromatization etc.
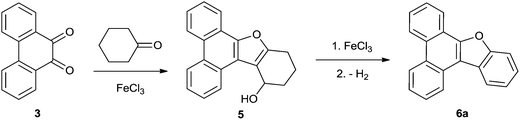 |
| Scheme 3 Proposed pathway for the formation of furan annulated phenanthrene. | |
Next, we conducted a series of reactions of iron(III) chloride mediated condensation of pyrene-4,5-dione 7 with cyclohexanone 4a, 2-methylcyclohexanone 4b, 3-methylcyclohexanone 4c and 4-methylcyclohexanone 4d (Scheme 4) with the intention of studying the photochromic properties of the resulting benzofuran pyrene hybrids 8a–d. In each case the corresponding benzofuran pyrene hybrids 8a–d formed, albeit in lower yield compared to those formed when PQ 3 was involved. The spectra of 8a–d compared well with the spectra of benzofuran–phenanthrene hybrids 6a–e and the structure of 8b was confirmed by single crystal X-ray structure analysis (Fig. 2). Unfortunately our attempts to induce condensation of pyrene-4,5-dione 7 with α-tetralone 4e or 6-methoxy-α-tetralone 4f proved to be futile.
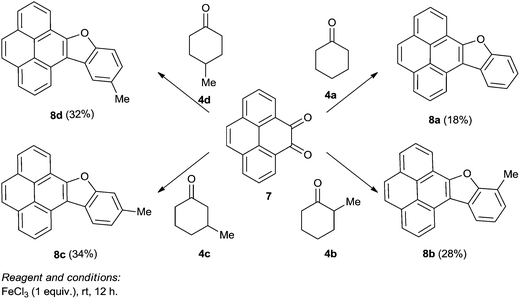 |
| Scheme 4 FeCl3 mediated condensation of pyrene-4,5-dione with substituted cyclohexanones. | |
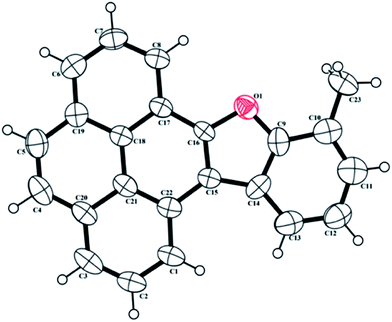 |
| Fig. 2 ORTEP diagram of 10-methylbenzo[d]pyreno[4,5-b]furan 8b† with crystallographic numbering. | |
3. Absorption and emission spectra of pyrene appended benzofurans 8a–d
Of all the polycyclic hydrocarbons, pyrene and its derivatives have attracted the most attention owing to their useful photoluminescence properties, high thermal stability and easy synthesis.10 Several pyrene derivatives exhibit intense fluorescence emission with high sensitivity to their surroundings, which makes them ideal candidates for being micro-environment sensors.11 The useful photo-luminance properties of pyrene derivatives lead them to be explored for technological applications like organic light-emitting diodes (OLEDs),12 liquid crystal lasers13 and dye sensitized solar cells.14 Previously it was shown that benzo[b]furan annulated pyrene 8c, one of the pyrene derivatives prepared in this study, undergoes self-assembly into nano-wires and consequently exhibits light-emitting diode properties.15 We have now investigated the absorption and emission properties of all the newly prepared pyrene benzofuran hybrids 8a–d in different solvents and compared the optical properties with the those of the parent pyrene and 10-methylpyreno[4,5-b]furan 9 which we synthesized according to Scheme 5.
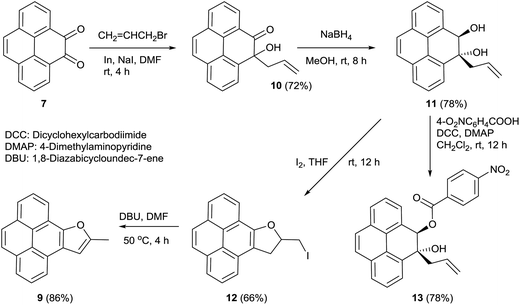 |
| Scheme 5 Four-step synthesis of 10-methylpyreno[4,5-b]furan 9 from pyrene-4,5-dione 7. | |
We achieved the synthesis of 10-methylpyreno[4,5-b]furan 9 starting from pyrene-4,5-dione 7 in four convenient steps. Indium metal mediated allylation of 7 delivered keto–alcohol 10.16 Stereoselective reduction of the carbonyl group in 10 with sodium borohydride furnished trans-diol 11. Anchimeric assistance provided by the adjacent hydroxyl group allowed syn-facial delivery of hydride ion by sodium borohydride. The structure of 11 was deduced from analysis of the 2D NMR spectra, especially NOESY. Furthermore, the structure of 11 was unequivocally established by single crystal X-ray structure analysis of its 4-nitrobenzoate derivative 13 (Fig. 3). The iodine mediated cyclization of 11 delivered the dihydrofuran derivative 12.17 Finally dehydroiodination of 12 with the non-nucleophilic base 1,8-diazabicycloundec-7-ene (DBU) yielded furan appended pyrene 9.
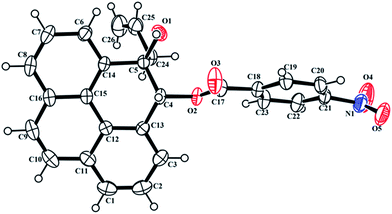 |
| Fig. 3 ORTEP diagram of (4RS,5RS)-5-allyl-5-hydroxy-4,5-dihydropyren-4-yl 4-nitrobenzoate 13† with crystallographic numbering. | |
First, UV-vis spectra of pyrene 2, 10-methylpyreno[4,5-b]furan 9, benzo[d]pyreno[4,5-b]furan 8a, 10-methylbenzo[d]pyreno[4,5-b]furan 8b, 11-methylbenzo[d]pyreno[4,5-b]furan 8c, 12-methylbenzo[d]pyreno[4,5-b]furan 8d were measured as dilute solutions in deaerated MeOH (Fig. 4A). As described in Fig. 4A, upon the installation of a 2-methylfuran ring on the pyrene scaffold the most intense band in the UV spectrum showed a red shift of 10 nm and upon introduction of a benzofuran ring the band further red shifted to the extent of 8 nm. The red shift indicates that the entire PAH is flat and the HOMO–LUMO band gaps of pyrene and the benzofuran annulated pyrenes (ΔE = (HOMO − LUMO)) tend to become narrower with increased conjugation. Similar to pyrene which exhibits three broad bands between 300 and 350 nm in MeOH, the furanopyrene 9 and benzofuranopyrenes 8a–d exhibit three bands but with much finer structure. In addition, they exhibit a low intensity band at about 385 nm. The UV spectra of pyrene, 9 and 8a–d recorded in six different solvents, namely hexane (non-polar), ethyl acetate (EA, moderately polar aprotic), dichloromethane (DCM, moderately polar aprotic), methanol (MeOH, polar protic), ethanol (EtOH, polar protic) and dimethylformamide (DMF, highly polar aprotic), do not show much variance in the absorption maximum values (Fig. 5, Table 1), excepting in EtOH in which the bands show much finer structure and a hyperchromic effect.
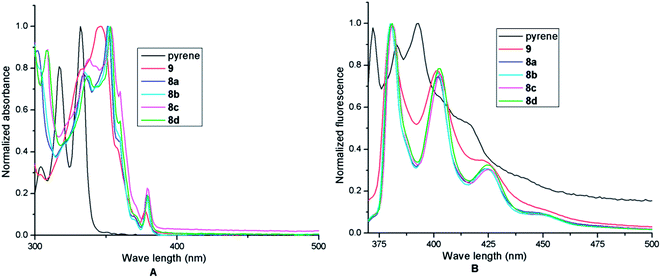 |
| Fig. 4 Normalized UV-vis and fluorescence spectra of pyrene, 10-methylpyreno[4,5-b]furan 9, benzo[d]pyreno[4,5-b]furan 8a, 10-methylbenzo[d]pyreno[4,5-b]furan 8b, 11-methylbenzo[d]pyreno[4,5-b]furan 8c, 12-methylbenzo[d]pyreno[4,5-b]furan 8d (MeOH, λex = λabs,max). | |
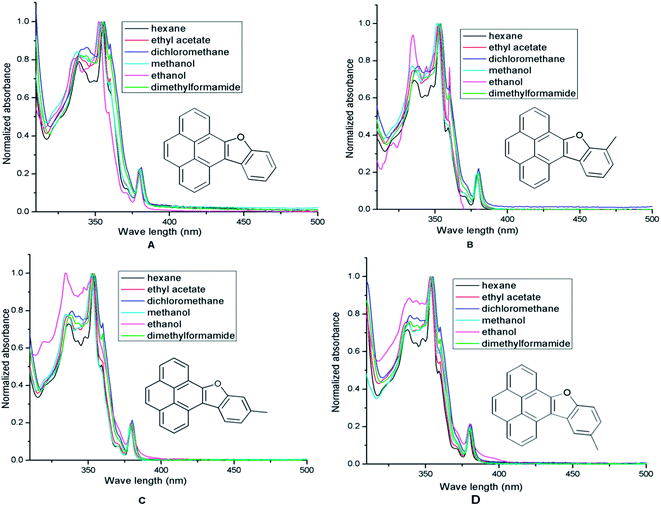 |
| Fig. 5 Normalized absorbance spectra of pyrene benzofuran hybrids (A) 8a; (B) 8b; (C) 8c; (D) 8d in hexane (black), EtOAc (red), DCM (blue), MeOH (cyan), EtOH (magenta), DMF (green). | |
Table 1 Absorption characteristics of the most intense band of the compounds pyrene, 9, 8a–d in hexane, ethyl acetate (EtOAc), dichloromethane (DCM), MeOH, EtOH and dimethylformamide (DMF); HOMO and LUMO energies were calculated by TD-DFT/B3LYP/6-311G(d,p) using Gaussian
Entry |
Compound |
Hexane (nm) |
EtOAc (nm) |
DCM (nm) |
MeOH (nm) |
EtOH (nm) |
DMF (nm) |
HOMO (ev) |
LUMO (ev) |
(LUMO–HOMO) (ev) |
1 |
Pyrene |
333 |
334 |
336 |
333 |
334 |
336 |
−5.67 |
−1.86 |
3.81 |
2 |
9 |
350 |
347 |
350 |
343 |
347 |
349 |
−5.46 |
−1.74 |
3.72 |
3 |
8a |
353 |
352 |
354 |
351 |
352 |
353 |
−5.62 |
−1.97 |
3.65 |
4 |
8b |
354 |
353 |
354 |
352 |
353 |
354 |
−5.59 |
−1.94 |
3.65 |
5 |
8c |
355 |
354 |
356 |
353 |
354 |
355 |
−5.55 |
−1.92 |
3.63 |
6 |
8d |
354 |
353 |
354 |
352 |
353 |
354 |
−5.58 |
−1.93 |
3.65 |
The DFT studies were conducted on pyrene, furan annulated pyrene 9 and benzofuran annulated pyrenes 8a–d to determine the HOMO–LUMO energy gaps (Table 1). A decrease in the HOMO–LUMO energy difference (ΔE) upon moving from pyrene to furan annulated pyrene 9 (entries 1 and 2, Table 1), and as a consequence the red shift of the λmax, is due to increase of delocalization in 9 arising from annulation of the furan ring. Similarly, a further red-shift in benzofuran annulated pyrene 8a (entry 3) in all the solvents is due to an increase in delocalization. Among the three isomeric methylpyreno[4,5-b]benzofurans 8b–d (entries 4–6) the lowest HOMO–LUMO energy gap was noticed for 11-methylpyreno[4,5-b]benzofuran 8c (entry 5). This observation indicates that compared to a methyl group located on C10 (8b) or C12 (8d), one on C11 (8c) participates in the resonance better, possibly through σ–π conjugation (hyperconjugation).
Similar to pyrene which exhibits three bands (I, II and III) in its fluorescence spectrum in MeOH, 9 and 8a–d exhibit three bands in their respective spectra (Fig. 4B, Table 2). Interestingly, the highly structured fluorescence of pyrene, known as the Ham effect,18 was not retained after introduction of an appended furan or benzofuran ring which indicates clear vibrational structures for 9 and 8a–d. While the red shift owing to introduction of a furan ring is clearly discernible in 9 for all the bands including the (0–0) band, further red shifts anticipated after benzofuran annulation 8a–d are nearly absent (Fig. 4B).
Table 2 Emission characteristics of the compounds pyrene, 10-methylpyreno[4,5-b]furan 9, 8a–d in hexane, EtOAc, DCM, MeOH, EtOH and DMF
Entry |
Compound |
Hexane |
EtOAc |
DCM |
MeOH |
EtOH |
DMF |
1 |
Pyrene |
372, 383, 393 |
372, 384, 393 |
373, 384, 394 |
372, 383, 393 |
372, 383, 393 |
373, 384, 394 |
2 |
9 |
379, 400, 418 |
380, 402, 423 |
382, 404, 427 |
380, 401, 420 |
380, 402, 424 |
382, 403, 425 |
3 |
8a |
380, 402, 424 |
381, 402, 424 |
381, 403, 426 |
380, 402, 424 |
381, 403, 426 |
382, 403, 425 |
4 |
8b |
380, 402, 425 |
381, 402, 425 |
382, 403, 426 |
380, 402, 424 |
380, 402, 425 |
382, 403, 426 |
5 |
8c |
381, 403, 425 |
381, 403, 426 |
383, 404, 427 |
381, 403, 425 |
380, 402, 426 |
382, 404, 427 |
6 |
8d |
381, 403, 425 |
381, 403, 425 |
382, 404, 426 |
381, 402, 425 |
381, 403, 426 |
382, 404, 427 |
We calculated the relative fluorescence quantum yields (Φf) for the furan annulated pyrene 9 and benzofuran annulated pyrenes 8a–d by taking 9,10-diphenylanthracene in EtOH (Φf = 0.95) as a standard (Table 3).19 The analysis showed that compared to furan annulated pyrene 9 the quantum yields of benzofuran annulated pyrenes 8a–d increased in all the solvents employed. Among the benzofuran annulated pyrenes, 8a displayed higher quantum yields compared to 8b–d in all solvents excepting in dichloromethane (DCM; Table 3). In DCM however, the quantum yield of 8d is greater than that of the rest of the benzofuran annulated pyrenes, which indicates that in DCM the methyl group at C12 has the highest influence on fluorescence possibly due to better σ–π conjugation in the excited state.20 The fluorescence spectra recorded in six solvents of different polarities (Fig. 6) did not show much variance which makes the benzofuran pyrene hybrids 9 and 8a–d Acree non-probe PAHs.21
Table 3 Quantum yields of 9, 8a–d in hexane, EtOAc, DCM, MeOH, EtOH and DMF
Entry |
Compound |
Hexane |
EtOAc |
DCM |
MeOH |
EtOH |
DMF |
1 |
9 |
0.17 |
0.23 |
0.38 |
0.18 |
0.28 |
0.40 |
2 |
8a |
0.24 |
0.37 |
0.41 |
0.33 |
0.43 |
0.51 |
3 |
8b |
0.22 |
0.35 |
0.41 |
0.28 |
0.34 |
0.47 |
4 |
8c |
0.22 |
0.34 |
0.39 |
0.29 |
0.38 |
0.45 |
5 |
8d |
0.21 |
0.34 |
0.49 |
0.32 |
0.43 |
0.43 |
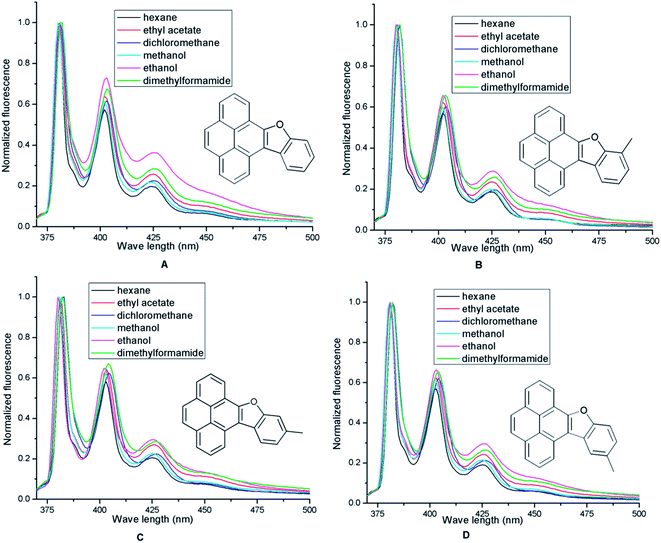 |
| Fig. 6 Normalized emission spectra of compounds (A) 8a; (B) 8b; (C) 8c; (D) 8d in hexane (black), EtOAc (red), DCM (blue), MeOH (cyan), EtOH (magenta), DMF (green). | |
4. Conclusion
In summary we have delineated a facile FeCl3 mediated synthesis of benzofuran phenanthrene and benzofuran pyrene hybrid PAHs by condensation of 9,10-phenanthrenequinone or pyrene-4,5-dione with cyclohexanone and its derivatives. Absorption and emission properties of the benzofuran pyrene hybrids were studied which reflect the expanded conjugation owing to annulation of a furan or benzofuran ring to pyrene. Among the benzofuran annulated pyrenes ones without any methyl appendage exhibited a high quantum yield in most of the solvents compared to those which had a methyl group. Solvatochromic studies revealed that polarity of solvents did not have noticeable effect on the absorption or the emission spectra.
5. Experimental section
5.1 General
All melting points were uncorrected and were determined using open-ended capillary tubes on VEEGO VMP-DS instrument. The reactions and chromatographic separations were monitored by thin layer chromatography. Glass plates coated with silica gel (SRL chemicals) were used for thin layer chromatography. Column chromatography was carried out on silica gel (100–200 mesh) using hexane and ethyl acetate as eluent. IR spectra were recorded as KBr pellets on a Nicolet-6700 spectrometer. Electronic absorption spectra were obtained on a Shimadzu UV-2450 spectrophotometer. Emission spectra were recorded on a Fluoromax-4 spectrofluorometer. Solvents used for UV-vis and fluorescence spectroscopic experiments were of spectroscopic grade. 1H-NMR (400 MHz), 13C-NMR (100 MHz) and DEPT-135 spectra were recorded for (CDCl3 or CDCl3 + CCl4, 1
:
1) solutions on a Bruker-400 spectrometer with tetramethylsilane (TMS) as internal standard; J-values are in Hz. 1H-NMR data are reported as follows: chemical shift (multiplicity (s = singlet, d = doublet, dd = doublet of doublet, t = triplet and m = multiplet), coupling constant, integrations, type of proton). 13C NMR spectra were determined with 1H decoupling. Elemental analysis (C, H and N) was performed using Thermo Scientific FLASH 2000 Organic Elemental Analyzer. High resolution mass spectra were recorded on a Water Q-TOF micro mass spectrometer using the electron spray ionization mode. The X-ray diffraction measurements were carried out at 298 K on an Oxford CrysAlis CCD area detector system equipped with a graphite monochromator and a Mo-Kα fine-focus sealed tube (λ = 0.71073 Å). PQ 3, pyrene, cyclohexanones and FeCl3 were procured from commercial sources and used as received. Pyrene-4,5-dione 7 was prepared according to the literature procedure.22 The equilibrium structures of the benzofuran–pyrene hybrids were fully optimized by the B3LYP method with 6-311G(d,p) basis set. All calculations were performed by using the Gaussian program.
5.2 General procedure for iron(III) chloride mediated reactions of PQ or pyrene 4,5-dione with ketones
To 9,10-phenanthrenequinone or pyrene-4,5-dione (1 equiv.) taken in a covered 10 mL test tube the cyclic ketone (5 equiv.) was added and stirred at rt for 5 min. To the resulting mixture anhydrous FeCl3 (1 equiv.) was added and stirring continued at rt for 12 h. After completion of the reaction (absence of PQ or pyrene-4,5-dione by TLC) excess ketone was evaporated under reduced pressure. The crude product was purified by column chromatography using silica gel (100–200 mesh) and 100% hexane as eluent.
5.2.1. Benzo[d]phenanthro[9,10-b]furan (6a). Following the general procedure, the reaction of 9,10-phenanthrenequinone (200.2 mg, 0.96 mmol), cyclohexanone (471.3 mg, 4.80 mmol) and iron(III) chloride (154.6 mg, 0.96 mmol) afforded benzo[d]phenanthro[9,10-b]furan 6a as a white solid in 42% yield, mp 153–154 °C, reported 156–158 °C;23 IR (KBr) νmax 3021, 1612, 1582, 1519, 1447, 1359, 1322, 1219, 1163, 1113, 1065, 1034, 960, 748, 721, 691, 663 cm−1; 1H NMR (400 MHz, CDCl3 + CCl4) δ 8.72–8.68 (m, 2H, ArH4, H5), 8.58 (dd, J = 8.0, 1.0 Hz, 1H, ArH1), 8.49–8.47 (m, 1H, ArH8), 8.35–8.33 (m, 1H, ArH13), 7.75–7.60 (m, 5H, ArH2, H3, H6, H7 and H10), 7.50–7.43 (m, 2H, ArH11, H12) ppm; 13C NMR (100 MHz, CDCl3 + CCl4) 156.0 (C), 151.4 (C), 130.8 (C), 128.8 (C), 128.4 (C), 127.5 (CH), 127.20 (CH), 127.15 (CH), 125.9 (C), 125.5 (CH), 125.2 (CH), 124.3 (CH), 123.9 (CH), 123.54 (CH), 123.49 (CH), 122.4 (C), 121.9 (2 × CH), 114.6 (C), 112.1 (CH) ppm; anal. calcd for C20H12O: C, 89.53; H, 4.51. Found: C, 89.19; H, 4.50%.
5.2.2. 10-Methylbenzo[d]phenanthro[9,10-b]furan (6b). Following the general procedure, the reaction of 9,10-phenanthrenequinone (201.2 mg, 0.96 mmol), 2-methylcyclohexanone (538.4 mg, 4.80 mmol) and iron(III) chloride (156.2 mg, 0.96 mmol) afforded 10-methylbenzo[d]phenanthro[9,10-b]furan 6b as a white solid in 38% yield, mp 195–196 °C, reported 197–198 °C;24 IR (KBr) νmax 3057, 2916, 2852, 1612, 1585, 1513, 1450, 1422, 1356, 1317, 1219, 1197, 1143, 1088, 1030, 932, 854, 761, 735, 690 cm−1; 1H NMR (400 MHz, CDCl3 + CCl4) δ 8.76–8.72 (m, 2H, ArH4, H5), 8.61 (dd, J = 8.1, 1.1 Hz, 1H, ArH1), 8.55–8.52 (m, 1H, ArH8), 8.18 (d, J = 7.7 Hz, 1H, ArH13), 7.76–7.62 (m, 4H, ArH2, H3, H6 and H7), 7.35 (t, J = 7.6 Hz, 1H, ArH12), 7.28 (d, J = 7.5 Hz, 1H, ArH11), 2.75 (s, 3H, CH3) ppm; 13C NMR (100 MHz, CDCl3 + CCl4) δ 154.9 (C), 151.1 (C), 130.7 (C), 129.0 (C), 128.4 (C), 127.5 (CH), 127.2 (CH), 127.1 (CH), 126.7 (CH), 125.3 (C), 125.1 (CH), 124.4 (CH), 123.9 (CH), 123.58 (CH), 123.55 (CH), 122.6 (C), 122.2 (C), 122.0 (CH), 119.5 (CH), 115.0 (C), 15.7 (CH3) ppm; anal. calcd for C21H14O: C, 89.34; H, 5.00. Found: C, 89.09; H, 4.98%.
5.2.3. 11-Methylbenzo[d]phenanthro[9,10-b]furan (6c). Following the general procedure, the reaction of 9,10-phenanthrenequinone (101.8 mg, 0.48 mmol), 3-methylcyclohexanone (268.2 mg, 2.40 mmol) and iron(III) chloride (78.1 mg, 0.48 mmol) afforded 11-methylbenzo[d]phenanthro[9,10-b]furan 6c as a white solid in 42% yield, mp 202–203 °C; IR (KBr) νmax 3075, 3028, 2919, 2852, 1616, 1517, 1449, 1354, 1227, 1190, 1113, 1066, 1037, 999, 959, 861, 802, 755, 719, 678 cm−1; 1H NMR (400 MHz, CDCl3 + CCl4) δ 8.77–8.72 (m, 2H, ArH4, H5), 8.60 (dd, J = 8.1, 1.1 Hz, 1H, ArH1), 8.50–8.47 (m, 1H, ArH8), 8.21 (d, J = 8.0 Hz, 1H, ArH13), 7.77–7.64 (m, 5H, ArH2, H3, H6, H7 and H12), 7.54 (s, 1H, ArH10), 2.59 (s, 3H, CH3) ppm; 13C NMR (100 MHz, CDCl3 + CCl4) δ 156.2 (C), 150.8 (C), 135.6 (C), 130.2 (C), 128.5 (C), 128.0 (C), 127.2 (CH), 126.9 (CH), 126.6 (CH), 124.8 (CH), 124.5 (CH), 124.1 (CH), 123.6 (CH), 123.3 (CH), 123.0 (C), 122.2 (C), 121.5 (CH), 121.1 (CH), 114.4 (C), 112.1 (CH), 21.8 (CH3) ppm; anal. calcd for C21H14O: C, 89.34; H, 5.00. Found: C, 89.29; H, 4.99%.
5.2.4. 12-Methylbenzo[d]phenanthro[9,10-b]furan (6e). Following the general procedure, the reaction of 9,10-phenanthrenequinone (101.2 mg, 0.48 mmol), 4-methylcyclohexanone (271.2 mg, 2.40 mmol) and iron(III) chloride (78.4 mg, 0.48 mmol) afforded 12-methylbenzo[d]phenanthro[9,10-b]furan 6e as a white solid in 43% yield, mp 166–167 °C, reported 168–169 °C;25 IR (KBr) νmax 3069, 2921, 2856, 1611, 1515, 1458, 1375, 1325, 1219, 1157, 1114, 1069, 1037, 936, 859, 798, 755, 722, 669 cm−1; 1H NMR (400 MHz, CDCl3) δ 8.77–8.71 (m, 2H, ArH4, H5), 8.59 (dd, J = 8.1, 1.0 Hz, 1H, ArH1), 8.48–8.46 (m, 1H, ArH8), 8.12 (s, 1H, ArH13), 7.77–7.61 (m, 5H, ArH2, H3, H6, H7 and H10), 7.29 (dd, J = 8.3, 1.1 Hz, 1H, ArH11), 2.61 (s, 3H, CH3) ppm; 13C NMR (100 MHz, CDCl3) δ 154.2 (C), 151.3 (C), 132.7 (C), 130.4 (C), 128.6 (C), 128.1 (C), 127.3 (CH), 127.0 (CH), 126.9 (CH), 126.5 (CH), 125.6 (C), 124.9 (CH), 124.1 (CH), 123.7 (CH), 123.3 (CH), 122.3 (C), 121.7 (CH), 121.6 (CH), 114.2 (C), 111.3 (CH), 21.7 (CH3) ppm; anal. calcd for C21H14O: C, 89.34; H, 5.00. Found: C, 89.61; H, 4.99%.
5.2.5. Naphtho[2,1-d]phenanthro[9,10-b]furan (6f). Following the general procedure, the reaction of 9,10-phenanthrenequinone (100.3 mg, 0.48 mmol), α-tetralone (351.2 mg, 2.40 mmol) and iron(III) chloride (79.1 mg, 0.48 mmol) afforded naphtho[2,1-d]phenanthro[9,10-b]furan as a white solid 6f in 36% yield, mp 205–206 °C, reported 208–210 °C;26 IR (KBr) νmax 3055, 1611, 1521, 1450, 1376, 1290, 1218, 1112, 1074, 1023, 938, 871, 803, 757, 719, 674 cm−1; 1H NMR (400 MHz, CDCl3) δ 8.82–8.73 (m, 3H, ArH4, H5, H10), 8.67–8.61 (m, 2H, ArH1, H8), 8.45 (d, J = 8.4 Hz, 1H, ArH13), 8.04 (d, J = 7.7 Hz, 1H, ArH15), 7.90 (d, J = 7.7 Hz, 1H, ArH14), 7.80–7.59 (m, 6H, ArH2, H3, H6, H7, H11 and H12) ppm; 13C NMR (100 MHz, CDCl3) δ 151.6 (C), 150.8 (C), 132.0 (C), 130.2 (C), 128.7 (C), 128.53 (C), 128.49 (CH), 127.5 (CH), 127.4 (CH), 126.9 (CH), 126.8 (CH), 126.0 (CH), 125.4 (CH), 124.4 (CH), 124.1 (CH), 124.0 (CH), 123.6 (CH), 122.6 (C), 121.7 (C), 121.6 (CH), 121.0 (C), 120.8 (CH), 120.0 (CH), 115.7 (C) ppm; anal. calcd for C24H14O: C, 90.54; H, 4.43. Found: C, 89.99; H, 4.43%.
5.2.6. 12-Methoxynaphtho[2,1-d]phenanthro[9,10-b]furan (6g). Following the general procedure, the reaction of 9,10-phenanthrenequinone (100.2 mg, 0.48 mmol), 6-methoxy α-tetralone (169.8 mg, 0.96 mmol) and iron(III) chloride (78.8 mg, 0.48 mmol) in 0.5 mL dry dichloroethane (DCE) as solvent afforded 12-methoxynaphtho[2,1-d]phenanthro[9,10-b]furan 6g as a white solid in 28% yield, mp 220–221 °C; IR (KBr) νmax 3057, 3010, 2933, 2833, 1639, 1600, 1473, 1453, 1416, 1371, 1328, 1266, 1244, 1218, 1187, 1164, 1142, 1075, 1030, 936, 844, 819, 792, 755, 719, 689, 671 cm−1; 1H NMR (400 MHz, CDCl3) δ 8.76 (d, J = 8.3 Hz, 2H, ArH4, H5), 8.69 (d, J = 7.8 Hz, 1H, ArH1), 8.60 (dd, J = 8.0, 1.0 Hz, 1H, ArH8), 8.49 (d, J = 8.7 Hz, 1H, ArH15), 8.38 (d, J = 8.7 Hz, 1H, ArH14), 7.79–7.66 (m, 5H, ArH2, H3, H6, H7 and H10), 7.36–7.33 (m, 2H, ArH11, H13), 3.99 (s, 3H, OCH3) ppm; 13C NMR (100 MHz, CDCl3) δ 157.9 (C), 152.0 (C), 150.3 (C), 134.2 (C), 133.4 (C), 129.9 (C), 128.7 (C), 128.5 (C), 127.4 (CH), 127.3 (CH), 126.6 (CH), 125.2 (CH), 124.3 (CH), 123.9 (CH), 123.6 (CH), 123.0 (CH), 122.5 (CH), 121.4 (CH), 120.6 (CH), 119.2 (CH), 119.1 (C), 116.9 (C), 115.7 (C), 107.1 (CH), 55.5 (CH3) ppm; anal. calcd for C25H16O2: C, 86.19; H, 4.63. Found: C, 86.13; H, 4.61%.
5.2.7. Benzo[d]pyreno[4,5-b]furan (8a). Following the general procedure, the reaction of pyrene-4,5-dione (100.2 mg, 0.43 mmol), cyclohexanone (211.2 mg, 2.15 mmol) and iron(III) chloride (69.8 mg, 0.43 mmol) afforded benzo[d]pyreno[4,5-b]furan 8a as a white solid in 18% yield, mp 198–199 °C; IR (KBr) νmax 1593, 1448, 1357, 1276, 1217, 1175, 1105, 1062, 894, 869, 821, 767, 741, 710 cm−1; 1H NMR (400 MHz, CDCl3) δ 8.81 (dd, J = 7.6, 1.2 Hz, 1H, ArH3), 8.70 (dd, J = 7.7, 1.1 Hz, 1H, ArH6), 8.47–8.45 (m, 1H, ArH13), 8.21 (dd, J = 7.7, 1.0 Hz, 1H, ArH4), 8.16 (dd, J = 7.7, 1.2 Hz, 1H, ArH5), 8.12–8.06 (m, 4H, ArH1, H2, H7 and H8), 7.83–7.81 (m, 1H, ArH10), 7.55–7.52 (m, 2H, ArH11, H12) ppm; 13C NMR (100 MHz, CDCl3) δ 156.1 (C), 151.8 (C), 132.0 (C), 131.7 (C), 128.2 (CH), 127.7 (C), 127.5 (CH), 126.5 (CH), 126.2 (CH), 125.9 (CH + C), 125.7 (CH), 124.44 (C), 124.42 (CH), 123.6 (CH), 122.7 (C), 122.0 (CH), 121.38 (C), 121.36 (CH), 118.8 (CH), 115.1 (C), 112.1 (CH) ppm; anal. calcd for C22H12O: C, 90.39; H, 4.14. Found: C, 89.82; H, 4.14%.
5.2.8. 10-Methylbenzo[d]pyreno[4,5-b]furan (8b). Following the general procedure, the reaction of pyrene-4,5-dione (100.8 mg, 0.43 mmol), 2-methylcyclohexanone (241.6 mg, 2.15 mmol) and iron(III) chloride (69.7 mg, 0.43 mmol) afforded 10-methylbenzo[d]pyreno[4,5-b]furan 8b as a white solid in 28% yield, mp 188–189 °C; IR (KBr) νmax 3040, 2923, 2857, 1594, 1456, 1364, 1311, 1266, 1179, 1092, 1029, 965, 896, 832, 781, 746, 712 cm−1; 1H NMR (400 MHz, CDCl3) δ 8.82 (dd, J = 7.6, 1.2 Hz, 1H, ArH3), 8.73 (dd, J = 7.7, 1.1 Hz, 1H, ArH6), 8.29 (d, J = 7.6 Hz, 1H, ArH4), 8.21 (dd, J = 7.7, 1.1 Hz, 1H, ArH5), 8.18–8.05 (m, 5H, ArH1, H2, H7, H8 and H13), 7.42 (t, J = 7.5 Hz, 1H, ArH12), 7.33 (d, J = 7.2 Hz, 1H, ArH11), 2.78 (s, 3H, CH3) ppm; 13C NMR (100 MHz, CDCl3) δ 155.0 (C), 151.6 (C), 132.0 (C), 131.7 (C), 128.2 (CH), 127.8 (C), 127.4 (CH), 126.7 (CH), 126.5 (CH), 126.1 (CH), 125.8 (CH), 125.3 (C), 124.4 (C), 124.3 (CH), 123.5 (CH), 122.7 (C), 122.3 (C), 121.5 (C), 121.4 (CH), 119.4 (CH), 118.8 (CH), 115.5 (C), 15.5 (CH3) ppm; anal. calcd for C23H14O: C, 90.17; H, 4.61. Found: C, 89.89; H, 4.63%.
Crystal data for 8b. Empirical formula, C23H14O; formula weight, 306.35; crystal color, habit: colorless, rectangular block; crystal system, orthorhombic; crystal dimensions, 0.45 × 025 × 0.20 mm3; lattice parameters, a = 4.8868(5) Å, b = 16.136(3) Å, c = 19.0524(16) Å; α = 90.00, β = 90.00, γ = 90.00; V = 1502.3(3) A3; space group P2
; Z = 4; Dcalcd = 1.354 g cm−3; F000 = 640; λ (Mo-Kα) = 0.7107 Å; R(I ≥ 2σ1) = 0.0745, wR2 = 0.2324.†
5.2.9. 11-Methylbenzo[d]pyreno[4,5-b]furan (8c). Following the general procedure, the reaction of pyrene-4,5-dione (100.8 mg, 0.43 mmol), 3-methylcyclohexanone (241.2 mg, 2.15 mmol) and iron(III) chloride (69.9 mg, 0.43 mmol) afforded 11-methylbenzo[d]pyreno[4,5-b]furan 8c as a white solid in 32% yield, mp 193–194 °C; IR (KBr) νmax 3052, 2917, 2852, 1593, 1512, 1430, 1356, 1307, 1250, 1172, 1107, 1054, 953, 894, 829, 751, 711 cm−1; 1H NMR (400 MHz, CDCl3) δ 8.74 (dd, J = 7.5, 1.1 Hz, 1H, ArH3), 8.65 (dd, J = 7.7, 1.0 Hz, 1H, ArH6), 8.25 (d, J = 8.0 Hz, 1H, ArH4), 8.17 (dd, J = 7.6, 0.9 Hz, 1H, ArH5), 8.18–8.02 (m, 5H, ArH1, H2, H7, H8 and H13), 7.56 (s, 1H, ArH10), 7.29 (d, J = 7.9 Hz, 1H, ArH12), 2.60 (s, 3H, CH3) ppm; 13C NMR (100 MHz, CDCl3) 13C NMR (100 MHz, CDCl3) δ 156.5 (C), 151.4 (C), 136.1 (C), 132.0 (C), 131.7 (C), 128.2 (CH), 127.6 (C), 127.4 (CH), 126.4 (CH), 126.1 (CH), 125.6 (CH), 124.8 (CH), 124.23 (CH), 124.20 (C), 123.3 (C), 122.6 (C), 121.5 (C), 121.34 (CH), 121.32 (CH), 118.6 (CH), 115.2 (C), 112.3 (CH), 22.0 (CH3) ppm; anal. calcd for C23H14O: C, 90.17; H, 4.61. Found: C, 89.96; H, 4.60%.
5.2.10. 12-Methylbenzo[d]pyreno[4,5-b]furan (8d). Following the general procedure, the reaction of pyrene-4,5-dione (100.2 mg, 0.43 mmol), 4-methylcyclohexanone (240.8 mg, 2.15 mmol) and iron(III) chloride (70.1 mg, 0.43 mmol) afforded 12-methylbenzo[d]pyreno[4,5-b]furan 8d as a white solid in 34% yield, mp 182–183 °C; IR (KBr) νmax 3045, 2920, 2860, 1596, 1454, 1373, 1310, 1217, 1175, 1109, 823, 761, 714 cm−1; 1H NMR (400 MHz, CDCl3 + CCl4) δ 8.72 (dd, J = 7.5, 1.1 Hz, 1H, ArH3), 8.64 (dd, J = 7.7, 1.1 Hz, 1H, ArH6), 8.17–8.14 (m, 2H, ArH4, H5), 8.10–8.00 (m, 5H, ArH1, H2, H7 and H8, H13), 7.62 (d, J = 8.3 Hz, 1H, ArH10), 7.28 (dd, J = 8.3, 1.1 Hz, 1H, ArH11), 2.63 (s, 3H, CH3) ppm; 13C NMR (100 MHz, CDCl3 + CCl4) δ 154.5 (C), 152.1 (C), 132.8 (C), 132.1 (C), 131.8 (C), 128.2 (CH), 127.9 (C), 127.4 (CH), 126.8 (CH), 126.4 (CH), 126.08 (CH), 126.05 (C), 125.8 (CH), 124.5 (C), 124.3 (CH), 122.8 (C), 122.0 (CH), 121.6 (C), 121.4 (CH), 118.8 (CH), 115.1 (C), 111.6 (CH), 22.0 (CH3) ppm; anal. calcd for C23H14O: C, 90.17; H, 4.61. Found: C, 89.91; H, 4.59%.
5.2.11. 5-Allyl-5-hydroxypyren-4(5H)-one (10). Pyrene-4,5-dione (501.2 mg, 2.16 mmol) was dissolved in 5 mL dry dimethylformamide and to this solution allyl bromide (406.3 mg, 3.35 mmol), indium powder (258.6 mg, 2.26 mmol), and sodium iodide (501.8 mg, 3.35 mmol) were added and stirred at rt for 4 h. The reaction mixture was quenched with 1 N HCl solution (1 mL) and the organic layer extracted using dichloromethane (30 mL). The DCM layer was washed with water (2 × 30 mL), brine solution (2 × 30 mL) and then dried over anhydrous sodium sulfate. The concentrated crude product was purified by column chromatography using silica (100–200 mesh) and increasing amounts of (2 to 5%) EtOAc in hexanes to afford 5-allyl-5-hydroxypyren-4(5H)-one 10 as a white solid in 72% yield; mp 152–153 °C; IR (KBr) νmax 3488, 3054, 3012, 2958, 2924, 2854, 1690, 1638, 1620, 1587, 1494, 1459, 1429, 1379, 1342, 1296, 1228, 1172, 1092, 1069, 1034, 997, 920, 871, 837, 780, 760, 723, 652 cm−1; 1H NMR (400 MHz, CDCl3 + CCl4) δ 8.24 (dd, J = 7.3, 1.2 Hz, 1H, ArH3), 8.12 (dd, J = 7.9, 1.2 Hz, 1H, ArH1), 7.93 (dd, J = 7.4, 1.2 Hz, 1H, ArH10), 7.83–7.66 (m, 5H, ArH2, H6, H7, H8 and H9), 5.68–5.58 (m, 1H, alkene CH), 5.01 (dd, J = 9.2, 0.9 Hz, 1H, alkene CH2), 4.84 (dd, J = 17.0, 1.7 Hz, 1H, alkene CH2), 4.20 (s, 1H, OH), 2.58–2.48 (m, 2H, allyl CH2) ppm; 13C NMR (100 MHz, CDCl3 + CCl4) δ 202.7 (C
O), 140.5 (C), 134.3 (CH), 131.9 (C), 131.3 (C), 131.2 (CH), 129.9 (C), 128.0 (C), 127.9 (CH), 127.7 (CH), 127.2 (CH), 127.0 (CH), 126.23 (CH), 126.18 (CH), 124.4 (CH), 123.6 (C), 119.9 (CH2), 80.5 (C), 49.7 (CH2) ppm; HRMS (ESI) calcd for C19H14O2Na [M + Na]+ 297.0891, found 297.0891; anal. calcd for C19H14O2: C, 83.19; H, 5.14. Found: C, 82.39; H, 5.15%.
5.2.12. (4R,5R)-4-Allyl-4,5-dihydropyrene-4,5-diol (11). 5-Allyl-5-hydroxypyren-4(5H)-one (100.3 mg, 0.37 mmol) was dissolved in 3 mL MeOH and cool to 0 °C and then sodium borohydride (28.1 mg, 0.74 mmol) was added under nitrogen atmosphere at 0 °C and stirred for 10 min and then the temperature was raised to room temperature and the mixture stirred at rt for 8 h. After the completion of the reaction (by TLC) MeOH was evaporated. Then the crude product was extracted using dichloromethane (30 mL). The DCM layer was washed with water (2 × 30 mL), brine solution (2 × 30 mL) and then dried over anhydrous sodium sulfate. The concentrated crude product was purified by recrystallization using hexane to afford (4R,5R)-4-allyl-4,5-dihydropyrene-4,5-diol 11 as a white solid in 78% yield; mp 113–114 °C; IR (KBr) νmax: 3421, 3052, 3012, 2925, 2854, 1638, 1592, 1430, 1291, 1239, 1169, 1100, 1058, 1000, 916, 864, 834, 810, 773, 729 cm−1; 1H NMR (400 MHz, CDCl3 + CCl4) δ 7.87 (d, J = 7.2 Hz, 1H, ArH6), 7.78 (d, J = 7.9 Hz, 2H, Ar H9, H10), 7.74–7.69 (m, 3H, ArH1, H3 and H8), 7.61–7.52 (m, 2H, ArH2, H7), 5.54–5.44 (m, 2H, H5, alkene CH), 4.99 (d, J = 10.1 Hz, 1H, alkene CH2), 4.92 (d, J = 17.1 Hz, 1H, alkene CH2), 3.76 (s, 1H, OH), 3.37 (s, 1H, OH), 2.78 (dd, J = 14.1, 8.8 Hz, 1H, allyl CH2), 2.28 (dd, J = 14.0, 6.0 Hz, 1H, allyl CH2) ppm; 13C NMR (100 MHz, CDCl3 + CCl4) δ 139.0 (C), 135.8 (C), 133.4 (CH), 131.2 (C), 130.9 (C), 127.15 (CH), 127.13 (CH), 126.78 (CH), 126.75 (CH), 126.7 (CH), 126.5 (CH), 125.9 (C), 125.7 (C), 123.1 (CH), 122.5 (CH), 119.6 (CH2), 77.9 (C), 76.5 (CH), 38.2 (CH2) ppm; HRMS (ESI) calcd for C19H16O2Na [M + Na]+ 299.1048, found 299.1045; anal. calcd for C19H16O2: C, 82.58; H, 5.84%. Found: C, 82.97; H, 5.79.
5.2.13. 10-(Iodomethyl)-10,11-dihydropyreno[4,5-b]furan (12). To a solution of (4R,5R)-4-allyl-4,5-dihydropyrene-4,5-diol (100.3 mg, 0.37 mmol) in tetrahydrofuran (2 mL), iodine (143.1 mg, 1.11 mmol) was added and stirred at rt for 12 h. After the completion of the reaction (by TLC) the THF was evaporated and the crude product was extracted using dichloromethane (20 mL). The organic layer was washed with hypo solution (2 × 20 mL), brine solution (2 × 20 mL) and then dried over anhydrous sodium sulfate. The concentrated crude product was purified by column chromatography using silica (100–200 mesh) and hexane as eluent to afford 10-(iodomethyl)-10,11-dihydropyreno[4,5-b]furan 12 as a white solid in 66% yield; mp 130–131 °C; IR (KBr) νmax: 3044, 2923, 2850, 1639, 1593, 1473, 1404, 1347, 1299, 1240, 1200, 1173, 1082, 1024, 973, 946, 887, 827, 761, 716, 624 cm−1; 1H NMR (400 MHz, CDCl3) δ 8.32 (d, J = 7.6 Hz, 1H, ArH3), 8.18 (d, J = 7.4 Hz, 1H, ArH6), 8.07–7.96 (m, 5H, ArH1, H2, H4, H5 and H7), 7.87 (d, J = 7.6 Hz, 1H, ArH8), 5.34–5.26 (m, 1H, H10), 3.83 (dd, J = 15.5, 7.6 Hz, 1H, H11), 3.67 (dd, J = 10.0, 4.7 Hz, 1H, H11), 3.55–3.45 (m, 2H, CH2); 13C NMR (100 MHz, CDCl3) δ 153.3 (C), 131.7 (C), 131.5 (C), 129.1 (C), 127.9 (CH), 127.4 (CH), 126.4 (CH), 125.8 (CH), 125.6 (CH), 125.1 (C), 122.9 (CH), 121.6 (C), 121.2 (C), 120.0 (CH), 119.3 (CH), 113.7 (C), 82.7 (CH), 36.1 (CH2), 9.6 (CH2) ppm; anal. calcd for C19H13IO: C, 59.40; H, 3.41. Found: C, 59.46; H, 3.42%.
5.2.14. 10-Methylpyreno[4,5-b]furan (9). To the solution of 10-(iodomethyl)-10,11-dihydropyreno[4,5-b]furan (100.2 mg, 0.26 mmol) in DMF (2 mL), 1,8-diazabicyclo[5,4,0]undec-7-ene (DBU) (39.6 mg, 0.26 mmol) was added and stirred at 50 °C for 4 h. After the completion of the reaction (by TLC) the DMF was evaporated and the crude product was extracted using dichloromethane (20 mL). The organic layer was washed with 0.01 N HCl solution (2 × 20 mL), brine solution (2 × 20 mL) and then dried over anhydrous sodium sulfate. The concentrated crude product was purified by crystallization using hexane and ethyl acetate to afford 10-methylpyreno[4,5-b]furan 9 as a white solid in 86% yield; mp 170–171 °C; IR (KBr) νmax: 1595, 1577, 1423, 1063, 1020, 937, 824, 800, 759, 711 cm−1; 1H NMR (400 MHz, CDCl3) δ 8.52 (dd, J = 7.6, 1.1 Hz, 1H, ArH3), 8.31 (dd, J = 7.6, 1.0 Hz, 1H, ArH6), 8.12–8.10 (m, 2H, ArH4, H5), 8.05–7.99 (m, 4H, ArH1, H2, H7 and H8), 6.99 (s, 1H, ArH11), 2.69 (s, 3H, CH3); 13C NMR (100 MHz, CDCl3) δ 154.6 (C), 148.9 (C), 132.1 (C), 131.9 (C), 128.0 (CH), 127.7 (CH), 126.9 (C), 126.1 (CH), 126.0 (CH), 124.2 (2 × CH), 123.1 (C), 122.7 (C), 122.2 (C), 121.8 (C), 121.0 (CH), 117.1 (CH), 103.0 (CH), 14.0 (CH3). ppm; anal. calcd for C19H12O: C, 89.04; H, 4.72. Found: C, 89.21; H, 4.69%.
5.2.15. (4R,5R)-5-Allyl-5-hydroxy-4,5-dihydropyren-4-yl 4-nitrobenzoate (13). (4R,5R)-4-Allyl-4,5-dihydropyrene-4,5-diol (101.2 mg, 0.37 mmol) was dissolved in 3 mL dichloromethane, then 4-nitrobenzoic acid (61.8 mg, 0.37 mmol), dicyclohexylcarbodiimide (DCC) (71.2 mg, 0.37 mmol) and 4-dimethylaminopyridine (DMAP) (45.3 mg, 0.37 mmol) were added and the mixture was stirred at rt for 12 h. Then the crude product was extracted using dichloromethane (30 mL). The DCM layer was washed with water (2 × 30 mL), brine solution (2 × 30 mL) and then dried over anhydrous sodium sulfate. The concentrated crude product was purified by crystallization using hexane and ethyl acetate to afford (4R,5R)-5-allyl-5-hydroxy-4,5-dihydropyren-4-yl 4-nitrobenzoate 13 as a light yellow color solid in 78% yield; mp 192–193 °C; IR (KBr) νmax: 3331, 2929, 2853, 1728, 1645, 1601, 1562, 1527, 1445, 1379, 1342, 1270,1218, 1103, 836, 809, 724 cm−1; 1H NMR (400 MHz, CDCl3) δ 8.41–8.35 (m, 4H, 4-nitrobenzoate ArH), 7.90–7.78 (m, 5H, ArH1, H3, H6, H9 and H10), 7.68–7.61 (m, 1H, ArH8), 7.59–7.54 (m, 2H, ArH2, H7), 6.97 (s, 1H, H4), 5.61–5.57 (m, 1H, alkene CH), 4.99 (d, J = 10.0 Hz, 1H, alkene CH2), 4.86 (d, J = 17.2 Hz, 1H, alkene CH2), 2.87–2.81 (m, 1H, allyl CH2), 2.62–2.57 (m, 2H, 1H in allyl CH2, OH) ppm; 13C NMR (100 MHz, CDCl3) δ 164.9 (C), 151.0 (C), 138.2 (C), 135.4 (C), 132.7 (CH), 131.9 (C), 131.3 (2 × C), 131.2 (2 × CH), 127.9 (CH), 127.8 (2 × CH), 127.3 (CH), 127.0 (CH), 126.7 (CH), 126.1 (C), 125.6 (C), 124.0 (2 × CH), 123.5 (CH), 122.8 (CH), 119.4 (CH2), 78.8 (CH), 76.7 (C), 39.6 (CH2) ppm; anal. calcd for C26H19NO5: C, 73.40; H, 4.50; N, 3.29. Found: C, 73.97; H, 4.48; N, 3.29%.
Crystal data for 13. Empirical formula, C26H19NO5; formula weight, 425.43; crystal color, habit: light yellow, needle; crystal system, triclinic; crystal dimensions, 0.35 × 025 × 0.20 mm3; lattice parameters, a = 8.6817(5) Å, b = 10.0211(6) Å, c = 12.9825(9) Å; α = 109.18(6), β = 97.30(5), γ = 99.85(5); V = 1030.43(12) A3; space group P
; Z = 2; Dcalcd = 1.381 g cm−3; F000 = 444; λ (Mo-Kα) = 0.7107 Å; R(I ≥ 2σ1) = 0.0547, wR2 = 0.1535.†
Acknowledgements
H. S. P. R thanks UGC, UGC-SAP, DST-FIST for financial assistance. V. S. thanks UGC for a fellowship. We thank CIF, Pondicherry University and IISc, Bengaluru for recording spectra. We thank Professor A. K. Mishra, IITM, Chennai for helpful discussions.
References
- R. J. Harvey, Polycyclic Aromatic Hydrocarbons: Chemistry and Carcinogenicity, Cambridge University Press, Cambridge, 1991 Search PubMed.
-
(a) G. Grimmer, Environmental carcinogens, polycyclic aromatic hydrocarbons: Chemistry, occurrence, biochemistry, carcinogenicity, CRC Press, United Kingdom, 1983 Search PubMed;
(b) H. Yamasaki, K. Kuwata and H. Miyamoto, Environ. Sci. Technol., 1982, 16, 189–194 CrossRef CAS.
- K. Mullen and U. Scherf, Organic Light-Emitting Devices: Synthesis, Properties and Applications, Wiley, Weinheim, 2006 Search PubMed.
-
(a) R. L. Danheiser, R. G. Brisbois, J. J. Kowalczyk and R. F. Miller, J. Am. Chem. Soc., 1990, 112, 3093–3100 CrossRef CAS;
(b) Z. Liu, X. Zhang and R. C. Larock, J. Am. Chem. Soc., 2005, 127, 15716–15717 CrossRef CAS PubMed.
- P. L. Majumder, S. Banerjee and S. Sen, Phytochemistry, 1996, 42, 847–852 CrossRef CAS.
- J. M. Schmidt, J. Mercure, G. B. Tremblay, M. Page, A. Kalbakji, M. Feher, R. Dunn-Dufault, M. G. Peter and P. R. Redden, J. Med. Chem., 2003, 46, 1408–1418 CrossRef CAS PubMed.
- F. Tanaka, N. Mase and C. F. Barbas III, J. Am. Chem. Soc., 2004, 126, 3692–3693 CrossRef CAS PubMed.
- H. S. P. Rao and V. Satish, RSC Adv., 2012, 2, 6773–6783 RSC.
- G. Takeuchi, H. Okazaki, T. Kito and Y. Kosugi, Anal. Sci., 1991, 7, 843–848 CrossRef CAS.
- Z. Zhao, S. Chen, J. W. Y. Lam, P. Lu, Y. Zhong, K. S. Wong, H. S. Kwok and B. Z. Tang, Chem. Commun., 2010, 46, 2221–2223 RSC.
- S. A. Ingale and F. Seela, J. Org. Chem., 2012, 77, 9352–9356 CrossRef CAS PubMed.
-
(a) K. Kalyanasundaram and J. K. Thomas, J. Am. Chem. Soc., 1977, 99, 2039–2044 CrossRef CAS;
(b) K. L. Chan, J. P. F. Lim, X. H. Yang, A. Dodabalapur, G. E. Jabbour and A. Sellinger, Chem. Commun., 2012, 48, 5106–5108 RSC.
- M. Uchimura, Y. Watanabe, F. Araoka, W. Watanabe, H. Takezoe and G. Konishi, Adv. Mater., 2010, 22, 4473–4478 CrossRef CAS PubMed.
- A. Baheti, C. P. Lee, K. R. Thomas and K. C. Ho, Phys. Chem. Chem. Phys., 2011, 13, 17210–17221 RSC.
- J. Xiao, B. Yang, J. I. Wong, Y. Liu, F. Wei, K. J. Tan, X. Teng, Y. Wu, L. Huang, C. Kloc, F. Boey, J. Ma, H. Zhang, H. Y. Yang and Q. Zhang, Org. Lett., 2011, 13, 3004–3007 CrossRef CAS PubMed.
- V. Nair, C. N. Jayan and S. Ros, Tetrahedron, 2001, 57, 9453–9459 CrossRef CAS.
- A. K. Yadav, B. K. Singh, N. Singh and R. P. Tripathi, Tetrahedron Lett., 2007, 48, 6628–6632 CrossRef CAS PubMed.
- N. J. Turro, V. Ramamurthy and J. C. Scaiano, Modern Molecular Photochemistry of Organic Molecules, University Science Books, Sausalito, CA, 2010, p. 912 Search PubMed.
-
(a) J. Xiao, B. Yang, J. I. Wong, Y. Liu, F. Wei, K. J. Tan, X. Teng, Y. Wu, L. Huang, C. Kloc, F. Boey, J. Ma, H. Zhang, H. Y. Yang and Q. Zhang, Org. Lett., 2011, 13, 3004–3007 CrossRef CAS PubMed;
(b) J. Xiao, H. Yang, Z. Yin, J. Guo, F. Boey, H. Zhang and Q. Zhang, J. Mater. Chem., 2011, 21, 1423–1427 RSC;
(c) J. C. Xiao, Y. Divayana, Q. Zhang, H. M. Doung, H. Zhang, F. Boey, X. W. Sun and F. Wudl, J. Mater. Chem., 2010, 20, 8167–8170 RSC;
(d) J. V. Morris, M. A. Mahaney and J. R. Huber, J. Phys. Chem., 1976, 80, 969–974 CrossRef CAS.
- Y. Niko, S. Kawauchi, S. Otsu, K. Tokumaru and G. Konishi, J. Org. Chem., 2013, 78, 3196–3207 CrossRef CAS PubMed.
- W. E. Acree Jr, S. A. Tucker and J. C. Fetzer, Polycyclic Aromat. Compd., 1991, 2, 75–105 CrossRef.
- J. Hu, D. Zhang and F. W. Harris, J. Org. Chem., 2005, 70, 707–708 CrossRef CAS PubMed.
- C. T. Tomas, B. P. Adriana and T. B. Maria, J. Org. Chem., 2005, 70, 6508–6511 CrossRef PubMed.
- L. Cires, V. Botomei, I. Humelnicu, H. Ofenberg and R. Neier, Rev. Roum. Chim., 2003, 48, 33–37 CAS.
- R. W. Paul, K. C. Sunil, M. V. John and H. M. Reginald, J. Chem. Soc., Chem. Commun., 1984, 9, 613–614 Search PubMed.
- B. J. Morrison and O. C. Musgrave, Phosphorus, Sulfur, Silicon Relat. Elem., 2002, 177, 2725–2744 CrossRef CAS.
Footnote |
† Electronic supplementary information (ESI) available. CCDC 929911 and 988268. For ESI and crystallographic data in CIF or other electronic format see DOI: 10.1039/c4ra02435d |
|
This journal is © The Royal Society of Chemistry 2014 |