DOI:
10.1039/C4RA02420F
(Communication)
RSC Adv., 2014,
4, 18930-18932
Tuneable radical cyclisations: a tin-free approach towards tricyclic and spirocyclic heterocycles via a common precursor†
Received
11th March 2014
, Accepted 1st April 2014
First published on 2nd April 2014
Abstract
A novel common precursor approach towards both tricyclic and spirocyclic heterocycles is described. Cyclisations are based on thiyl radical/isocyanide methodology and avoid the use of tin.
Tricyclic heterocycles and their related spirocyclic congeners are attractive synthetic targets due to their occurrence in natural products and their frequent use as therapeutics.1 Nearly all members of these two distinct chemical series possess potent and widely differing biological activities and have attracted significant attention from both synthetic and medicinal chemists.1 In particular, martinelline 1 and martinellic acid 2 – isolated from the roots of Martinella iquitosensis, have featured frequently in the literature, due to their unique occurrence in nature and their antibiotic/antagonistic activity towards several G-protein coupled receptors (GPCR) systems including bradykinin and muscarinic receptors.2 Amongst the non-natural product based compounds, the related tricyclic molecule – NVP-BEZ235 3, which is a dual inhibitor of PI3K/mTor pathway serves to highlight the anticancer potential of this class of compound.3 Related to their tricyclic counterparts, the spirooxindole heterocycles have also attracted significant attention, not least due to their structural complexity. This ranges from simple members such as horsfiline 4 and coerulescine 5,4 through to more substituted derivatives such as alstonisine 6 and spirotryprostatin A 7.5,6 There have been a significant number of synthetic efforts directed towards 7 and related derivatives due to its potent antinioplastic activity via inhibition of the cell cycle (Fig. 1).6
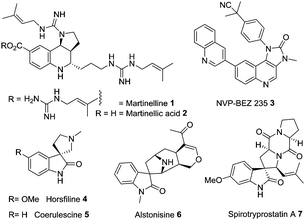 |
| Fig. 1 Spirocyclic and tricyclic heterocycles. | |
There have been several approaches to both these classes of compound using radical methodology as a result of the mild flexible and predictable conditions that can be used, preventing the need for protection/deprotection steps.7 In particular Takeda et al. have reported an attractive radical route to the core structure of Martinelline,7 whilst Beckwith and Storey have developed a route towards oxindoles via hydrogen atom abstraction using tributyltin hydride.8 Despite the large number of reports towards both classes of compound, many rely upon the use of organo-tin hydride reagents as a way of mediating these processes.7,8 An alternative method for the formation of nitrogen heterocycles that avoids the use of tin involves the addition of thiyl radicals to isocyanides, generating α-imidoyl radicals which can undergo cyclisation onto a pendant alkene/alkyne.9 However, there is no approach that allows for the synthesis of both chemical classes commencing from a common precursor. We now wish to disclose a novel tuneable approach to both tricyclic and spirocyclic heterocycles that is able to select for both classes via simple modification of the reaction conditions. The disconnection sequence towards both the pyrroloquinoline core 8 and the spirocyclic structure 9 from reaction of the precursor 11 is shown in detail (Scheme 1).
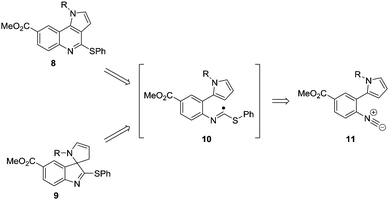 |
| Scheme 1 Disconnection of the tricyclic and spirocyclic cores. | |
We envisaged that under suitable reaction conditions the intermediate α-imidoyl radical derived from addition of a thiyl radical to the isocyanide 11, would undergo either 6-endo or 5-exo cyclisation to generate either the tricyclic core 8, or the spirocyclic structure 9 respectively. Our approach was inspired by the reports of Saegusa, Fukuyama and Rainier who had demonstrated precedent for 5-exo cyclisation, but there was limited evidence for successful obtention of the product of 6-endo cyclisation.9,10 The synthesis of the cyclisation precursor 11 commenced with iodination of methyl-4-aminobenzoate 12 to give the palladium coupling precursor 13 in 80% yield.11 Use of Suzuki–Miyaura conditions for the formation of amine 15 proved problematic with commercially available N-Boc-pyrrole-2-boronic acid 14. However, switching to the use of freshly prepared 14 following the procedure of Martina,12 amine 15 was obtained in 82% yield. Subsequent formylation with formic acetic anhydride,13 followed by dehydration with phosphorus oxychloride and diisopropylamine proceeded smoothly, resulting in isocyanide 11 in 79% yield (Scheme 2).14
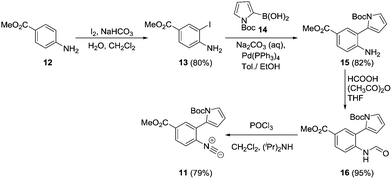 |
| Scheme 2 Synthesis of common cyclisation precursor 11. | |
With the cyclisation precursor 11 in hand, investigation of a range of conditions were explored in order to select for either 6-endo or 5-exo cyclisation in a thermodynamic or kinetic manner respectively and the results are outlined below (Table 1).
Table 1 Optimisation of reaction conditions for obtention of both tricyclic and spirocyclic heterocycles 8 and 9 respectivelya

|
Entry |
Concentration (M) |
AIBN (equiv.) |
PhSH (equiv.) |
Product ratio |
6-endo (8) |
5-exo (9) |
Ratios of 8 and 9 were determined from the 1H-NMR spectrum of the crude reaction mixture. All reactions were carried out at 80 °C in toluene for 2 hours by direct addition of reagents. |
1 |
0.2 |
0.3 |
5 |
100 |
0 |
2 |
0.1 |
0.3 |
5 |
100 |
0 |
3 |
0.05 |
0.3 |
5 |
56 |
44 |
4 |
0.03 |
0.3 |
5 |
11 |
89 |
5 |
0.05 |
0.5 |
5 |
48 |
52 |
6 |
0.05 |
0.1 |
5 |
100 |
0 |
7 |
0.05 |
1.0 |
5 |
42 |
58 |
8 |
0.05 |
1.5 |
5 |
13 |
87 |
9 |
0.05 |
1.5 |
50 |
0 |
100 |
10 |
0.05 |
0.1 |
50 |
94 |
6 |
Evaluation of the effects of reaction concentration (Table 1, entries 1–3) on the ratio of 8 and 9, showed that only the 6-endo product 8 was formed at higher concentrations, but under more dilute conditions (entries 3–4), the ratio of 8
:
9 changed to a more favorable 11
:
89 ratio of the two products. At this point, it was evident that the reaction was tunable and either the product of 5-exo or 6-endo addition could be readily selected. Simple variation of the amount of AIBN (Table 1, entries 4–8), whilst the amount of thiophenol and the reaction concentration were kept constant also insinuated that the radical cyclisation could be tuned easily. The kinetic 5-exo spirocycle was favoured at higher concentrations of AIBN, suggesting that the cyclised product is rapidly trapped and that the reaction itself is reversible. However, when the concentration of AIBN is low, the rate of cyclisation and subsequent reduction is slow and accordingly, the more stable thermodynamic 6-endo product predominates. To confirm the effects of the equivalents of AIBN on the reaction outcome, a large excess of thiophenol was used whilst varying the amount of AIBN (Table 1, entries 9 and 10). There was little difference on the reaction outcome when 50 equivalents of thiophenol were used, with selectivity easily tuned for either 5-exo or 6-endo addition.
With confirmation that the equivalents of AIBN and reaction concentration were critical, the reaction was carried out on a larger scale to fully isolate and characterise both products. As predicted, the tricyclic heterocycle 8 was obtained in 62% isolated yield when the reaction was carried out with 0.1 equivalents of AIBN. On repeating the reaction, but with conditions tuned for the synthesis of the spirocyclic product, compound 9 was isolated in 47% yield. A second product – 17 was also observed following purification, which corresponded to the double bond isomer, in 18% yield, from the parent compound 9. On contact with silica, the double bond undergoes a 1,3-hydride shift to give 17 and both products in a combined yield of 64% (Scheme 3).
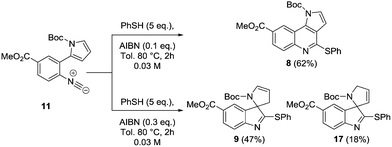 |
| Scheme 3 Selection for 6-endo or 5-exo cyclisation. | |
In summary, we have developed a novel approach towards the synthesis of pyrroloquinoline and spirocyclic heterocycles using tin-free radical methodology, starting from a common precursor. This short flexible reaction sequence will provide a rapid approach to structurally diverse compounds of significant interest and the application of this methodology is currently under active investigation using other heterocyclic motifs. Examination of their corresponding biological effects and the results obtained will be reported in due course.
Acknowledgements
This work was gratefully supported by the first grant scheme from the EPSRC [EP/J01544X/1], and a PhD studentship from UCL School of Pharmacy. We thank the EPSRC UK National Mass Spectrometry Facility at the Swansea University for spectroscopic services.
Notes and references
-
(a) A. I. S. Almeida, A. M. S. Silva and J. A. S. Cavaleiro, Synlett, 2011, 2955 CAS;
(b) Y. Hamada, Chem. Pharm. Bull., 2012, 60, 1 CrossRef CAS;
(c) H. Benakki, E. Colacino, C. André, F. Guenoun, J. Martinez and F. Lamaty, Tetrahedron, 2008, 64, 5949 CrossRef CAS PubMed;
(d) M. Nyerges, Heterocycles, 2004, 63, 1685 CrossRef CAS PubMed;
(e) M. L. Testa, L. Lamartina and F. Mingoia, Tetrahedron, 2004, 60, 5873 CrossRef CAS PubMed;
(f) T. Stalling and J. Martens, Synthesis, 2013, 355 CAS;
(g) Q. Zhang, M. Ding, Z. Cao, J. Zhang, F. Ding and K. Ke, Neurochem. Res., 2013, 38, 1661 CrossRef CAS PubMed;
(h) T. Li, H. Yin, C. Yao, X. Wang, B. Jiang, S. Tu and G. Li, Chem. Commun., 2012, 48, 11996 RSC;
(i) C. Marti and E. M. Carreira, Eur. J. Org. Chem., 2003, 2209 CrossRef CAS.
-
(a) K. M. Witherup, R. W. Ransom, A. C. Graham, A. M. Bernard, M. J. Salvatore, W. C. Lumma, P. S. Anderson, S. M. Pitzenberger and S. L. Varga, J. Am. Chem. Soc., 1995, 117, 6682 CrossRef CAS;
(b) M. K. Gurjar, S. Pal and A. V. R. Rao, Heterocycles, 1997, 45, 231 CrossRef CAS;
(c) B. B. Snider, Y. Ahn and B. M. Foxman, Tetrahedron Lett., 1999, 40, 3339 CrossRef CAS;
(d) C. J. Lovely and H. Mahmud, Tetrahedron Lett., 1999, 40, 2079 CrossRef CAS;
(e) O. Hara, K. Sugimoto and Y. Hamada, Tetrahedron, 2004, 60, 9381 CrossRef CAS PubMed;
(f) R. Le Goff, A. M. Lawson, A. Daïch and S. Comesse, Org. Biomol. Chem., 2013, 11, 1818 RSC;
(g) M. Hadden, M. Nieuwenhuyzen, D. Osborne, P. J. Stevenson, N. Thompson and A. D. Walker, Tetrahedron, 2006, 62, 3977 CrossRef CAS PubMed;
(h) C. Xia, L. Heng and D. Ma, Tetrahedron Lett., 2002, 43, 9405 CrossRef CAS.
- F. D. R. Stauffer and S.-M. Maira, et al., Bioorg. Med. Chem. Lett., 2008, 18, 1027 CrossRef CAS PubMed.
-
(a) S. Hong, M. Jung, Y. Park, M. W. Ha, C. Park, M. lee and H. Park, Chem.–Eur. J., 2013, 19, 9599 CrossRef CAS PubMed;
(b) E. Rajanarendar, S. Ramakrishna, K. G. Reddy, D. Nagaraju and Y. N. Reddy, Bioorg. Med. Chem. Lett., 2013, 23, 3954 CrossRef CAS PubMed;
(c) Y. Arun, G. Bhaskar, C. Balachandran, S. Ignacimuthu and P. T. Perumal, Bioorg. Med. Chem. Lett., 2013, 23, 1839 CrossRef CAS PubMed;
(d) J. A. Murphy, R. Tripoli, T. A. Khan and U. W. Mali, Org. Lett., 2005, 7, 3287 CrossRef CAS PubMed;
(e) M. Görmen, R. Le Goff, A. M. Lawson, A. Daïch and S. Comesse, Tetrahedron Lett., 2013, 54, 2174 CrossRef PubMed;
(f) A. Jossang, P. Jossang, H. A. Hadi, T. Sévenet and B. Bodo, J. Org. Chem., 1991, 56, 6527 CrossRef CAS;
(g) N. Anderton, P. A. Cockrum, S. M. Colegate, J. A. Edgar, K. Flower, I. Vit and R. I. Willing, Phytochemistry, 1998, 48, 437 CrossRef CAS;
(h) S. Bascop, J. Sapi, J. Laronze and J. Lévy, Heterocycles, 1994, 38, 725 CrossRef CAS.
- J. Yang, X. Z. Wearing, P. W. Le Quesne, J. R. Deschamps and J. M. Cook, J. Nat. Prod., 2008, 71, 1431 CrossRef CAS PubMed.
-
(a) C. Cui, H. Kakeya and H. Osada, Tetrahedron, 1996, 52, 12651 CrossRef CAS;
(b) A. P. Antonchick, H. Schuster, H. Bruss, M. Schürmann, H. Preut, D. Rauh and H. Waldmann, Tetrahedron, 2011, 67, 10195 CrossRef CAS PubMed;
(c) M. Cheng, H. Wang and L. Gong, Org. Lett., 2011, 13, 2418 CrossRef CAS PubMed;
(d) F. Y. Miyake, K. Yakushijin and D. A. Horne, Org. Lett., 2004, 6, 4249 CrossRef CAS PubMed;
(e) T. Onishi, P. R. Sebahar and R. M. Williams, Tetrahedron, 2004, 60, 9503 CrossRef CAS PubMed;
(f) S. Edmondson, S. J. Danishefsky, L. Sepp-Lorenzino and N. Rosan, J. Am. Chem. Soc., 1999, 121, 2147 CrossRef CAS.
-
(a) S. T. Hilton, T. C. T. Ho, G. Pljevaljcic and K. Jones, Org. Lett., 2000, 2, 2639 CrossRef CAS;
(b) C. Escolano and K. Jones, Tetrahedron, 2002, 58, 1453 CrossRef CAS;
(c) O. Miyata, A. Shirai, S. Yoshino, T. Nakabayashi, Y. Takeda, T. Kiguchi, D. Fukumoto, M. Ueda and T. Naito, Tetrahedron, 2007, 63, 10092 CrossRef CAS PubMed;
(d) T. Naito, Pure Appl. Chem., 2008, 80, 717 CrossRef CAS;
(e) T. C. T. Ho and K. Jones, Tetrahedron, 1997, 53, 8287 CrossRef CAS.
- A. L. J. Beckwith and J. M. D. Storey, J. Chem. Soc., Chem. Commun., 1995, 977 RSC.
-
(a) J. D. Rainier and A. R. Kennedy, J. Org. Chem., 2000, 65, 6213 CrossRef CAS PubMed;
(b) D. Nanni, G. Calestani, R. Leardini and G. Zanardi, Eur. J. Org. Chem., 2000, 707 CrossRef CAS;
(c) M. D. Bachi, A. Balanov and N. Bar-Ner, J. Org. Chem., 1994, 59, 7752 CrossRef CAS;
(d) K. C. Majumdar and P. Debnath, Tetrahedron, 2008, 64, 9799 CrossRef CAS PubMed;
(e) M. Lamberto and J. D. Kilburn, Tetrahedron Lett., 2008, 49, 6364 CrossRef CAS PubMed;
(f) M. Lamberto, D. F. Corbett and J. D. Kilburn, Tetrahedron Lett., 2004, 45, 8541 CrossRef CAS PubMed.
-
(a) T. Fukuyama and G. Liu, Pure Appl. Chem., 1997, 69, 501 CrossRef CAS;
(b) H. Tokuyama, M. Watanabe, Y. Hayashi, T. Kurokawa, G. Peng and T. Fukuyama, Synlett, 2001, 1403 CrossRef CAS;
(c) T. Saegusa, S. Kobayashi and Y. Ito, J. Org. Chem., 1970, 35, 2118 CrossRef CAS.
- A. G. Arvanitis and P. J. Gilligan, et al., J. Med. Chem., 1999, 42, 805 CrossRef CAS PubMed.
- S. Martina, V. Enkelmann, G. Wegner and A. Schlüter, Synthesis, 1991, 613 CrossRef CAS PubMed.
- T. G. Back, M. Parveez and J. E. Wulff, J. Org. Chem., 2003, 68, 2223 CrossRef CAS PubMed.
- R. Obrecht, R. Hermann and I. Ugi, Synthesis, 1985, 400 CrossRef CAS.
Footnote |
† Electronic supplementary information (ESI) available: Experimental details and characterisation of all compounds and copies of all spectra are available. See DOI: 10.1039/c4ra02420f |
|
This journal is © The Royal Society of Chemistry 2014 |