DOI:
10.1039/C4RA00559G
(Paper)
RSC Adv., 2014,
4, 17832-17839
Magnetically recoverable and reusable CuFe2O4 nanoparticle-catalyzed synthesis of benzoxazoles, benzothiazoles and benzimidazoles using dioxygen as oxidant†
Received
22nd January 2014
, Accepted 1st April 2014
First published on 3rd April 2014
Abstract
A green and efficient strategy for the synthesis of benzoxazoles, benzothiazoles and benzimidazoles has been developed by using inexpensive, readily available, dioxygen-stable and recyclable CuFe2O4 as the nanocatalyst, and o-substituted aminobenzene and various aldehydes as the starting materials. The CuFe2O4 nanoparticles are dioxygen insensitive and easily recoverable with an external magnet from the reaction medium. The catalyst can be reused ten times without significant loss of catalytic activity.
Introduction
N-Heterocycles widely occur in natural products, biologically active molecules and organic materials, and they play an important role in the design and discovery of new drugs. Therefore, the development of novel, efficient and practical methods for heterocycle synthesis is an important goal in modern organic synthesis. Benzoxazoles, benzothiazoles and benzimidazoles are now known to have a wide range of useful biological and medicinal properties, such as polymers,1 enzyme inhibitors,2 antibacterial,3 anticancer agents,4 antimicrobial,5 anti-inflammatory,6 antiparkinson,7 antioxidants,8 and antiallergy activities,9 so their synthesis is attracting much attention. Consequently, a lot of significant methods for the synthesis of these important building blocks have been developed. The conventional methods for the synthesis of these important compounds typically involve two approaches. One is the metal-catalyzed intramolecular condensation of o-haloanilides or their analogues (Scheme 1a).10 The second approach mainly involves the coupling of o-substituted aminoaromatics with carboxylic acids or acyl halides (Scheme 1b).11 Despite these methods have made various successes, the uneasily available precursors and undesired by-products limit their wide applications. Therefore, a more effective process is needed.
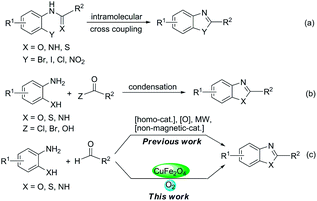 |
| Scheme 1 Strategies for the synthesis of benzoxazoles, benzothiazoles and benzimidazoles. | |
Aldehydes are important and common building blocks, and they are easily prepared from readily available materials. Using o-substituted aminoaromatics and aldehyde as the starting materials to construct these heterocycles have caught considerable attention (Scheme 1c). In this regard, several examples of aerobic oxidation pathways have been reported with various transition metal salts or oxidants, such as ZrOCl2·8H2O,12 Pd(OAc)2/O2,13 CuCl2,14 Sc(OTf)3,15 Yb(OTf)3,16 FeCl3·6H2O,17 HAuCl4·4H2O/O2,18 DDQ,19 PhI(OAc)2,20 H2O2–HCl,21 TEMPO,22 activated carbon23 and cyanide.24 However, in some cases, most of these methods might suffer from some drawbacks such as undesirable stoichiometric oxidants, noble transition metal catalyst, long reaction times, toxic reaction reagents, and residual metal catalysts in the end products, which should still impede their applications for the heterocycle synthesis on a large scale.
In recent years, heterogeneous catalysts have attracted much attention in organic transformations due to their interesting reactivity as well as for economic and environmental reasons. A large number of recyclable supported catalytic systems have been developed (Scheme 1c).25–27 For example, Satyanarayana's group reported an efficient method for the synthesis of benzoxazoles using silica-supported sodium hydrogen sulphate.25 Recently, Kidwai and co-workers reported an efficient CuO nanoparticles catalyzed coupling aromatic or heteroaromatic aldehydes with 2-aminophenol to construct benzoxazoles in the presence of K2CO3 in MeOH.26 Gracefully excellent as these works could be, the small size of catalyst particles might often make their separation and recyclization difficult, especially the catalysis efficiency of the recovered catalysts might be somewhat reduced through a filtration step.
Recently, magnetic nanoparticles (MNPs) have been extensively used in organic transformations owing to their easy preparation, large surface area ratio, low toxicity, high dispersion property in organic solvents, facile separation by using an external magnetic force and without the need for filtration step.28 Very recently, Brahmachari et al. reported an elegant work for the synthesis of 2-substituted benzimidazoles and quinoxalines using MnFe2O4 as a heterogeneous catalyst.29 However, challenges still remain, magnetic-nanoparticles catalyzed direct coupling of 2-aminophenol or 2-aminobenzenethiol with aldehyde has not been reported to date. Additionally, we couldn't get benzoxazoles and benzothiazoles under the standard conditions reported by lit.28 We therefore set out to look for an improved catalyst system for this transformation and to demonstrate the generality with which it can be employed. Recently, for economical and environmental reasons, there is an increasing demand for the use of dioxygen as an oxidant for many oxidation reactions, because water is the only waste when dioxygen is used as oxidant. Inspired by the utilization of magnetically separable CuFe2O4 nanoparticles as a powerful and excellent catalyst for many organic transformations.30 Herein, we report a simple, practical and efficient method for the synthesis of substituted benzoxazoles, benzothiazoles and benzimidazoles by using the cheap, dioxygen-stable CuFe2O4 nanoparticles as a magnetically recoverable catalyst and O2 as a green oxidant (Table 1).
Table 1 Magnetic CuFe2O4-catalyzed condensation of 2-aminophenol (1a) with 4-methylbenzaldehyde (2b) leading to 2-p-tolylbenzo[d]oxazole (3b): optimization of conditionsa

|
Entry |
Solvent |
Temp. [°C] |
Yieldb [%] |
Reaction conditions: 2-aminophenol (1a) (0.75 mmol), benzaldehyde (2b) (0.5 mmol), catalyst (0.1 mmol), solvent (0.5 mL) under oxygen atmosphere.
Isolated yield.
Without catalyst.
In the presence of catalyst (0.005 mmol).
In the presence of catalyst (0.0125 mmol).
In the presence of catalyst (0.05 mmol).
Under air conditions.
|
1 |
H2O |
100 |
0 |
2 |
EtOH |
80 |
0 |
3 |
CH3CN |
80 |
0 |
4 |
THF |
80 |
0 |
5 |
Toluene |
110 |
94 |
6 |
— |
110 |
45 |
7 |
Toluene |
110 |
Tracec |
8 |
Toluene |
110 |
30d |
9 |
Toluene |
110 |
45e |
10 |
Toluene |
110 |
65f |
11 |
Toluene |
25 |
0 |
12 |
Toluene |
60 |
0 |
13 |
Toluene |
90 |
58 |
14 |
Toluene |
100 |
78 |
15 |
Toluene |
110 |
76g |
Results and discussion
The CuFe2O4 nanoparticles were synthesis by reaction of Cu2+ ions and Fe3+ in alkaline condition according to the literature procedure31 and characterized by X-ray diffraction (Fig. 1), TEM spectrum (Fig. 2) and EDX spectrum (Fig. 1, ESI†). The diffraction patterns of all the peaks are in agreement with the standard XRD pattern (JCPDS34-0425). The XRD pattern of the CuFe2O4 nanoparticles before reaction (Fig. 1a) and after reaction in the 3th cycle (Fig. 1b) showed that the Cu remains in the +2 oxidation state. Additionally, the TEM and SEM images analysis of the recovered nano CuFe2O4 particles revealed that the morphology of the catalyst remains unchanged, even after three cycles under dioxygen atmosphere (Fig. 2 and 3).
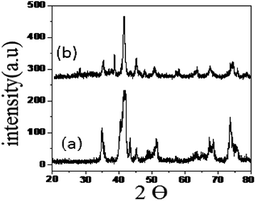 |
| Fig. 1 (a) XRD spectrum of native CuFe2O4 catalyst. (b) XRD spectrum of reused CuFe2O4 catalyst after 3th cycle. | |
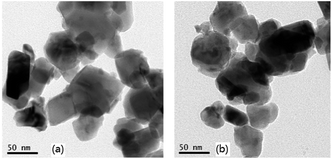 |
| Fig. 2 TEM image of the fresh CuFe2O4 nanoparticles (a). TEM image of the CuFe2O4 nanoparticles after 3th cycle (b). | |
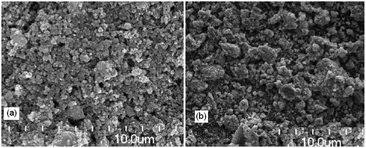 |
| Fig. 3 SEM images of CuFe2O4 nanoparticles before (a) and after (b) 3th cycle. | |
At first, 2-aminophenol (1a) and 4-methylbenzaldehyde (2b) were chosen as the model substrates to optimize reaction conditions including the amount of catalysts, solvents and reaction temperatures under oxygen atmosphere. First, five solvents were tested in the presence of 0.2 equiv. of CuFe2O4 nanoparticles, and toluene gave the highest yield (94%), interestingly, without solvent, also afforded the target product (3a) in 45% yield (entries 1–6). Furthermore, when the amount of the catalyst was changed from 20 mol% to 1 mol%, the reaction yield decreased, providing only 30% yield (entries 5, 8–10). Control experiments confirmed that the product was not formed in the absence of the catalyst (entries 8). We attempted different temperature (compare entries 5 and 11–14), and 110 °C was optimal. The reaction under air also gave a good yield (76%) (entry 15). Therefore, the standard reaction condition for the CuFe2O4-catalyzed synthesis of benzoxazole derivatives is as follows: 20 mol% of CuFe2O4 as the catalyst and toluene as the solvent under oxygen atmosphere.
We then investigated the scope of CuFe2O4-catalyzed reactions of substituted 2-aminophenol (1) with benzaldehyde (2) under the optimized catalytic conditions determined above. As shown in Table 2, most of the examined substrates provided good to excellent yields. For the substituted 2-aminophenol and benzaldehyde the electronic effect of the substituted groups including electron-rich, -neutral, and -deficient substituents did not display evident difference in reactivity as shown in Table 2. For the substituted benzaldehyde, the substrates containing nitro groups gave moderate yields. Under a similar condition, the methodology was extended to the synthesis of various benzothiazoles and benzimidazoles from other building blocks like o-aminothiophenol and o-phenyldiamine. The results are also summarized in Table 2. The CuFe2O4-catalyzed domino reactions could tolerate some functional groups such as alkyl group, C–F bonds, C–Cl bonds, C–Br bonds, and nitro groups. Although aromatic aldehyde showed high reactivity, unfortunately, aliphatic ones were poor substrates, they are suitable for 2-aminobenzenethiol but unactive for o-aminophenols or o-phenylenediamines. In order to explain this, two control experiments were performed under the standard conditions as shown in Scheme 2. Treatment of (E)-2-(butylideneamino)phenol and (E)-2-(butylideneamino)benzenethiol under the standard conditions provided 2-propylbenzo[d]thiazole (3x) in 97% yield and no 2-propylbenzo[d]oxazole was observed. This result indicate an weaker nucleophilicity of the hydroxyl group under the CuFe2O4 catalyzed conditions.
Table 2 Magnetic CuFe2O4-catalyzed synthesis of benzoxazoles, benzothiazoles and benzimidazolesa
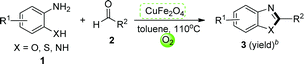
|
Reaction conditions: o-substituted aminobenzene (1) (0.75 mmol), benzaldehyde (2) (0.5 mmol), catalyst (0.1 mmol), solvent (0.5 mL) under oxygen atmosphere.
Isolated yield.
|
|
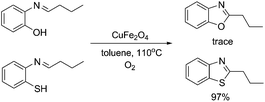 |
| Scheme 2 Control experiments. | |
We also studied the recyclability of the catalyst. For this, we investigated the CuFe2O4-catalyzed cyclization of 2-aminophenol (1a) with benzaldehyde (2a) under the optimized conditions. After completion of the reaction, the reaction mixture was cooled to room temperature, and the catalyst was magnetically separated from the reaction mixture, washed with ethanol and dried at 100 °C for 2 h and then used directly for further catalytic reactions. The catalyst could be reused ten times without significant loss in catalytic activity (average yields in 90%).
Finally, we investigated the formation mechanism of benzoxazole derivatives. As shown in Scheme 3, when one equivalent of TEMPO (2,2,6,6-tetramethyl-1-piperidinyloxy, a well known radical-capturing species) was added to the reaction system, no significant difference was observed in the yield, ruling out the presence of radicals during the reaction.
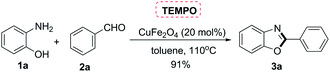 |
| Scheme 3 Reactions of o-aminophenol with benzaldehyde in the presence of TEMPO under the optimized reaction condition. | |
On the basis of these results above, a possible mechanism is thus proposed as illustrated in Scheme 4. Initially, CuFe2O4 nanoparticles could act as a Lewis acid which activates the aldehyde and promote the imine (A) formation. The resulting imine could further undergo the ring closure by the intramolecular attack of hydroxyl, sulfhydryl and amino group on the C
N double bond to give intermediate (B) that subsequently could proceed the aromatization by aerial oxidation under the reaction conditions so as to afford the desired products (3).
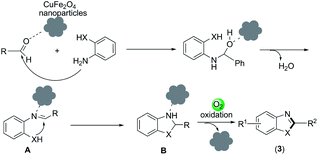 |
| Scheme 4 Possible mechanism for CuFe2O4-catalyzed synthesis of benzoxazoles, benzimidazoles and benzothiazoles. | |
Experimental section
General
All reagents and solvents were obtained from commercial suppliers and used without further purification. All reagents were weighed and handled in air at room temperature. Flash chromatography was performed on silica gel (200–300 mesh). 1H and 13C NMR data were recorded at 400 and 100 MHz on a BRUKER 400 spectrometer. Chemical shifts (δ) are expressed in parts per million (ppm) coupling constants (J) are in Hz. Proton and carbon magnetic resonance spectra (1H NMR and 13C NMR) were recorded using tetramethylsilane (TMS) in the solvent of CDCl3 as the internal standard (1H NMR: TMS at 0.00 ppm, CDCl3 at 7.24 ppm; 13C NMR: CDCl3 at 77.0 ppm) or were recorded using tetramethylsilane (TMS) in the solvent of DMSO-D6 as the internal standard (1H NMR: TMS at 0.00 ppm, DMSO at 2.50 ppm; 13C NMR: DMSO at 40.0 ppm).
General procedure for CuFe2O4-catalyzed synthesis of benzoxazoles, benzimidazoles and benzothiazoles: (3)
A 25 mL Schlenk tube equipped with a magnetic stirring bar was charged with CuFe2O4 nanoparticles (0.05 mmol, 12 mg), substituted o-substituted aminobenzene (1) (0.5 mmol). The tube was evacuated twice and backfilled with oxygen, and toluene (0.5 mL) was added to the tube under oxygen atmosphere. The tube was sealed with a balloon and then the mixture was allowed to stir under oxygen atmosphere at 110 °C for 24 h. After completion of the reaction, the resulting solution was cooled to room temperature, and the solvent was removed with the aid of a rotary evaporator. The residue was purified by column chromatography on silica gel using petroleum ether–ethyl acetate as eluent to provide the desired product (3).
2-Phenylbenzo[d]oxazole (3a)32.
Eluent petroleum ether–ethyl acetate (30
:
1). White solid. mp 94–95 °C (lit.32 94–96 °C) (petroleum ether–ethyl acetate = 40
:
1, Rf = 0.3). 1H NMR (CDCl3, 400 MHz, ppm) δ 8.29 (d, 2H, J = 7.6 Hz), 7.81 (d, 1H, J = 3.3 Hz), 7.61 (d, 1H, J = 3.4 Hz), 7.62–7.54 (m, 3H), 7.38 (d, 2H, J = 6.0 Hz) 13C NMR (CDCl3, 200 MHz, ppm) δ 163.1, 150.8, 142.1, 131.5, 128.9, 127.6, 127.2, 125.1, 124.6, 120.0, 110.6. ESI-MS [M + H]+m/z 196.4.
2-p-Tolylbenzo[d]oxazole (3b)33.
Eluent petroleum ether–ethyl acetate (30
:
1). White solid. mp 116–117 °C (lit.33 118–119 °C) (petroleum ether–ethyl acetate = 40
:
1, Rf = 0.3). 1H NMR (CDCl3, 400 MHz, ppm) δ 8.15 (d, 2H, J = 8.0 Hz), 7.78 (d, 1H, J = 4.8 Hz), 7.59 (d, 1H, J = 5.2 Hz), 7.37–7.34 (m, 4H), 2.46(s, 3H) 13C NMR (CDCl3, 200 MHz, ppm) δ 163.3, 150.7, 142.2, 142.1, 129.7, 127.6, 124.9, 124.5, 124.4, 119.9, 110.5, 21.7. ESI-MS [M + H]+m/z 210.4.
2-(4-Chlorophenyl)benzo[d]oxazole (3c)34.
Eluent petroleum ether–ethyl acetate (30
:
1). White solid. mp 157–159 °C (lit.34 155–156 °C) (petroleum ether–ethyl acetate = 30
:
1, Rf = 0.4). 1H NMR (CDCl3, 400 MHz, ppm) δ 8.21 (d, 2H, J = 4.0 Hz), 7.79 (d, 1H, J = 6.4 Hz), 7.60 (d, 1H, J = 4.0 Hz), 7.57–7.41 (m, 2H), 7.36–7.36 (m, 2H). 13C NMR (CDCl3, 200 MHz, ppm) δ 162.1, 150.8, 142.0, 137.8, 129.3, 128.9, 125.7, 124.7, 120.1, 124.8, 110.6. ESI-MS [M + H]+m/z 230.5.
2-(4-Bromophenyl)benzo[d]oxazole (3d)35.
Eluent petroleum ether–ethyl acetate (15
:
1). White solid. mp 158–160 °C (lit.35 156–158 °C) (petroleum ether–ethyl acetate = 30
:
1, Rf = 0.3). 1H NMR (CDCl3, 400 MHz, ppm) δ 8.14 (d, 2H, J = 8.8 Hz), 7.79 (d, 1H, J = 3.2 Hz), 7.69 (d, 2H, J = 8.8 Hz), 7.60 (d, 1H, J = 5.6 Hz), 7.42–7.37 (m, 2H). 13C NMR (CDCl3, 200 MHz, ppm) δ 162.2, 150.8, 142.0, 132.3, 129.0, 126.3, 125.4, 124.8, 120.1, 110.7. ESI-MS [M + H]+m/z 273.5, 275.4.
5-Methyl-2-p-tolylbenzo[d]oxazole (3e)36.
Eluent petroleum ether–ethyl acetate (30
:
1). White solid. mp 104–105 °C (lit.36 103–104 °C) (petroleum ether–ethyl acetate = 20
:
1, Rf = 0.4). 1H NMR (CDCl3, 400 MHz, ppm) δ 8.15 (d, 2H, J = 8.4 Hz), 7.56 (d, 1H, J = 0.8 Hz), 7.45 (d, 1H, J = 8.4 Hz), 7.35–7.33 (m, 2H), 7.16 (m, 1H), 2.52 (s, 1H), 2.46(s, 1H). 13C NMR (CDCl3, 200 MHz, ppm) δ 163.4, 148.9, 142.4, 142.9, 127.5, 126.0, 109.9, 21.7, 21.6. ESI-MS [M + H]+m/z 223.6.
6-Methyl-2-p-tolylbenzo[d]oxazole (3f)37.
Eluent petroleum ether–ethyl acetate (30
:
1). White solid. mp 90–92 °C (lit.37 89–91 °C) (petroleum ether–ethyl acetate = 20
:
1, Rf = 0.4). 1H NMR (CDCl3, 400 MHz, ppm) δ 8.14 (d, 2H, J = 8.4 Hz), 7.64 (d, 1H, J = 8.4 Hz), 7.39–7.33 (m, 3H), 7.17 (d, 1H, J = 8.4 Hz), 2.52 (s, 1H), 2.46(s, 1H). 13C NMR (CDCl3, 200 MHz, ppm) δ 162.8, 151.0, 141.8, 140.0, 135.3, 129.6, 127.4, 125.7, 124.6, 119.2, 110.7, 21.8, 21.6. ESI-MS [M + H]+m/z 223.7.
2-(4-Bromophenyl)-5-methylbenzo[d]oxazole (3g).
Eluent petroleum ether–ethyl acetate (20
:
1). White solid. mp 190–191 °C (petroleum ether–ethyl acetate = 20
:
1, Rf = 0.5). 1H NMR (CDCl3, 400 MHz, ppm) δ 8.12 (d, 2H, J = 8.4 Hz), 7.66 (d, 1H, J = 8.4 Hz), 7.57 (s, 1H), 7.46 (d, 1H, J = 8.4 Hz), 7.18 (d, 1H, J = 8.4 Hz), 2.50 (s, 3H). 13C NMR (CDCl3, 200 MHz, ppm) δ 162.2, 149.0, 128.9, 126.5, 126.3, 126.1, 120.0, 110.0, 21.6. ESI-MS [M + H]+m/z 287.2, 289.1. HR-MS: m/z calcd for C14H11BrON: 288.0024; found: 288.0027, 290.0006. IR: max(thin film) (cm−1) = 3078, 2919, 1592, 1548, 1455, 1398, 1068, 838, 796, 7283.
(R)-2-(3-Nitrophenyl)benzo[d]oxazole (3h)38.
Eluent petroleum ether–ethyl acetate (20
:
1). White solid. mp 210–212 °C (lit.38 210 °C) (petroleum ether–ethyl acetate = 30
:
1, Rf = 0.4).1H NMR (CDCl3, 400 MHz, ppm) δ 9.10 (s, 1H), 8.59 (d, 1H, J = 7.6 Hz), 8.40 (d, 1H, J = 7.6 Hz), 7.83 (d, 1H, J = 8.4 Hz), 7.77–7.64 (m, 2H), 7.43 (d, 2H, J = 8.4). 13C NMR (CDCl3, 200 MHz, ppm) δ 160.6, 150.9, 148.7, 141.8, 133.0, 130.1, 129.5, 128.6, 126.1, 125.1, 122.5, 120.5, 110.9. ESI-MS [M + H]+m/z 240.6.
6-Methyl-2-phenylbenzo[d]oxazole (3i)39.
Eluent petroleum ether–ethyl acetate (20
:
1). White solid. mp 100–101 °C (lit.39 99–102 °C) (petroleum ether–ethyl acetate = 20
:
1, Rf = 0.4). 1H NMR (CDCl3, 400 MHz, ppm) δ 8.26 (d, 2H, J = 5.6 Hz), 7.58–7.52 (m, 4H), 7.47 (d, 1H, J = 8.4 Hz), 7.17 (d, 1H, J = 8.4 Hz), 2.5 (s, 1H). 13C NMR (CDCl3, 200 MHz, ppm) δ 163.1, 149.0, 142.3, 134.4, 131.4, 128.9, 127.6, 127.3, 126.2, 119.9, 109.9, 21.6. ESI-MS [M + H]+m/z 209.6.
5-Methyl-2-phenylbenzo[d]oxazole (3j)39.
Eluent petroleum ether–ethyl acetate (20
:
1). White solid. mp 112–114 °C (lit.39 112–114 °C) (petroleum ether–ethyl acetate = 30
:
1, Rf = 0.4). 1H NMR (CDCl3, 400 MHz, ppm) δ 8.29–8.26 (m, 2H), 7.58 (s, 1H), 7.56–7.53 (m, 3H), 7.62–7.55 (m, 3H), 7.19(d, 1H, J = 7.6 Hz), 7.17 (d, 1H, J = 6.0 Hz), 2.51 (s, 1H). 13C NMR (CDCl3, 200 MHz, ppm) δ 163.1, 149.0, 142.3, 134.4, 131.4, 128.9, 127.6, 127.3, 126.2, 119.9, 109.9, 21.6. ESI-MS [M + H]+m/z 210.2.
2-(4-Fluorophenyl)-6-methylbenzo[d]oxazole (3k)40.
Eluent petroleum ether–ethyl acetate (20
:
1). White solid. mp 112–113 °C (lit.40 113–116 °C) (petroleum ether–ethyl acetate = 20
:
1, Rf = 0.5). 1H NMR (CDCl3, 400 MHz, ppm) δ 8.27–8.24 (m, 2H), 7.56 (s, 1H), 7.45 (d, 1H, J = 8.4 Hz), 7.24–7.17 (m, 3H), 2.5 (s, 3H). 13C NMR (CDCl3, 200 MHz, ppm) δ 160.0, 163.5, 162.2, 149.0, 142.3, 134.5, 129.8, 129.7, 126.3, 123.7, 123.6, 119.9, 116.3, 116.0, 109.9, 21.5. ESI-MS [M + H]+m/z 227.7.
2-(4-Chlorophenyl)-6-methylbenzo[d]oxazole (3l)36.
Eluent petroleum ether–ethyl acetate (20
:
1). White solid. mp 149–152 °C (lit.36 151–152 °C) (petroleum ether–ethyl acetate = 20
:
1, Rf = 0.4).1H NMR (CDCl3, 400 MHz, ppm) δ 8.17 (d, 1H, J = 8.4 Hz), 7.55 (s, 1H), 7.51–7.44 (m, 3H), 7.17 (s, 1H, J = 8.0 Hz), 2.50 (s, 3H). 13C NMR (CDCl3, 200 MHz, ppm) δ 162.1, 148.9, 142.2, 137.6, 134.6, 129.2, 128.8, 120.0, 110.0, 21.5. ESI-MS [M + H]+m/z 243.6.
2-(4-Bromophenyl)-6-methylbenzo[d]oxazole (3m).
Eluent petroleum ether–ethyl acetate (30
:
1). White solid. mp 171–173 °C (petroleum ether–ethyl acetate = 20
:
1, Rf = 0.5).1H NMR (CDCl3, 400 MHz, ppm) δ 8.10 (d, 2H, J = 8.4 Hz), 7.68–7.64 (m, 3H), 7.39 (s, 1H), 7.19 (d, 1H, J = 8.0 Hz), 2.53 (s, 3H). 13C NMR (CDCl3, 200 MHz, ppm) δ 161.6, 151.0, 139.8, 135.9, 132.2, 128.9, 126.3, 126.0, 125.9, 119.4, 110.8, 21.9. ESI-MS [M + H]+m/z 288.2, 290.4. HR-MS: m/z calcd for C14H11BrON: 288.0024; found: 288.0026, 290.0010. IR: max(thin film) (cm−1) = 3086, 2920, 1612, 1588, 1548, 1480, 1397, 1068, 835, 813, 726.
2-(4-Chlorophenyl)-5-methylbenzo[d]oxazole (3n)35.
Eluent petroleum ether–ethyl acetate (20
:
1). White solid. mp 149–151 °C (lit.35 150–151 °C) (petroleum ether–ethyl acetate = 20
:
1, Rf = 0.4). 1H NMR (CDCl3, 400 MHz, ppm) δ 8.15 (d, 2H, J = 8.4 Hz), 7.55(s, 1H), 7.49 (d, 2H, J = 8.4 Hz), 7.44 (d, 1H, J = 8.4 Hz), 7.16 (d, 1H, J = 8.2 Hz), 2.50 (s, 3H). 13C NMR (CDCl3, 200 MHz, ppm) δ 162.1, 149.0, 142.1, 137.6, 134.6, 129.2, 128.8, 126.5, 125.8, 120.0, 109.9, 21.5. ESI-MS [M + H]+m/z 243.7.
6-Chloro-2-phenylbenzo[d]oxazole (3o)41.
Eluent petroleum ether–ethyl acetate (20
:
1). White solid. mp 108–110 °C (lit.41 107–108 °C) (petroleum ether–ethyl acetate = 20
:
1, Rf = 0.4). 1H NMR (CDCl3, 400 MHz, ppm) δ 8.25 (d, 2H, J = 5.6 Hz), 7.69 (d, 1H, J = 8.4 Hz), 7.62 (s, 1H), 7.61–7.53 (m, 3H), 7.34 (d, 1H, J = 5.6 Hz). 13C NMR (CDCl3, 200 MHz, ppm) δ 163.7, 150.9, 140.9, 131.8, 130.7, 129.0, 127.7, 126.7, 125.3, 120.5, 111.3. ESI-MS [M + H]+m/z 229.5.
6-Chloro-2-(4-fluorophenyl)benzo[d]oxazole (3p)42.
Eluent petroleum ether–ethyl acetate (20
:
1). White solid. mp 130–131 °C (lit.42 132–133 °C) (petroleum ether–ethyl acetate = 20
:
1, Rf = 0.5). 1H NMR (CDCl3, 400 MHz, ppm) δ 8.26–8.21 (m, 2H), 7.67 (d, 1H, J = 8.4 Hz), 7.59 (s, 1H), 7.36 (d, 1H, J = 8.4 Hz), 7.33–7.20 (m, 2H). 13C NMR (CDCl3, 200 MHz, ppm) δ 166.2, 163.7, 162.8, 150.9, 140.8, 130.7, 130.0, 129.9, 125.4, 123.1, 123.0, 120.4, 116.4, 116.2, 111.2. ESI-MS [M + H]+m/z 247.7.
6-Chloro-2-(4-chlorophenyl)benzo[d]oxazole (3q)41.
Eluent petroleum ether–ethyl acetate (20
:
1). White solid. mp 149–150 °C (lit.41 148–149 °C) (petroleum ether–ethyl acetate = 20
:
1, Rf = 0.5). 1H NMR (CDCl3, 400 MHz, ppm) δ 8.18–8.15 (m, 2H), 7.68 (d, 1H, J = 8.4 Hz), 7.60 (s, 1H), 7.54–7.50 (m, 2H), 7.36 (d, 1H, J = 8.4 Hz). 13C NMR (CDCl3, 200 MHz, ppm) δ 162.7, 150.9, 140.8, 138.1, 131.0, 129.4, 128.9, 125.5, 125.2, 120.6, 111.3. ESI-MS [M + H]+m/z 263.4.
2-(4-Bromophenyl)-6-chlorobenzo[d]oxazole (3r)42.
Eluent petroleum ether–ethyl acetate (20
:
1). White solid. mp 168–170 °C (lit.42 168–170 °C) (petroleum ether–ethyl acetate = 30
:
1, Rf = 0.4). 1H NMR (CDCl3, 400 MHz, ppm) δ 8.09 (d, 2H, J = 8.4 Hz), 7.69–7.66 (m, 3H), 7.59 (s, 1H), 7.36 (d, 2H, J = 8.4 Hz). 13C NMR (CDCl3, 200 MHz, ppm) δ 162.8, 151.0, 140.8, 132.3, 131.0, 129.0, 126.6, 125.6, 125.5, 120.6, 111.3. ESI-MS [M + H]+m/z 308.4, 310.3.
6-Chloro-2-p-tolylbenzo[d]oxazole (3s)43.
Eluent petroleum ether–ethyl acetate (20
:
1). White solid. mp 126–128 °C (lit.43 126–127 °C) (petroleum ether–ethyl acetate = 30
:
1, Rf = 0.4). 1H NMR (CDCl3, 400 MHz, ppm) δ 8.12 (d, 2H, J = 8.0 Hz), 7.67 (d, 2H, J = 8.4 Hz), 7.58 (s, 1H), 7.34–7.33 (m, 3H), 2.46 (s, 3H). 13C NMR (CDCl3, 200 MHz, ppm) δ 164.0, 150.9, 142.5, 141.0, 130.4, 129.7, 127.6, 125.2, 123.9, 120.3, 111.2, 21.7. ESI-MS [M + H]+m/z 243.7.
2-Phenylbenzo[d]thiazole (3t)43.
Eluent petroleum ether–ethyl acetate (10
:
1). White solid. mp 113–115 °C (lit.43 112–114 °C) (petroleum ether–ethyl acetate = 30
:
1, Rf = 0.4). 1H NMR (CDCl3, 400 MHz, ppm) δ 8.14–8.11 (m, 3H), 7.93 (d, 2H, J = 8.0 Hz), 7.55–7.51 (m, 4H), 7.42 (t, 1H, J = 8.0 Hz). 13C NMR (CDCl3, 200 MHz, ppm) δ 168.1, 154.2, 135.1, 133.6, 131.0, 129.1, 127.6, 126.3, 125.2, 123.3, 121.7. ESI-MS [M + H]+m/z 211.7.
2-p-Tolylbenzo[d]thiazole (3u)44.
Eluent petroleum ether–ethyl acetate (30
:
1). White solid. mp 83–85 °C (lit.44 85–86 °C) (petroleum ether–ethyl acetate = 30
:
1, Rf = 0.3). 1H NMR (CDCl3, 400 MHz, ppm) δ 8.09 (d, 1H, J = 8.0 Hz), 8.01 (d, 2H, J = 8.0 Hz), 7.92 (d, 2H, J = 8.0 Hz), 7.51 (m, 1H), 7.41 (t, 1H, J = 8.0 Hz), 7.37–7.31 (m, 2H), 2.45 (s, 3H). 13C NMR (CDCl3, 200 MHz, ppm) δ 168.2, 154.2, 141.4, 135.0, 131.0, 129.7, 127.5, 126.2, 125.0, 123.1, 121.6, 21.5. ESI-MS [M + H]+m/z 225.6.
2-(4-Bromophenyl)benzo[d]thiazole (3v)45.
Eluent petroleum ether–ethyl acetate (10
:
1). White solid. mp 130–131 °C (lit.45 132–133 °C) (petroleum ether–ethyl acetate = 30
:
1, Rf = 0.4). 1H NMR (CDCl3, 400 MHz, ppm) δ 8.10 (d, 1H, J = 8.0 Hz), 7.97 (d, 2H, J = 8.0 Hz), 7.92 (d, 2H, J = 8.0 Hz), 7.51 (m, 1H), 7.65 (t, 2H, J = 8.0 Hz), 7.54 (t, 1H, J = 8.0 Hz), 7.43 (t, 1H, J = 8.0 Hz). 13C NMR (CDCl3, 200 MHz, ppm) δ 166.7, 154.1, 135.1, 132.6, 132.2, 128.9, 126.5, 125.5, 125.4, 123.3, 121.7. ESI-MS [M + H]+m/z 290.4, 291.3.
2-(4-Chlorophenyl)benzo[d]thiazole (3w)46.
Eluent petroleum ether–ethyl acetate (10
:
1). White solid. mp 116–117 °C (lit.46 115–116 °C) (petroleum ether–ethyl acetate = 30
:
1, Rf = 0.4). 1H NMR (CDCl3, 400 MHz, ppm) δ 8.08 (d, 1H, J = 8.0 Hz), 8.01 (d, 2H, J = 8.0 Hz), 7.92 (d, 1H, J = 7.6 Hz), 7.52 (t, 1H, J = 7.6 Hz), 7.45 (t, 2H, J = 8.4 Hz), 7.39 (t, 1H, J = 7.6 Hz). 13C NMR (CDCl3, 200 MHz, ppm) δ 166.6, 154.1, 137.0, 135.1, 132.1, 129.3, 128.7, 126.5, 125.4, 123.3, 121.7. ESI-MS [M + H]+m/z 245.5.
2-Propylbenzo[d]thiazole (3x).
Eluent petroleum ether–ethyl acetate (10
:
1). Yellow oil (petroleum ether–ethyl acetate = 30
:
1, Rf = 0.2). 1H NMR (CDCl3, 400 MHz, ppm) δ 7.98 (d, 1H, J = 8.4 Hz), 7.83 (d, 2H, J = 8.4 Hz), 7.43 (t, 1H, J = 8.0 Hz), 7.31 (t, 1H, J = 8.0 Hz), 3.09 (t, 2H, J = 7.6 Hz), 1.91 (dt, 2H, J = 7.2 Hz), 1.05 (t, 2H, J = 7.2 Hz). 13C NMR (CDCl3, 200 MHz, ppm) δ 172.1, 153.3, 135.2, 125.9, 124.6, 122.5, 121.5, 36.3, 23.1, 13.7. ESI-MS [M + H]+m/z 177.6. HR-MS: m/z calcd for C10H11NS: 178.0690; found: 178.0687. IR: max(thin film) (cm−1) = 3414, 2968, 1617, 1560, 1518, 1455, 1405, 1381, 1068, 879, 759.
2-Butylbenzo[d]thiazole (3y).
Eluent petroleum ether–ethyl acetate (10
:
1). yellow oil (petroleum ether–ethyl acetate = 30
:
1, Rf = 0.2). 1H NMR (CDCl3, 400 MHz, ppm) δ 7.90 (d, 1H, J = 8.0 Hz), 7.85 (d, 2H, J = 8.0 Hz), 7.47 (t, 1H, J = 6.8 Hz), 7.34 (t, 1H, J = 6.8 Hz), 7.45 (t, 2H, J = 8.4 Hz), 7.39 (t, 1H, J = 7.6 Hz), 3.14 (t, 2H, J = 7.2 Hz), 1.88 (t, 2H, J = 6.8 Hz), 1.50 (t, 2H, J = 7.2 Hz), 0.99 (t, 3H, J = 7.2 Hz). 13C NMR (CDCl3, 200 MHz, ppm) δ 172.4, 153.3, 135.1, 125.9, 124.6, 122.5, 121.5, 34.1, 31.8, 22.3, 13.8. ESI-MS [M + H]+m/z 191.6. HR-MS: m/z calcd for C11H15NS: 192.0847; found: 192.0850. IR: max(thin film) (cm−1) = 3436, 3306, 2957, 2871, 1630, 1561, 1520, 1456, 1436, 1381, 1127, 855, 758.
2-(4-Fluorophenyl)benzo[d]thiazole (3z)47.
Eluent petroleum ether–ethyl acetate (10
:
1). White solid. mp 98–99 °C (lit.47 100–102 °C) (petroleum ether–ethyl acetate = 20
:
1, Rf = 0.4). 1H NMR (CDCl3, 400 MHz, ppm) δ 8.16–8.07 (m, 3H), 8.05 (d, 2H, J = 8.0 Hz), 7.57 (t, 1H, J = 7.2 Hz), 7.55–7.38 (m, 3H). 13C NMR (CDCl3, 200 MHz, ppm) δ 172.4, 153.3, 135.1, 125.9, 124.6, 122.5, 121.5, 34.1, 31.8, 22.3, 13.8. ESI-MS [M + H]+m/z 229.5.
(R)-2-(2-Bromo-5-methoxyphenyl)benzo[d]thiazole (3a′).
Eluent petroleum ether–ethyl acetate (10
:
1). White solid. mp 252–254 °C (petroleum ether–ethyl acetate = 20
:
1, Rf = 0.3).1H NMR (DMSO-D6, 400 MHz, ppm) δ 8.16 (d, 2H, J = 8.4 Hz), 7.97 (d, 2H, J = 7.6 Hz), 7.64–7.54 (m, 3H), 7.47 (t, 1H, J = 8.0 Hz), 7.47 (d, 1H, J = 8.4 Hz), 3.90 (s, 3H). 13C NMR (DMSO-D6, 200 MHz, ppm) δ 165.6, 158.9, 152.6, 136.2, 135.1, 134.8, 126.3, 125.5, 123.6, 121.5, 118.2, 116.6, 112.5, 55.7. ESI-MS [M + H]+m/z 319.5, 321.4. HR-MS: m/z calcd for C14H11BrNOS: 319.9745; found: 319.9745, 321.9725. IR: max(thin film) (cm−1) = 3064, 3005, 2939, 2834, 1593, 1564, 1484, 1380, 1316, 853, 759, 603.
(R)-2-(2-Bromo-4-fluorophenyl)benzo[d]thiazole (3b′).
Eluent petroleum ether–ethyl acetate (10
:
1). White solid. mp 246–248 °C (petroleum ether–ethyl acetate = 5
:
1, Rf = 0.4). 1H NMR (DMSO-D6, 400 MHz, ppm) δ 8.15 (d, 2H, J = 8.0 Hz), 8.06 (t, 1H, J = 8.8 Hz), 7.97 (d, 2H, J = 8.0 Hz), 7.58–7.45 (m, 3H), 7.20 (t, 1H, J = 8.8 Hz). 13C NMR (DMSO-D6, 200 MHz, ppm) δ 164.6, 164.3, 161.8, 152.7, 136.1, 133.6, 133.5, 131.0, 130.9, 126.4, 125.6, 123.6, 122.6, 122.5, 121.4, 121.2, 115.2, 115.0. ESI-MS [M + H]+m/z 307.5, 309.4. HR-MS: m/z calcd for C13H8BrFNS: 307.9545; found: 307.9557, 308.9547. IR: max(thin film) (cm−1) = 3084, 2971, 2900, 1614, 1490, 1464, 1241, 1042, 857, 750.
2-p-Tolyl-1H-benzo[d]imidazole (3c′)48.
Eluent petroleum ether–ethyl acetate (3
:
1). White solid. mp 276–277 °C (lit.48 275–276 °C) (petroleum ether–ethyl acetate = 5
:
1, Rf = 0.3).1H NMR (DMSO-D6, 400 MHz, ppm) δ 12.83 (s, br, 1H), 8.07 (d, 2H, J = 8.4 Hz), 7.58 (m, 2H), 7.36 (d, 2H, J = 8.0 Hz), 7.20 (d, 2H, J = 8.5 Hz). 13C NMR (DMSO-D6, 200 MHz, ppm) δ 168.2, 151.8, 140.0, 130.0, 127.9, 126.9, 21.4. ESI-MS [M + H]+m/z 209.6.
2-(4-Chlorophenyl)-1H-benzo[d]imidazole (3d′)49.
Eluent petroleum ether–ethyl acetate (3
:
1). White solid. mp 300–301 °C (lit.49 303 °C) (petroleum ether–ethyl acetate = 5
:
1, Rf = 0.4).1H NMR (DMSO-D6, 400 MHz, ppm) δ 13.00 (s, br, 1H), 8.20 (d, 2H, J = 8.8 Hz), 7.69–7.54 (m, 4H), 7.23 (t, 2H, J = 8.0 Hz), 7.20 (d, 2H, J = 8.5 Hz). 13C NMR (DMSO-D6, 200 MHz, ppm) δ 150.6, 144.2, 135.5, 134.9, 129.5, 128.6, 123.2, 122.3, 119.4, 111.9. ESI-MS [M + H]+m/z 228.7.
2-(4-Bromophenyl)-1H-benzo[d]imidazole (3e′)50.
Eluent petroleum ether–ethyl acetate (5
:
1). White solid. mp 300–301 °C (lit.50 299–300 °C) (petroleum ether–ethyl acetate = 5
:
1, Rf = 0.5).1H NMR (DMSO-D6, 400 MHz, ppm) δ 13.00 (s, br, 1H), 8.12 (d, 2H, J = 8.8 Hz), 7.77 (d, 2H, J = 6.8 Hz), 7.61 (m, 2H), 7.22 (d, 2H, J = 8.8 Hz). 13C NMR (DMSO-D6, 200 MHz, ppm) δ 150.6, 135.5, 132.4, 129.9, 123.7, 122.3, 119.4, 111.9. ESI-MS [M + H]+m/z 272.7, 274.6.
6-Bromo-2-(4-fluorophenyl)-1H-benzo[d]imidazole (3f′).
Eluent petroleum ether–ethyl acetate (5
:
1). White solid. mp 320–321 °C (petroleum ether–ethyl acetate = 5
:
1, Rf = 0.4).1H NMR (DMSO-D6, 400 MHz, ppm) δ 13.10 (s, br, 1H), 8.23–8.21(m, 2H), 7.86–7.49 (m, 2H), 7.43–7.34 (m, 3H). 13C NMR (DMSO-D6, 200 MHz, ppm) δ 164.9, 165.2, 134.6, 129.4, 125.5, 116.5, 114.4, 113.6. ESI-MS [M + H]+m/z 290.4, 292.3. HR-MS: m/z calcd for C13H9BrFN2: 290.9933; found: 290.9936, 292.9911. IR: max(thin film) (cm−1) = 3445, 2965, 1628, 1600, 1464, 1430, 1383, 1233, 915, 805, 734.
6-Bromo-2-(4-chlorophenyl)-1H-benzo[d]imidazole (3g′).
Eluent petroleum ether–ethyl acetate (2
:
1). White solid. mp 336–337 °C (petroleum ether–ethyl acetate = 1
:
1, Rf = 0.5). 1H NMR (DMSO-D6, 400 MHz, ppm) δ 13.17 (s, br, 1H), 8.17 (d, 2H, J = 8.8 Hz), 7.79 (m, 1H), 7.62–7.55 (m, 3H), 7.34 (d, 1H, J = 8.4 Hz). 13C NMR (DMSO-D6, 200 MHz, ppm) δ 152.1, 132.5, 129.4, 129.0.125.8, 125.3, 124.1, 121.8, 121.1, 114.5, 113.6. ESI-MS [M + H]+m/z 306.4, 308.3. HR-MS: m/z calcd for C13H9BrClN2: 306.9638; found: 306.9634, 308.9604. IR: max(thin film) (cm−1) = 3271, 2900, 1629, 1507, 1393, 1241, 1015, 1233, 879, 732.
Conclusions
In conclusion, we have developed a simple, green and efficient strategy for the synthesis of benzoxazoles, benzothiazoles and benzimidazoles. The couplings were performed using readily available starting materials (o-substituted aminobenzene and various aldehydes), magnetically separable and reusable CuFe2O4 nanoparticles as the catalyst and dioxygen as the green oxidant, importantly, organic oxidizing agents, strong acids or bases were not necessary. The present method shows eco-friendly, economical, broad scope of substrates and practical advantages over the previous methods. Further applications of CuFe2O4 magnetic nanoparticles in the synthesis of other useful heterocycles is underway in our laboratory.
Acknowledgements
The authors gratefully acknowledge the financial support from the National Natural Science Foundation of China (no. 21302110, 21375075 and 21302109), the Taishan Scholar Foundation of Shandong Province, the Project of Shandong Province Higher Educational Science and Technology Program (J13LD14), the Natural Science Foundation of Shandong Province (ZR2013BQ017), and the Scientific Research Foundation of Qufu Normal University (BSQD 2012021). We thank Ning Zhang in this group for reproducing the results of 3k, 3u and 3C′.
References
- J. A. Asensio and P. Gomez-Romero, Fuel Cell, 2005, 5, 336 CrossRef CAS
.
-
(a) N. H. Hauel, H. Nar, H. Priepke, U. Ries, J. Stassen and W. Wienen, J. Med. Chem., 2002, 45, 1757 CrossRef CAS
;
(b) A. W. White, R. Almassy, A. H. Calvert, N. J. Curtin, R. J. Griffin, Z. Hostomsky, K. Maegley, D. R. Newell, S. Srinivasan and B. T. Golding, J. Med. Chem., 2000, 43, 4084 CrossRef CAS
.
-
(a) E. Kashiyama, I. Hutchinson, M. S. Chua, S. F. Stinson, L. R. Phillips, G. Kaur, E. A. Sausville, T. D. Bradshaw, A. D. Westwell and M. F. G. Stevens, J. Med. Chem., 1999, 42, 4172 CrossRef CAS
;
(b) W. R. Bowman, H. Heaney and P. H. G. Smith, Tetrahedron Lett., 1982, 23, 5093 CrossRef CAS
.
- N. J. Liverton, D. J. Armstrong, D. A. Claremon, D. C. Remy, J. J. Baldwin, R. J. Lynch, G. Zhang and R. J. Gould, Bioorg. Med. Chem. Lett., 1998, 8, 483 CrossRef CAS
.
- P. J. Palmer, R. B. Trigg and J. V. Warrington, J. Med. Chem., 1971, 14, 248 CrossRef CAS
.
- M. L. McKee and S. M. Kerwin, Bioorg. Med. Chem., 2008, 16, 1775 CrossRef CAS
.
- A. Benazzou, T. Boraund, P. Dubedat, J. M. Boireau and C. Stutzmann, Eur. J. Pharmcol., 1995, 284, 299 CrossRef
.
- S. K. Ivanov and V. S. Yuritsyn, Neftekhimiya, 1971, 11, 99 CAS
.
- H. Nakano, T. Inoue, N. Kawasaki, H. Miyataka, H. Matsumoto, T. Taguchi, N. Inagaki, H. Nagai and T. Satoh, Bioorg. Med. Chem., 2000, 8, 373 CrossRef CAS
.
- For selected examples, see:
(a) H. Wang, L. Wang, J. Shang, X. Li, H. Wang, J. Gui and A. W. Lei, Chem. Commun., 2012, 48, 76 RSC
;
(b) J. Bonnamour and C. Bolm, Org. Lett., 2008, 10, 2665–2667 CrossRef CAS
;
(c) B. Zou, Q. Yuan and D. Ma, Angew. Chem., Int. Ed., 2007, 46, 2598 CrossRef CAS
;
(d) E. A. Jaseer, D. J. C. Prasad, A. Dandapat and G. Sekar, Tetrahedron Lett., 2010, 51, 5009 CrossRef CAS
;
(e) G. Evindar and R. A. Batey, J. Org. Chem., 2006, 71, 1802 CrossRef CAS
;
(f) P. Saha, T. Ramana, N. Purkait, M. Ali, R. Paul and T. Punniyamurthy, J. Org. Chem., 2009, 74, 8719 CrossRef CAS
;
(g) S. Ueda and H. Nagasawa, Angew. Chem., Int. Ed., 2008, 47, 6411 CrossRef CAS
;
(h) D. Bernardi, L. A. Ba and G. Kirsch, Synlett, 2007, 18, 2121 CrossRef
;
(i) C. Benedi, F. Bravo, P. Uriz, E. Fernandez, C. Claver and S. Castillon, Tetrahedron Lett., 2003, 44, 6073 CrossRef CAS
.
- For selected examples, see:
(a) L. M. Dudd, E. Venardou, E. Garcia-Verdugo, P. Licence, A. J. Blake, C. Wilson and M. Poliakoff, Green Chem., 2003, 5, 187 RSC
;
(b) K. Takeda, S. Yano, M. Sato and E. Yoshii, J. Org. Chem., 1987, 52, 413 CrossRef
;
(c) V. K. Tandon and M. Kumar, Tetrahedron Lett., 2004, 45, 4185 CrossRef CAS
;
(d) J. Charton, S. Girault-Mizzi and C. Sergheraert, Chem. Pharm. Bull., 2005, 53, 492 CrossRef CAS
;
(e) S.-Y. Lin, Y. Isome, E. Stewart, J.-F. Liu, D. Yohannes and L. Yu, Tetrahedron Lett., 2006, 47, 2883 CrossRef CAS
;
(f) L. M. Dudd, E. Venardou, E. Garcia-Verdugo, P. Licence, A. J. Blake, C. Wilson and M. Poliakoff, Green Chem., 2003, 5, 187 RSC
;
(g) M. B. Maradolla, S. K. Allam, A. Mandha and G. V. P. Chandramouli, ARKIVOC, 2008, 15, 42 Search PubMed
;
(h) V. K. Tandon and M. Kumar, Tetrahedron Lett., 2004, 45, 4185 CrossRef CAS
;
(i) X. Wen, J. E. Bakali, R. D. Poulain and B. Deprez, Tetrahedron Lett., 2012, 53, 2440 CrossRef CAS
.
- I. M. Baltork, A. R. Khosropour and S. F. Hojati, Catal. Commun., 2007, 8, 1865 CrossRef
.
- R. S. Varma and D. J. Kumar, Heterocycl. Chem., 1998, 35, 1539 CrossRef CAS
.
- A. B. Naidu and G. Sekar, Synthesis, 2010, 579 CAS
.
- K. Nagata, T. Itoh, H. Ishikawa and A. Ohsawa, Heterocycles, 2003, 61, 93 CrossRef CAS
.
- H. Hashem, H. S. Mona and M. Fatemeh, Can. J. Chem., 2008, 86, 1044 CrossRef
.
- B. Das, H. Holla and Y. Srinivas, Tetrahedron Lett., 2007, 48, 61 CrossRef CAS
.
- Y. K. Liu, D. J. Mao, S. J. Lou, J. Q. Qian and Z. Y. Xu, J. Zhejiang Univ., Sci., B, 2009, 10, 472 CrossRef CAS
.
- J. Chang, K. Zhao and S. Pan, Tetrahedron Lett., 2002, 43, 951 CrossRef CAS
.
- R. S. Varma, R. K. Saini and O. Prakash, Tetrahedron Lett., 1997, 38, 2621 CrossRef CAS
.
- J. B. Chang, K. Zhao and S. F. Pan, Tetrahedron Lett., 2002, 43, 951 CrossRef CAS
.
- Y.-X. Chen, L.-F. Qian, W. Zhang and B. Han, Angew. Chem., Int. Ed., 2008, 47, 9330 CrossRef CAS PubMed
.
- Y. Kawashita, N. Nakamichi, H. Kawabata and M. Hayashi, Org. Lett., 2003, 5, 3713 CrossRef CAS PubMed
.
- Y. Ho Cho, C.-Y. Lee, D.-C. Ha and C.-H. Cheon, Adv. Synth. Catal., 2012, 354, 2992 CrossRef
.
- G. W. Breton, J. Org. Chem., 1997, 62, 8952 CrossRef CAS
.
- M. Kidwai, V. Bansal, A. Saxena, S. Aerry and S. Mozumdar, Tetrahedron Lett., 2006, 47, 8049 CrossRef CAS
.
-
(a) S. M. Inamdar, V. K. More and S. K. Mandal, Tetrahedron Lett., 2013, 54, 579 CrossRef CAS
;
(b) A. Corma and H. Garcia, Chem. Soc. Rev., 2008, 37, 2096 RSC
;
(c) M. B. Gawande, R. K. Pandey and R. V. Jayaram, Catal. Sci. Technol., 2012, 2, 1113 RSC
;
(d) M. B. Gawande, P. S. Branco, I. D. Nogueira, C.
A. A. Ghumman, N. Bundaleski, A. Santos, O. M. N. D. Teodoro and R. Luque, Green Chem., 2013, 15, 682 RSC
.
-
(a) M. B. Gawande, P. S. Brancoa and R. S. Varma, Chem. Soc. Rev., 2013, 42, 3371 RSC
;
(b) R. B. Nasir Baig and R. S. Varma, Chem. Commun., 2013, 49, 752 RSC
, and references therein;
(c) M. B. Gawande, A. K. Rathi, P. S. Branco, I. D. Nogueira, A. Velhinho, J. J. Shrikhande, U. U. Indulkar, R. V. Jayaram, C. A. A. Ghumman, N. Bundaleski and O. M. N. D. Teodoro, Chem.–Eur. J., 2012, 18, 12628 CrossRef CAS PubMed
;
(d) M. B. Gawande, A. K. Rathi, I. D. Nogueira, R. S. Varma and P. S. Branco, Green Chem., 2013, 15, 1895 RSC
;
(e) M. B. Gawande, V. D. B. Bonifácio, R. S. Varma, I. D. Nogueira, N. Bundaleski, C. A. A. Ghumman, O. M. N. D. Teodoro and P. S. Branco, Green Chem., 2013, 15, 1226 RSC
;
(f) C. Ó. Dálaigh, S. A. Corr, Y. Gun'ko and S. J. Connon, Angew. Chem., Int. Ed., 2007, 46, 4329 CrossRef PubMed
.
- G. Brahmachari, S. Laskar and P. Barik, RSC Adv., 2013, 3, 14245 RSC
.
-
(a) D. Kundu, T. Chatterjee and B. C. Ranu, Adv. Synth. Catal., 2013, 355, 2285 CrossRef CAS
;
(b) A. Dandia, A. K. Jain and S. Sharma, RSC Adv., 2013, 3, 2924 RSC
;
(c) S. Yang, C. Wu, H. Zhou, Y. Yang, Y. Zhao, C. Wang, W. Yang and J. Xu, Adv. Synth. Catal., 2013, 355, 53 CrossRef CAS
;
(d) R. Parella, K. N. B. Amit and A. Srinivasarao, Tetrahedron Lett., 2013, 54, 1738 CrossRef CAS
;
(e) K. Swapna, S. N. Murthy, M. T. Jyothi and Y. V. D. Nageswar, Org. Biomol. Chem., 2011, 5989 RSC
;
(f) R. Hudson, S. Ishikawa, C.-J. Li and A. Moores, Synlett, 2013, 24, 1637 CrossRef CAS
;
(g) A. S. Kumar, M. Reddy, A. M. Knorn, O. Reiser and B. Sreedhar, Eur. J. Org. Chem., 2013, 4674 CrossRef CAS
;
(h) J. Feng, L. Su and Y. Ma, Chem. Eng. J., 2013, 221, 16 CrossRef CAS
;
(i) P. Ramarao, Naveen and B. Srinivasarao Arulananda, Catal. Commun., 2012, 29, 118–121 CrossRef
;
(j) B. S. P. Anil Kumar, K. Harsha Vardhan Reddy, B. Madhav, K. Ramesh and Y. V. D. Nageswar, Tetrahedron Lett., 2012, 53, 4595 CrossRef CAS
;
(k) G. Brahmachari, S. Laskar and P. Barik, RSC Adv., 2013, 3, 14245 RSC
;
(l) S. M. Baghbanian and M. Farhang, RSC Adv., 2014, 4, 11624 RSC
;
(m) D. Kundu, N. Mukherjee and B. C. Ranu, RSC Adv., 2013, 3, 117 RSC
;
(n) R. Zhang, J. Liu, S. Wang, J. Niu, C. Xia and W. Sun, ChemCatChem, 2011, 3, 146 CrossRef CAS
.
- N. Panda, A. K. Jena, S. Mohapatra and S. R. Rout, Tetrahedron Lett., 2011, 51, 1924 CrossRef
.
- N. Barbero, M. Carril, R. SanMartin and E. Domínguez, Tetrahedron, 2007, 63, 10425 CrossRef CAS
.
- K. Kawashita, N. Nakamichi, H. Kawabata and M. Hayashi, Org. Lett., 2003, 5, 3713 CrossRef PubMed
.
- S. Ponnala and D. P. Sahu, Synth. Commun., 2006, 36, 2189 CrossRef
.
- M. M. Guru, M. A. Ail and T. Punniyamurthy, Org. Lett., 2011, 13, 1194 CrossRef CAS PubMed
.
- J. Peng, C. Zong, M. Ye, T. Chen, D. Gao, Y. Wang and C. Chen, Org. Biomol. Chem., 2011, 9, 1225 CAS
.
- Y. Leng, F. Yang, W. Zhu, D. Zou, Y. Wu and R. Cai, Tetrahedron, 2011, 67, 6191 CrossRef CAS
.
- P. Saha, T. Ramana, N. Purkait, M. A. A. Rajesh Paul and T. Punniyamurthy, J. Org. Chem., 2009, 74, 8719 CrossRef CAS PubMed
.
- J.-J. Lee, J. Kim, Y. M. Jun, B. M. Lee and B. H. Kim, Tetrahedron, 2009, 65, 8821 CrossRef CAS
.
- N. Barbero, M. Carril, R. SanMartin and E. Domínguez, Tetrahedron, 2007, 63, 10425 CrossRef CAS
.
- P.-J. Boissarie, Z. E. Hamilton, S. Lang, J. A. Murphy and C. J. Suckling, Org. Lett., 2011, 13, 6256 CrossRef CAS PubMed
.
- Y. Leng, F. Yang, W. Zhu, Y. Wu and X. Li, Org. Biomol. Chem., 2011, 9, 5288 CAS
.
- M. M. Firouz and Z. B. Hassan, Synlett, 2005, 10, 1612 Search PubMed
.
- K. Bahrami, M. M. Khodaei and A. Nejati, Green Chem., 2010, 12, 1237 RSC
.
- A. Brembilla, Synth. Commun., 1990, 20, 3379 CrossRef CAS
.
- D. L. Boger, J. Org. Chem., 1978, 43, 2296 CrossRef CAS
.
- H. Hachiya, K. Hirano, T. Satoh and M. Miura, Org. Lett., 2009, 11, 1737 CrossRef CAS PubMed
.
- B. George and E. P. Papadopoulos, J. Org. Chem., 1977, 42, 441 CrossRef CAS
.
- M. Rope, R. W. Isensee and L. Joseph, J. Am. Chem. Soc., 1952, 74, 1095 CrossRef CAS
.
- L.-H. Du and Y.-G. Wang, Synthesis, 2007, 5, 675 Search PubMed
.
Footnote |
† Electronic supplementary information (ESI) available. See DOI: 10.1039/c4ra00559g |
|
This journal is © The Royal Society of Chemistry 2014 |