DOI:
10.1039/C4RA00902A
(Paper)
RSC Adv., 2014,
4, 24816-24819
Organocatalytic enantioselective aza-Henry reaction of ketimines derived from isatins: access to optically active 3-amino-2-oxindoles†
Received
31st January 2014
, Accepted 20th May 2014
First published on 21st May 2014
Abstract
An organocatalytic asymmetric aza-Henry reaction of ketimines derived from isatins with nitroalkanes has been achieved using Cinchona alkaloid organocatalysts. This method works efficiently with several ketimines to produce a good (up to 82%) yield of the corresponding 3-substituted 3-amino-2-oxindoles with a good (up to 89%) enantiomeric excess.
Introduction
An oxindole skeleton bearing a tetra-substituted stereogenic centre at the 3-position is a privileged heterocyclic framework present in many biologically active natural products and pharmaceutically active compounds.1 Among these compounds, 3-substituted 3-amino-2-oxindole2 has been recognised as a key structure because of its presence in a variety of natural products and biologically active compounds, such as the potent gastrin/CCK-B receptor antagonist AG-041R,3 the vasopressin VIb receptor antagonist SSR-14941533,4 and the anti-malarial drug candidate NITD609.5 In this context, the development of new methodologies for the synthesis of 3-amino-2-oxindole derivatives is of interest. In the past few years, several catalytic asymmetric addition reactions of isatin-derived ketimines,6 including the Mannich reaction,6a,b the Strecker reaction,6c–e the aza-Friedel–Crafts reaction6f,g and the Morita–Baylis–Hillman reaction,6h have been reported. However, the synthesis of the 3-amino-2-oxindole subunit via the aza-Henry reaction, which is a powerful and efficient method for the synthesis of nitrogen-containing molecules through carbon–carbon bond formation,7 has not been reported. The nitroamine adduct resulting from this addition reaction can easily be transformed into vicinal diamines8 and α-amino acids,9 which can serve as building blocks for the synthesis of other complex molecules.10
For the last five years, our group has been actively engaged in the development of enantioselective addition reactions of carbon nucleophiles to isatins and derivatives of isatins in the presence of bifunctional organocatalysts.11 In the work reported here, we have extended this line of research to the enantioselective addition of nitroalkanes to isatin-derived imines in the presence of bifunctional organocatalysts (Fig. 1). We report here the catalytic potential of bifunctional 6′-OH Cinchona alkaloids for the aza-Henry reaction of nitroalkanes with ketimines.12 We reasoned that enantioinduction can be achieved in this reaction through the synergistic activation of N-Boc ketimines and nitroalkanes by a bifunctional 6′-OH Cinchona alkaloid catalyst (Scheme 1).
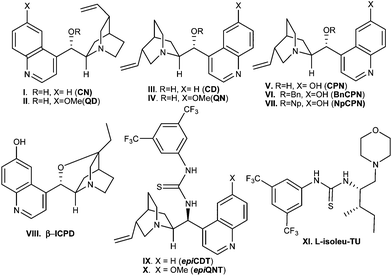 |
| Fig. 1 Screening of various organocatalysts for the asymmetric addition of nitroalkanes to ketimines. | |
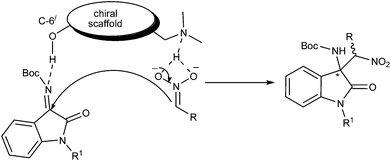 |
| Scheme 1 Proposed dual activation for the asymmetric aza-Henry reaction of nitroalkanes with ketimines. | |
Results and discussion
We initiated our investigation by performing the reaction of N-Boc ketimine (2a) with nitromethane (1a) in the presence of cinchonine (I, 20 mol%) as the catalyst in tetrahydrofuran (THF) and 4 Å molecular sieves at room temperature (Table 1). The reaction proceeded smoothly and provided the desired adduct 3a at 79% yield and 68% enantiomeric excess (ee) (Table 1, entry 1). Screening of other natural Cinchona alkaloids resulted in no further enhancement in the enantioinduction of 3a (Table 1, entries 2–4). The same reaction with the modified Cinchona alkaloid CPN (V) yielded 3a at 81% yield with a small enhancement of enantioselectivity (70% ee), suggesting a role for the 6′-OH group of quinoline in increasing the enantioselectivity (Table 1, entry 5). To determine the beneficial role of the 6′-OH group in terms of enantioinduction, the catalytic potential of the 9-OH blocked Cinchona alkaloids (BnCPN and NpCPN) was evaluated in the same reaction (Table 1, entries 6 and 7). Interestingly, BnCPN yielded the aza-Henry adduct 3a with the highest level of enantioselectivity (Table 1, entry 6). The quinidine-derived catalyst β-ICPD yielded product 3a at 62% yield with 7% ee (Table 1, entry 8). The model reaction carried out with Cinchona-derived thioureas (VIII–IX) resulted in a moderate yield and low enantioselectivity (Table 1, entries 9 and 10). No improvement was observed when using the amino-acid-derived catalyst XI (Table 1, entry 11). Lowering the temperature to −30 °C resulted in a prolonged reaction time with a lowering of the enantioselectivity of the adduct 3a (Table 1, entry 12).
Table 1 Optimization studya
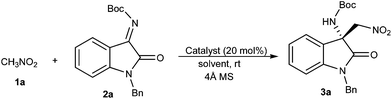
|
Entry |
Catalyst |
Solvent |
Time (h) |
Yieldb (%) |
eec (%) |
Reaction conditions: 0.1 mmol ketimine 2a, 0.25 mmol nitromethane, 4 Å molecular sieves (50 mg) and catalyst in dry solvent. Yield refers to isolated yield after column chromatography. Enantiomeric excess (ee) determined by chiral HPLC. Reaction performed at −30 °C. |
1 |
I |
THF |
24 |
79 |
68 |
2 |
II |
THF |
24 |
75 |
54 |
3 |
III |
THF |
24 |
78 |
−62 |
4 |
IV |
THF |
24 |
72 |
−34 |
5 |
V |
THF |
24 |
81 |
70 |
6 |
VI |
THF |
24 |
80 |
84 |
7 |
VII |
THF |
30 |
72 |
82 |
8 |
VIII |
THF |
36 |
62 |
−7 |
9 |
IX |
THF |
36 |
65 |
40 |
10 |
X |
THF |
36 |
69 |
40 |
11 |
XI |
THF |
36 |
65 |
38 |
12d |
VI |
THF |
36 |
65 |
56 |
13 |
VI |
CHCl3 |
24 |
78 |
81 |
14 |
VI |
DCM |
24 |
79 |
80 |
15 |
VI |
MTBE |
24 |
80 |
76 |
16 |
VI |
1,4-Dioxane |
24 |
76 |
74 |
17 |
VI |
Ethyl acetate |
24 |
74 |
79 |
18 |
VI |
Toluene |
24 |
81 |
83 |
Different solvents were also screened using 20 mol% of VI as a catalyst (Table 1, entries 4 and 13–18). After screening, THF was identified as the best solvent in terms of enantioinduction (Table 1, entry 6).
Once we had determined the optimum reaction conditions, the substrate scope of the BnCPN-catalyzed aza-Henry reaction was investigated by screening nitroalkanes with different derivatives of N-Boc ketimine. 5-Halo-N-benzylketimines (2b–2d) reacted efficiently with 1a to provide the corresponding 3-amino-2-oxindoles 3b–3d at 74–79% yield and 67–73% ee (Table 2, entries 2–4). Different derivatives of N-allyl ketimine gave the corresponding adducts 3e–3h at 73–78% yield with 70–89% ee (Table 2, entries 5–8). The N-protected isatins 2i–2j reacted smoothly with nitromethane to provide adducts 3i–3j at 70–71% yield and 75–79% ee (Table 2, entries 9 and 10). Ketimine 2k derived from 5-chloroisatin yielded product 3k at 68% yield and 74% ee (Table 2, entry 11). Nitroethane (1b) also reacted efficiently with ketimine 2i to give 3l at 72% yield, 72
:
28 dr and 80% ee of the major diastereomer (Table 2, entry 12). Nitropropane (1c), on reaction with ketimines (2i and 2h), gave the desired aza-Henry adducts 3m and 3n in good yield, but with low diastereomeric ratio and moderate enantiomeric excess (Table 2, entries 13 and 14).
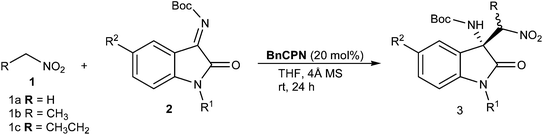
|
Entry |
1 |
2 (R1, R2) |
3 |
Yieldb (%) |
drc |
eed (%) |
Reaction conditions: 0.1 mmol ketimine 2, 0.25 mmol nitroalkane 1, 4 Å molecular sieve (50 mg) and catalyst VI (20 mol%) in dry THF. Yield refers to isolated yield after column chromatography. Diastereomeric ratio determined by HPLC after column purification of adducts (3l–3m). Enantiomeric excess (ee) determined by chiral HPLC. Values in parentheses are ee of minor diastereomer. |
1 |
1a |
2a (R1 = –CH2C6H5, R2 = H) |
3a |
80 |
— |
84 |
2 |
1a |
2b (R1 = –CH2C6H5, R2 = Cl) |
3b |
74 |
— |
67 |
3 |
1a |
2c (R1 = –CH2C6H5, R2 = Br) |
3c |
79 |
— |
73 |
4 |
1a |
2d (R1 = –CH2C6H5, R2 = I) |
3d |
77 |
— |
72 |
5 |
1a |
2e (R1 = –CH2CHCH2, R2 = H) |
3e |
73 |
— |
70 |
6 |
1a |
2f (R1 = –CH2CHCH2, R2 = Cl) |
3f |
76 |
— |
76 |
7 |
1a |
2g (R1 = –CH2CHCH2, R2 = Br) |
3g |
77 |
— |
73 |
8 |
1a |
2h (R1 = –CH2CHCH2, R2 = I) |
3h |
78 |
— |
89 |
9 |
1a |
2i (R1 = –CH2C(CH3)CH2, R2 = Cl) |
3i |
71 |
— |
75 |
10 |
1a |
2j (R1 = –CH2CHCHCH3, R2 = Cl) |
3j |
70 |
— |
79 |
11 |
1a |
2k (R1 = H, R2 = Cl) |
3k |
68 |
— |
74 |
12 |
1b |
2i (R1 = –CH2C(CH3)CH2, R2 = Cl) |
3l |
72 |
72 : 28 |
80(82)e |
13 |
1c |
2i (R1 = –CH2C(CH3)CH2, R2 = Cl) |
3m |
76 |
55 : 45 |
56(67)e |
14 |
1c |
2h (R1 = –CH2CHCH2, R2 = I) |
3n |
78 |
54 : 46 |
64(67)e |
To improve the synthetic utility, we studied the multicomponent version of this reaction by a combination of the aza-Wittig and aza-Henry reaction in a one-pot sequential protocol. The product 3a was isolated at 63% yield and 72% ee (Scheme 2).
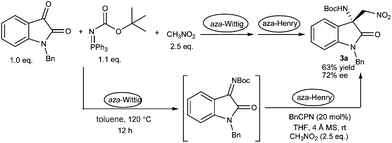 |
| Scheme 2 Tandem aza-Wittig/aza-Henry reaction. | |
The (R) absolute configuration of adduct 3 was unambiguously assigned on the basis of the single-crystal X-ray diffraction analysis of compound 3e (Fig. 2).13
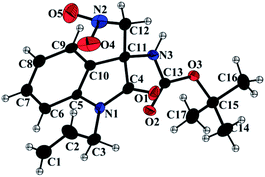 |
| Fig. 2 ORTEP diagram of molecule 3e at 30% probability. | |
We then designed some experiments to reveal the mechanism of the aza-Henry reaction catalyzed by the C6′-OH Cinchona alkaloid. The model reaction catalyzed by BnQN (XII), which has no free hydroxyl group, provided 3a at moderate yield (62%) and low enantioselectivity (27% ee), which suggests that the OH moiety has a role in enhancing the reactivity and selectivity of the reaction (Scheme 3). The natural Cinchona alkaloids (CN, CD, QD and QN), which have a free OH group at C9, gave products with a lower enantioselectivity than BnCPN/NpCPN with an OH group at C6′ (Table 1, entries 1–4, 6 and 7). This suggests the beneficial role of the C6′-OH group in term of enantioinduction. The catalyst XIII, which has no free amine moiety, failed to catalyze the model reaction, suggesting the role of the free amine moiety in the deprotonation of the nitroalkanes. These results show that a tertiary amine present in the catalyst is a prerequisite for this reaction to occur along, with the C6′-OH group, which provides a favourable orientation for high enantioinduction (Scheme 3).
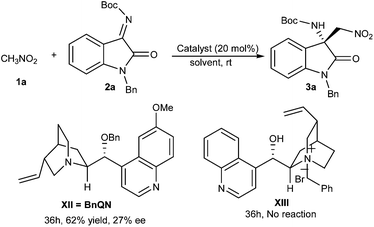 |
| Scheme 3 Elucidation of the bifunctional mode of activation. | |
On the basis of these experimental observations and the absolute configuration, a transition state involving a ternary complex between the catalyst and the substrates is proposed. The quinuclidine tertiary amine can deprotonate the α-proton of nitromethane, activating it for nucleophilic attack on the Re face of the ketimines, which is activated through hydrogen bonding with the C6′ OH group of the catalyst, thus providing the R enantiomer of the product (Scheme 4).
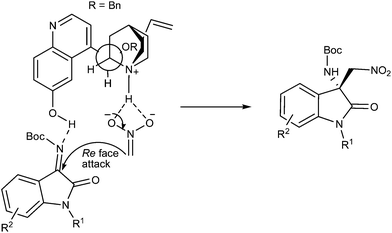 |
| Scheme 4 Proposed model for the transition state. | |
Conclusions
We have developed the first organocatalytic asymmetric aza-Henry reaction of ketimines derived from isatins and nitroalkanes using a C6′-OH Cinchona alkaloid catalyst. A variety of chiral 3-substituted 3-amino-2-oxindoles were successfully synthesized at good yields and with good enantioselectivity.
Acknowledgements
The authors are grateful for financial support from CSIR, India (Grant no. 02(0009)/11/EMR-II) to S.S.C. and R.A. fellowship to A.K. J.K. is thankful to the UGC, India for a Junior Research Fellowship. Financial support from the Department of Science and Technology (DST), India under FIST program and UGC, India under CAS-I to the Department of Chemistry is gratefully acknowledged.
Notes and references
- For selected reviews, see:
(a) C. V. Galliford and K. A. Scheidt, Angew. Chem., Int. Ed., 2007, 46, 8748 CrossRef CAS PubMed;
(b) C. Marti and E. M. Carreira, Eur. J. Org. Chem., 2003, 2209 CrossRef CAS;
(c) S. Hibino and T. Choshi, Nat. Prod. Rep., 2001, 18, 66 RSC;
(d) H. Lin and S. J. Danishefsky, Angew. Chem., Int. Ed., 2003, 42, 36–51 CrossRef CAS;
(e) B. S. Jensen, CNS Drug Rev., 2002, 8, 353 CrossRef CAS PubMed;
(f) B. M. Trost and M. K. Brennan, Synthesis, 2009, 3303 Search PubMed;
(g) F. Zhou, Y.-L. Liu and J. Zhou, Adv. Synth. Catal., 2010, 352, 1381 CrossRef CAS;
(h) K. Shen, X. Liu, L. Lin and X.-M. Feng, Chem. Sci., 2012, 3, 327 RSC;
(i) J. E. M. N. Klein and R. J. K. Taylor, Eur. J. Org. Chem., 2011, 6821 CrossRef CAS;
(j) A. Kumar and S. S. Chimni, RSC Adv., 2012, 2, 9748 RSC.
- For review on 3-amino-2-oxindoles, see:
(a) P. Chauhan and S. S. Chimni, Tetrahedron: Asymmetry, 2013, 24, 343 CrossRef CAS PubMed.
- M. Ochi, K. Kawasaki, H. Kataoka and Y. Uchio, Biochem. Biophys. Res. Commun., 2001, 283, 1118 CrossRef CAS PubMed.
-
(a) G. Decaux, A. Soupart and G. Vassart, Lancet, 2008, 371, 1624 CrossRef CAS;
(b) T. Shimazaki, M. Iijima and S. Chaki, Eur. J. Pharmacol., 2006, 543, 63 CrossRef CAS PubMed.
- M. Rottmann, C. McNamara, B. K. S. Yeung, M. C. S. Lee, B. Zou, B. Russell, P. Seitz, D. M. Plouffe, N. V. Dharia, J. Tan, S. B. Cohen, K. R. Spencer, G. E. González-Páez, S. B. Lakshminarayana, A. Goh, R. Suwanarusk, T. Jegla, E. K. Schmitt, H. P. Beck, R. Brun, F. Nosten, L. Renia, V. Dartois, T. H. Keller, D. A. Fidock, E. A. Winzeler and T. T. Diagana, Science, 2010, 329, 1175 CrossRef CAS PubMed.
-
(a) Q.-X. Guo, Y.-W. Liu, X.-C. Li, L.-Z. Zhong and Y.-G. Peng, J. Org. Chem., 2012, 77, 3589 CrossRef CAS PubMed;
(b) X.-B. Wang, T.-Z. Li, F. Sha and X.-Y. Wu, Eur. J. Org. Chem., 2014, 15, 739 CrossRef;
(c) Z.-Q. Qian, F. Zhou, T.-P. Du, B.-L. Wang, M. Ding, X.-L. Zhao and J. Zhou, Chem. Commun., 2009, 6753 RSC;
(d) Y.-L. Liu and J. Zhou, Chem. Commun., 2013, 49, 4421 RSC;
(e) Y.-L. Liu, F. Zhou, J. J. Cao, C.-B. Ji, M. Ding and J. Zhou, Org. Biomol. Chem., 2010, 8, 3847 RSC;
(f) S. Mouri, Z. Chen, H. Mitsunuma, M. Furutachi, S. Matsunaga and M. Shibasaki, J. Am. Chem. Soc., 2010, 132, 1255 CrossRef CAS PubMed;
(g) J. Feng, W. Yan, D. Wang, P. Li, Q. Sun and R. Wang, Chem. Commun., 2012, 48, 8003 RSC;
(h) F.-L. Hu, Y. Wei, M. Shi, S. Pindi and G. Li, Org. Biomol. Chem., 2013, 11, 1921 RSC;
(i) K. Shen, X. Liu, G. Wang, L. Lin and X.-M. Feng, Angew. Chem., Int. Ed., 2011, 50, 4684 CrossRef CAS PubMed;
(j) H. H. Jung, A. W. Buesking and J. A. Ellman, Org. Lett., 2011, 13, 3912 CrossRef CAS PubMed;
(k) W.-J. Yan, D. Wang, J.-C. Feng, P. Li and R. Wang, J. Org. Chem., 2012, 77, 3311 CrossRef CAS PubMed;
(l) J. George, B. Sridhar and B. V. Reddy, Org. Biomol. Chem., 2014, 12, 1595 RSC.
- For reviews on the catalytic asymmetric Henry reactions, see:
(a) Y. A. Casao, E. M. Lopez and R. P. Herrera, Symmetry, 2011, 3, 220 CrossRef PubMed;
(b) E. M. Lopez, P. Merino, T. Tejero and R. P. Herrera, Eur. J. Org. Chem., 2009, 2401 CrossRef;
(c) C. Palomo, M. Oiarbide and A. Laso, Eur. J. Org. Chem., 2007, 2561 CrossRef CAS;
(d) J. Boruwa, N. Gogoi, P. P. Saikia and N. C. Barua, Tetrahedron: Asymmetry, 2006, 17, 3315 CrossRef CAS PubMed;
(e) C. Palomo, M. Oiarbide and A. Mielgo, Angew. Chem., Int. Ed., 2004, 43, 5442 CrossRef CAS PubMed;
(f) F. A. Luzzio, Tetrahedron, 2001, 57, 915–945 CrossRef CAS;
(g) H. Xie, Y. Zhang, S. Zhang, X. Chen and W. Wang, Angew. Chem., Int. Ed., 2011, 50, 11773 CrossRef CAS PubMed;
(h) A. Parra, R. Alfaro, L. Marzo, A. M. Carrasco, J. L. G. Ruano and J. Aleman, Chem. Commun., 2012, 48, 9759 RSC;
(i) L. Liu, S. Zhang, F. Xue, G. Lou, H. Zhang, S. Ma, W. Duan and W. Wang, Chem.–Eur. J., 2011, 17, 7791 CrossRef CAS PubMed.
-
(a) A. K. Beck and D. Seebach, Chem. Ber., 1991, 124, 2897 CrossRef CAS;
(b) M. A. Poupart, G. Fazal, S. Goulet and L. T. Mar, J. Org. Chem., 1999, 64, 1356 CrossRef CAS;
(c) A. G. M. Barrett and C. D. Spilling, Tetrahedron Lett., 1988, 29, 5733 CrossRef CAS;
(d) D. H. Lloyd and D. E. Nichols, J. Org. Chem., 1986, 51, 4294 CrossRef CAS.
- H. W. Pinnick, Org. React., 1990, 38, 655 CAS.
-
(a) G. Calderari and D. Seebach, Helv. Chim. Acta, 1985, 68, 1592 CrossRef CAS;
(b) R. Tamura, A. Kamimura and N. Ono, Synthesis, 1991, 423 CrossRef CAS PubMed;
(c) V. Meyer and C. Wurster, Ber. Dtsch. Chem. Ges., 1873, 6, 1168 CrossRef;
(d) M. J. Kamlet, L. A. Kaplan and J. C. Dacons, J. Org. Chem., 1961, 26, 4371 CrossRef CAS;
(e) T. Mukaiyama and T. Hoshino, J. Am. Chem. Soc., 1960, 82, 5339 CrossRef CAS.
-
(a) A. Kumar and S. S. Chimni, Beilstein J. Org. Chem., 2014, 10, 929 CrossRef;
(b) A. Kumar, J. Kaur, P. Chauhan and S. S. Chimni, Chem.–Asian J., 2014, 9, 1305 CrossRef CAS PubMed;
(c) J. Kaur, A. Kumar and S. S. Chimni, Tetrahedron Lett., 2014, 55, 2138 CrossRef CAS PubMed;
(d) A. Kumar and S. S. Chimni, Eur. J. Org. Chem., 2013, 4780 CrossRef CAS;
(e) A. Kumar and S. S. Chimni, Tetrahedron, 2013, 69, 5197 CrossRef CAS PubMed;
(f) P. Chauhan and S. S. Chimni, Asian J. Org. Chem., 2013, 2, 533 CrossRef;
(g) P. Chauhan and S. S. Chimni, Chem.–Eur. J., 2010, 26, 7709 CrossRef PubMed.
- During the review process of manuscript, two independent related studies reported in the literature
(a) Y.-H. Wang, Y.-L. Liu, Z.-Y. Cao and J. Zhou, Asian J. Org. Chem., 2014, 3, 429 CrossRef CAS;
(b) T. Arai, E. Matsumura and H. Masu, Org. Lett., 2014, 16, 2768 CrossRef CAS PubMed.
- ESI.†.
Footnote |
† Electronic supplementary information (ESI) available. See DOI: 10.1039/c4ra00902a |
|
This journal is © The Royal Society of Chemistry 2014 |