DOI:
10.1039/C4RA00093E
(Paper)
RSC Adv., 2014,
4, 10402-10411
FeCl3·6H2O catalyzed aqueous media domino synthesis of 5-monoalkylbarbiturates: water as both reactant and solvent†
Received
6th January 2014
, Accepted 30th January 2014
First published on 31st January 2014
Abstract
A novel, simple and straightforward route to 5-monoalkylbarbiturates by FeCl3·6H2O catalyzed domino reactions of 6-aminouracils, water and α,β-unsaturated ketones, where water plays a key dual role as both reactant and solvent, is described. Significantly, all the reactions efficiently furnished exclusively 5-monoalkylbarbiturates and not pyrido[2,3-d]pyrimidines as generally produced from the reactions of 6-aminouracils and α,β-unsaturated carbonyls.
Introduction
In recent years, the development of greener synthetic methods has been highly prioritized in view of the adverse implications of various chemical processes. Especially, taking into account the undesirable impacts of organic solvents, efforts to accomplish efficient organic synthesis in aqueous medium present a focal point of research in current synthetic chemistry. Water is not only the most abundant and non-toxic solvent, it also enables novel reactivity and accelerates reaction by the hydrophobic and ‘on water’ effects.1 Meanwhile, multi-component reactions (MCRs)2 and auto-tandem catalysis3 have become powerful strategies towards convergent synthesis for the facts that the former allows flexible, convergent, pot, atom and step economic synthesis while the latter provides maximum catalyst utilization efficiency by catalyzing two or more mechanistically different organic transformations. However, the development of multi-component reactions in aqueous environments is a recent endeavour that has received relatively little attention and consequently requires greater emphasis.1a,g,4
In these developments, iron catalysts have emerged as a center of renewed interest both in homogeneous and heterogeneous catalysis. Well known for their wide range of tolerance, iron catalysts are diversely applied in addition, substitution, cycloaddition and polymerization reactions to name a few.5 In particular, FeCl3·6H2O has received tremendous applications in organic syntheses whose applicability has been further advantaged by its cost effectiveness, ease of handling and environmental benignity.5,6 Thus, iron catalyzed organic transformations are highly applicable approaches in organic syntheses.
5-Alkylbarbiturates are an intriguing and re-emerging privileged class of compounds in medicinal chemistry which have broad range of activities such as anticonvulsant,7 sedative,8 immunomodulating and antitumor properties;9 whilst a number of them also have found wide applications in the manufacture of dyes,10 non linear optical study11 and in supramolecular chemistry.12 Further synthetic interest on 5-alkylbarbiturates has been elevated with the development of highly potent antibacterial PNU-286607(-)-1 (ref. 13) and inhibitors of matrix metalloproteinase (MMP)14 and mutant SOD1-dependent protein aggregation.15 Classically, 5-alkylbarbiturates can be synthesized by condensation of alkylated malonic esters and urea in the presence of sodium alkoxide.16 However, the yields of this reaction are often modest due to the presence of side reactions such as hydrolysis of the malonate, decarbethoxylation, transesterification, and urea degradation. Moreover, the need for dry solvents and high temperature in addition to the requirement of inert atmosphere and metallic sodium limits the use of this classical method in the perspective of combinatorial purposes and diversity-oriented synthetic programs. Alternatively, 5-alkylation of unsubstituted barbituric acid could be a strategy towards 5-alkylbarbiturates.17 But, in particular, direct construction of 5-monoalkylbarbiturates by alkylation of barbituric acid derivatives still remains a difficult and an inspiring task.
A common challenge en route to 5-monoalkylbarbiturates is the specific 5-monoalkylation of barbituric acid derivatives. For decades, there was no simple procedure for this strategy until, lately, Jursic and co-workers developed an effective reductive alkylation procedure in the presence of platinum and palladium catalysts.18 More recently, Löfberg and group have described Ir(III) catalyzed reaction of barbituric acid and alcohols as an alternative route to 5-monoalkylbarbiturates.19 Another method for 5-monoalkylbarbiturates via ring opening of spiro[2.5]barbiturates was also described by Singh and Paul.20 Although these protocols are useful, the use of expensive catalysts and complex reaction conditions rather limits their applicability. The only multi-component strategy showing a prospect towards 5-monoalkylbarbiturates was developed recently by Volonterio and Zanda.21 On the other hand, the highly viable route to 5-monoalkylbarbiturates by Michael addition of barbituric acid to α,β-unsaturated carbonyls has been highly underrepresented since the first report given by Zalukaev and Trostyanetskaya22 and the compounds were characterized only on the basis of IR spectral data. And, to the best of our knowledge on literature survey, there is only another report describing Michael addition of barbituric acid to α,β-unsaturated carbonyls.23
Therefore, in view of the need to design effective synthetic route for 5-monoalkylbarbiturates, and in conjunction with our continued pursuit on environment friendly synthetic developments,24 we report herein the application of FeCl3·6H2O catalyzed domino reactions of 6-aminouracils, water and α,β-unsaturated ketones as a straightforward route to 5-monoalkylbarbiturates, where water significantly serves as both reactant and solvent (Scheme 1). This tandem reaction involves an initial FeCl3·6H2O and water mediated amine hydrolysis of the 6-aminouracil to barbituric acid followed by Michael type addition to α,β-unsaturated ketones. To the best of our knowledge, 6-aminouracils have not been explored for direct synthesis of 5-monoalkylbarbiturates. And at this point it can be noted that the general reactivity of 6-aminouracils with α,β-unsaturated carbonyls gives pyrido[2,3-d]pyrimidines,25 whereas this new found reaction produced solely 5-monoalkylbarbiturates as the final products. Thus, a new reactivity role of 6-aminouracils as valuable substrates towards 5-monoalkylbarbiturates is also discovered.
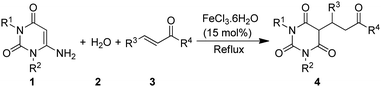 |
| Scheme 1 Domino synthesis of 5-monoalkylbarbiturates. | |
Results and discussion
Initially we refluxed 6-aminouracil (1a, 1 mmol), water (2, 10 mL) and benzylideneacetone (3a, 1 mmol) in the presence of FeCl3·6H2O (10 mol%) for sixty minutes (Table 1, entry 1). To our delight, the reaction gave 5-(3-oxo-1-phenylbutyl)pyrimidine-2,4,6(1H,3H,5H)-trione (4aa) in 80% yield. Much to our satisfaction, the reaction was then set for optimization as shown in Table 1. Fortunately, optimization of the FeCl3·6H2O catalyst was arrived at 15 mol% which afforded the highest yield of 4aa in 92% yield (Table 1, entry 2). Further, to get the best effect of the reaction, some potential Lewis acid catalysts and a number of solvents were screened (Table 1, entries 5–12). Interestingly, the reaction was found to work only with water and FeCl3·6H2O. Even solvents such as EtOH and DMSO or reactions performed under solventless condition failed to furnish the desired product (Table 1, entries 8–13). In an attempt to succeed the reaction at room temperature (≈24 °C), the process was not satisfactory and gave 4aa only in 10% yield, even after stirring for 24 hours (Table 1, entry 14) (Scheme 2). In another process, simultaneous mixing of 6-aminouracil (1a), benzaldehyde (6) and acetone (7) in the presence of FeCl3·6H2O and water did not yield the desired 5-monoalkylbarbiturate, and only barbituric acid (5) and benzylidenebarbituric acid (8) were isolated (Scheme 2). We observed that, although barbituric acid was formed in the process, the rate of formation of 8 greatly exceeded the rate of generation of benzylideneacetone (3a). Nevertheless, these results indicated that FeCl3·6H2O and water were specifically essential to obtain 5-monoalkylbarbiturates through this protocol.
Table 1 Optimization of the reaction under different conditionsa
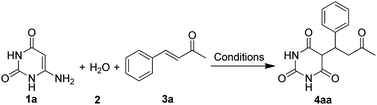
|
Entry |
Catalyst (mol%) |
Solventb |
Temp (°C) |
Time (min) |
Yieldc (%) |
Reaction scale: 1a (1 mmol), 2 (10 mL) and 3a (1 mmol). 10 mL. Isolated yield. RT ≈ 24 °C. |
1 |
FeCl3·6H2O (10) |
H2O |
Reflux |
60 |
80 |
2 |
FeCl3·6H2O (15) |
H2O |
Reflux |
45 |
92 |
3 |
FeCl3·6H2O (20) |
H2O |
Reflux |
45 |
92 |
4 |
FeCl3·6H2O (25) |
H2O |
Reflux |
45 |
92 |
5 |
CuCl2·2H2O (15) |
H2O |
Reflux |
60 |
0 |
6 |
NiCl2·6H2O (15) |
H2O |
Reflux |
60 |
0 |
7 |
CoCl2·6H2O (15) |
H2O |
Reflux |
60 |
0 |
8 |
FeCl3·6H2O (15) |
EtOH |
Reflux |
60 |
0 |
9 |
FeCl3·6H2O (15) |
CH3CN |
Reflux |
60 |
0 |
10 |
FeCl3·6H2O (15) |
CHCl3 |
Reflux |
60 |
0 |
11 |
FeCl3·6H2O (15) |
Toluene |
Reflux |
60 |
0 |
12 |
FeCl3·6H2O (15) |
DMSO |
Reflux |
60 |
0 |
13 |
FeCl3·6H2O (15) |
— |
Heat |
50 |
0 |
14 |
FeCl3·6H2O (15) |
H2O |
RTd |
1440 |
10 |
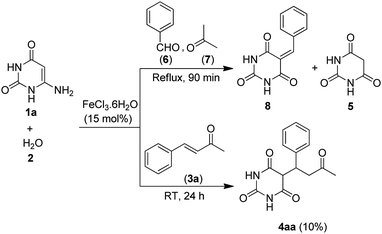 |
| Scheme 2 Reaction study towards 5-monoalkylbarbiturates. | |
Next, a comparative study on the substrate prospect of 6-aminouracil (1a) versus barbituric acid (5) towards 5-monoalkylbarbiturates was investigated by executing some parallel reactions with selected arylideneacetones (3) under the same reaction conditions (Table 2). Interestingly, both the reactions showed almost equal competency for 5-monoalkylbarbiturates yielding similar yields without significant differences in reaction times. Thus, this observation described that 6-aminouracil could be an equally competent and alternative substrate towards 5-monalkylbarbiturates.
Table 2 Comparative substrate prospect of 6-aminouracil (1a) versus barbituric acid (5) towards 5-monoalkylbarbiturates (4)a
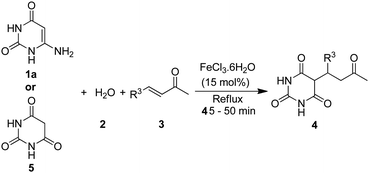
|
Entry |
R3 |
Product |
Time (min) |
Yieldb (%) |
1a |
5 |
1a |
5 |
All reactions were carried out using 1 mmol each of 1a/5, 3 and 10 mL of 2. Isolated yield. |
1 |
C6H5 |
4aa |
45 |
40 |
92 |
92 |
2 |
3-OCH3C6H4 |
4ad |
50 |
45 |
85 |
85 |
3 |
4-ClC6H4 |
4ae |
45 |
41 |
92 |
93 |
4 |
4-NO2C6H4 |
4af |
45 |
40 |
89 |
89 |
5 |
2-Thiophenyl |
4am |
50 |
45 |
81 |
82 |
Subsequently, under the optimized conditions, we then explored the scope of the reaction. As shown in Table 3, a wide array of 5-monoalkylbarbiturates was prepared from the reaction of 6-aminouracil (1a), water (2) and various α,β-unsaturated ketones (3). It was found that, the presence of electron withdrawing or donating groups in the ortho, meta- or para- positions of the benzene ring of various arylideneacetones (3a–f) or chalcones (3g–k) had no significant impact on the reaction and they were conveniently transformed to their corresponding 5-monoalkylbarbiturates (Table 3, entries 1–11; 76–92%). To our delight, heterylideneacetones (3l and 3m) and heterylideneacetophenones (3n and 3o) also participated well in the reaction and provided their corresponding products in good to high yields (Table 3, entries 12–15; 76–81%). Other substituted alken-2-ones (3p–r) also responded moderately to the reaction and their resultant 5-monoalkylbarbiturates were successfully obtained although the reactions were slightly sluggish (Table 3, entries 16–18; 71–74%).
Table 3 FeCl3·6H2O catalyzed aqueous media domino synthesis of 5-monoalkylbarbituratesa
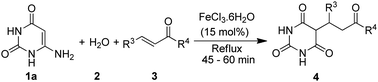
|
Entry |
3 |
Time (min) |
Product |
Yieldb (%) |
R3 |
R4 |
Reaction scale: 1a (1 mmol), 2 (10 mL) and 3 (1 mmol). Isolated yield. Purified by column chromatography. |
1 |
C6H5 |
CH3 |
3a |
45 |
4aa |
92 |
2 |
2-ClC6H4 |
CH3 |
3b |
45 |
4ab |
88 |
3 |
3-ClC6H4 |
CH3 |
3c |
50 |
4ac |
85 |
4 |
3-OCH3C6H4 |
CH3 |
3d |
50 |
4ad |
85 |
5 |
4-ClC6H4 |
CH3 |
3e |
45 |
4ae |
92 |
6 |
4-NO2C6H4 |
CH3 |
3f |
45 |
4af |
89 |
7 |
C6H5 |
C6H5 |
3g |
45 |
4ag |
87 |
8 |
2-ClC6H4 |
C6H5 |
3h |
45 |
4ah |
90 |
9 |
3-OCH3C6H4 |
C6H5 |
3i |
50 |
4ai |
83 |
10 |
4-ClC6H4 |
C6H5 |
3j |
45 |
4aj |
90 |
11 |
3,5-(OCH3)2C6H3 |
C6H5 |
3k |
50 |
4ak |
76 |
12 |
2-Furyl |
CH3 |
3l |
45 |
4al |
78 |
13 |
2-Thiophenyl |
CH3 |
3m |
50 |
4am |
81 |
14 |
2-Furyl |
C6H5 |
3n |
60 |
4anc |
76 |
15 |
2-Thiophenyl |
C6H5 |
3o |
50 |
4ao |
80 |
16 |
Ethyl |
CH3 |
3p |
50 |
4apc |
71 |
17 |
Butyl |
CH3 |
3q |
50 |
4aqc |
73 |
18 |
Butyl |
C6H5 |
3r |
50 |
4arc |
74 |
The scope of the reaction was also extended towards 1-methyl-6-aminouracil (1b) and 1,3-dimethyl-6-aminouracil (1c) under the same conditions and the results are shown in Table 4. Gratifyingly, all reactions of 1b or 1c with various α,β-unsaturated ketones (3) and water (2) proceeded successfully without much complexity and furnished their 5-monoalkylbarbiturates (68–91%). However, in the case of 1,3-dimethyl-6-aminouracil (1c), conversions were found to be relatively slower than when 6-aminouracil (1a) or 1-methyl-6-aminouracil (1b) was employed. This may be due to the presence of electron releasing methyl group/s which impeded hydrolysis of the amine to form barbituric acid. And, unlike those reactions with 6-aminouracil (1a), most of the reactions involving 1-methyl-6-aminouracil (1b) and 1,3-dimethyl-6-aminouracil (1c) were slightly sluggish. Thus, a reactivity aptitude: 6-aminouracil (1a) > 1-methyl-6-aminouracil (1b) > 1,3-dimethyl-6-aminouracil (1c) was observed in this study. Furthermore, the 5-monoalkylbarbiturates (4bi–4bl) obtained from reactions involving 1-methyl-6-aminouracil (1b) showed their existence as diastereoisomers as revealed by 1H and 13C NMR spectra. Meanwhile, to further supplement the structural characterization, a single crystal X-ray diffraction study was probed upon 4bb whose X-ray structure is depicted in Fig. 1.
Table 4 Substituted 6-aminouracils towards 5-monoalkylbarbituratesa
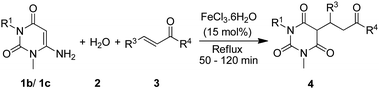
|
Entry |
1b/1c R1 |
3 |
Time (min) |
Product |
Yieldb (%) |
R3 |
R4 |
All reactions were carried out using 1 (1 mmol), 2 (10 mL) and 3 (1 mmol). Isolated yield. Purified by column chromatography. Combined diastereomeric yield. |
1 |
CH3 |
C6H5 |
CH3 |
3a |
90 |
4bac |
83 |
2 |
CH3 |
2-ClC6H4 |
CH3 |
3b |
120 |
4bb |
87 |
3 |
CH3 |
3-ClC6H4 |
CH3 |
3c |
120 |
4bcc |
78 |
4 |
CH3 |
3-OCH3C6H4 |
CH3 |
3d |
120 |
4bdc |
85 |
5 |
CH3 |
3-NO2C6H4 |
CH3 |
3s |
120 |
4bec |
84 |
6 |
CH3 |
4-BrC6H4 |
CH3 |
3t |
90 |
4bfc |
87 |
7 |
CH3 |
4-OHC6H4 |
CH3 |
3u |
120 |
4bg |
68 |
8 |
CH3 |
4-NO2C6H4 |
CH3 |
3f |
105 |
4bhc |
84 |
9 |
H |
2-ClC6H4 |
CH3 |
3b |
60 |
4bic,d |
88 |
10 |
H |
2-FC6H4 |
C6H5 |
3v |
60 |
4bjc,d |
85 |
11 |
H |
3-ClC6H4 |
CH3 |
3c |
60 |
4bkc,d |
88 |
12 |
H |
4-BrC6H4 |
CH3 |
3w |
50 |
4blc,d |
91 |
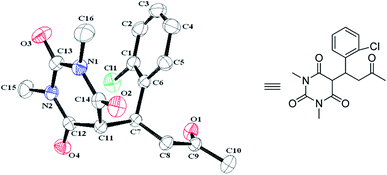 |
| Fig. 1 X-ray crystal structure of 4bb.26 | |
To probe the reaction mechanism, 6-aminouracil (1a) was refluxed alone in water in the presence of FeCl3·6H2O. The reaction completed swiftly within minutes and yielded barbituric acid (Scheme 3). Furthermore, when barbituric acid and benzylideneacetone were refluxed without any catalyst for 45 minutes, the product 4aa was resulted but only in 20% yield, which was indicative that FeCl3·6H2O also has catalytic role in the addition step. Thus, based upon literature reports6b,c,e,20,27 and our results, a possible mechanism is proposed in Scheme 4. The coordination of 6-aminouracil with FeCl3 facilitated amine hydrolysis by water and generated barbituric acid (5) via 9 and 10. The in situ formed barbituric acid further underwent complexation with FeCl3 to give intermediate 11 while, apparently, α,β-unsaturated ketone (3) also gets activated with FeCl3 to 12. Subsequent addition of the activated complex 11 to 12 followed by protonation finally led to the formation of 5-monoalkylbarbiturate 4.
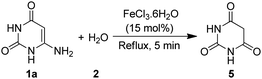 |
| Scheme 3 Barbituric acid from 6-aminouracil. | |
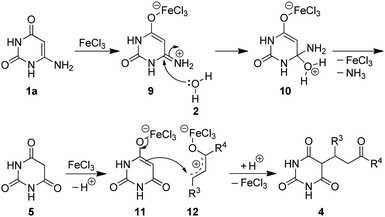 |
| Scheme 4 Proposed reaction mechanism. | |
Conclusions
In summary we have developed, for the first time, a simple, general and environment friendly protocol for synthesis of 5-monoalkybarbiturates directly through FeCl3·6H2O catalyzed domino reaction of 6-aminouracils, water and α,β-unsaturated ketones. Significantly, this study has also demonstrated the key role of water as both reactant and solvent in achieving the synthesis, which further exemplifies for wider applications of aqueous media organic synthesis. Moreover, while barbituric acid has served as common substrate for 5-monalkylbarbiturates thus far, our study has now demonstrated that 6-aminouracils can be also alternative and equally competent reactants towards obtaining the same compounds. Therefore, provided by the versatility of the catalyst, wide substrate scope and mild reaction conditions, the protocol is highly facile which remarkably expands the procedural scopes for the synthesis of a huge library of important 5-monoalkylbarbiturates, suitable as well for combinatorial synthetic study.
Experimental section
All reagents were purchased from commercial suppliers and were used without further purification. The α,β-unsaturated ketones were prepared according to literature procedure.28 IR spectra were recorded on a SHIMADZU infrared spectrometer as KBr pellets with absorption in cm−1. 1H and 13C NMR spectra were recorded in CDCl3 or DMSO-d6 or MeOH-d4 on 300 MHz Bruker NMR spectrometer at ≈25 °C and resonances (δ) are given in ppm relative to tetramethylsilane. Data are reported as follows: chemical shift (δ), multiplicity (s = singlet, d = doublet, t = triplet, m = multiplet, ‡ = double signal), coupling constants (Hz) and integration. LCMS were obtained on Waters ZQ 4000 and equipped with ESI source. Melting points were determined using Veego VMP-D and not corrected. The X-ray crystal structure determination was done on a Bruker, SMART APEX II CCD system. Elemental analysis was done on Perkin Elmer Series II Analyzer 2400. Column chromatography was performed on silica gel (200–300 mesh) using ethyl acetate
:
hexane (6
:
4) as the eluent. Thin Layer Chromatography (TLC) was performed using Merck pre-coated silica gel or silica gel G and the components were visualized under a UV or an iodine chamber.
General procedure for the synthesis of 5-monoalkylbarbiturates (4aa–ar and 4ba–bl) from 6-aminouracils, water and α,β-unsaturated ketones
A mixture of 6-aminouracil (1a/1b/1c, 1 mmol), α,β-unsaturated ketone (3, 1 mmol) in water (2, 10 mL) was refluxed in the presence of FeCl3·6H2O (15 mol%) as catalyst for appropriate time (Tables 3 and 4). On completion of the reaction, as indicated by TLC, the crude reaction mass was cooled and was extracted with ethyl acetate (10 mL × 4). After drying with anhydrous Na2SO4 and evaporation under reduced pressure, the crude product was purified suitably either by recrystallization from DCM
:
ethanol (6
:
4) solvent mixture or ethanol or column chromatography on silica gel using ethyl acetate
:
hexane (6
:
4) as the eluent to afford 5-monoalkylbarbiturates.
General procedure for the synthesis of 5-monoalkylbarbiturates from barbituric acid and α,β-unsaturated ketones (Table 2)
A mixture of barbituric acid (5, 1 mmol) and α,β-unsaturated ketone (3, 1 mmol) was refluxed in the presence of FeCl3·6H2O (15 mol%) in water (10 mL) for appropriate time. On completion of the reactions, as indicated by TLC, the reaction mass was cooled and extracted with ethyl acetate (10 mL × 4) and dried over anhydrous Na2SO4. After concentrated under reduced pressure, the obtained crude solids were further purified by recrystallization from DCM
:
ethanol (6
:
4) solvent mixture to afford the 5-monoalkylbarbiturates.
Procedure for the synthesis of barbituric acid from 6-aminouracil
6-Aminouracil (1a, 1 mmol) was refluxed in water (2, 10 mL) in the presence of FeCl3·6H2O (15 mol%) for 5 minutes. On completion of the reaction, as indicated by TLC, the reaction mass was cooled and extracted with ethyl acetate (10 mL × 4). The organic extract was dried over anhydrous Na2SO4 and concentrated under reduced pressure. The obtained solid product was purified by recrystallization from ethanol
:
water (1
:
9). The physical and chemical properties are identical to that reported in the literature.29
5-(3-Oxo-1-phenylbutyl)pyrimidine-2,4,6(1H,3H,5H)-trione (4aa)30
Yield 0.252 g (92%). White solid, mp 152–154 °C (from EtOH/DCM). 1H NMR (300 MHz, DMSO-d6): δH (ppm) 2.08 (s, 3H), 3.05–3.14 (m, 1H), 3.31–3.40 (m, 1H), 3.65 (d, 1H, J = 3.9 Hz), 3.88–3.95 (m, 1H), 7.04–7.13 (m, 2H), 7.18–7.27 (m, 3H), 11.03 (s, 1H), 11.09 (s, 1H); 13C NMR (75 MHz, DMSO-d6): δC (ppm) 30.7, 41.8, 45.6, 52.2, 127.8, 128.1, 128.8, 139.7, 150.9, 170.2, 170.7, 207.4; IR (KBr) (νmax/cm−1) 3392, 2923, 1729, 1704, 1681, 1664; MS (ESI): m/z calcd for C14H14N2O4: 274.10; found 275.10 [M + H]+, 297.00 [M + Na]+; anal. calcd for C14H14N2O4: C, 61.31; H, 5.14; N, 10.21. Found: C, 61.44; H, 5.26; N, 10.08%.
5-(1-(2-Chlorophenyl)-3-oxobutyl)pyrimidine-2,4,6(1H,3H,5H)-trione (4ab)
Yield, 0.271 g (88%). White solid, mp 102–104 °C (from EtOH/DCM). 1H NMR (300 MHz, CDCl3): δH (ppm) 2.10 (s, 3H), 2.90–2.94 (m, 1H), 3.24–3.33 (m, 1H), 3.84 (d, 1H, J = 3.3 Hz), 4.68 (m, 1H), 7.08–7.39 (m, 4H), 9.46 (s, 1H), 9.58 (s, 1H); 13C NMR (75 MHz, CDCl3): δC (ppm) 30.0, 36.6, 44.5, 50.8, 127.4, 128.7, 128.9, 129.9, 133.6, 137.9, 150.5, 168.8, 169.0, 208.8; IR (KBr) (νmax/cm−1) 3410, 3011, 2924, 1719, 1701, 1687, 1659, 1551; MS (ESI): m/z calcd for C14H13ClN2O4: 308.06; found 308.92 [M + H]+, 331.04 [M + Na]+; anal. calcd for C14H13ClN2O4: C, 54.47; H, 4.24; N, 9.07. Found: C, 54.69; H, 4.09; N, 9.19%.
5-(1-(3-Chlorophenyl)-3-oxobutyl)pyrimidine-2,4,6(1H,3H,5H)-trione (4ac)
Yield, 0.262 g (85%). White solid, mp 103–105 °C (from EtOH/DCM). 1H NMR (300 MHz, CDCl3): δH (ppm) 2.18 (s, 3H), 2.90–2.98 (m, 1H), 3.51–3.61 (m, 1H), 3.89 (d, 1H, J = 3.3 Hz), 4.09–4.16 (m, 1H), 7.09–7.27 (m, 4H), 9.10 (s, 1H), 9.16 (s, 1H); 13C NMR (75 MHz, CDCl3): δC (ppm) 30.3, 40.8, 45.1, 51.3, 126.3, 128.1, 130.2, 134.6, 141.2, 149.8, 168.8, 208.2; IR (KBr) (νmax/cm−1) 3232, 3109, 2924, 1728, 1701, 1693, 1647, 1554; MS (ESI): m/z calcd for C14H13ClN2O4: 308.06; found 308.92 [M + H]+, 331.01 [M + Na]+; anal. calcd for C14H13ClN2O4: C, 54.47; H, 4.24; N, 9.07. Found: C, 54.71; H, 4.06; N, 9.25%.
5-(1-(3-Methoxyphenyl)-3-oxobutyl)pyrimidine-2,4,6(1H,3H,5H)-trione (4ad)
Yield, 0.258 g (85%). White solid, mp 141–143 °C (from EtOH/DCM). 1H NMR (300 MHz, CDCl3): δH (ppm) 2.15 (s, 3H), 2.90–2.97 (m, 1H), 3.45–3.55 (m, 1H), 3.68 (s, 3H), 3.81 (d, 1H, J = 3.9 Hz), 4.03–4.12 (m, 1H), 6.69–6.78 (m, 3H), 7.10–7.15 (m, 1H), 9.39 (s, 1H), 9.47 (s, 1H); 13C NMR (75 MHz, CDCl3): δC (ppm) 30.2, 41.8, 45.2, 51.4, 55.1, 113.1, 113.8, 120.1, 129.9, 140.1, 150.2, 159.5, 169.4, 169.6, 208.3; IR (KBr) (νmax/cm−1) 3437, 3082, 2924, 1723, 1708, 1684, 1668, 1546; MS (ESI): m/z calcd for C15H16N2O5: 304.11; found 304.79 [M + H]+, 323.76 [M + H2O]+; anal. calcd for C15H16N2O5: C, 59.21; H, 5.30; N, 9.21. Found: C, 59.35; H, 5.45; N, 9.05%.
5-(1-(4-Chlorophenyl)-3-oxobutyl)pyrimidine-2,4,6(1H,3H,5H)-trione (4ae)
Yield, 0.284 g (92%). White solid, mp 247–249 °C (from EtOH/DCM). 1H NMR (300 MHz, CDCl3): δH (ppm) 2.15 (s, 3H), 2.91–2.97 (m, 1H), 3.48–3.57 (m, 1H), 3.86 (d, 1H, J = 3.0 Hz), 4.10–4.12 (m, 1H), 7.09 (d, 2H, J = 8.4 Hz), 7.18 (d, 2H, J = 8.4 Hz), 9.67 (s, 1H), 9.71 (s, 1H); 13C NMR (75 MHz, CDCl3): δC (ppm) 30.3, 40.7, 45.2, 51.3, 129.0, 129.4, 133.6, 137.4, 150.4, 169.3, 169.5, 208.4; IR (KBr) (νmax/cm−1) 3347, 3011, 2924, 1721, 1703, 1681, 1661, 1557; MS (ESI): m/z calcd for C14H13ClN2O4: 308.06; found 309.10 [M + H]+, 331.01 [M + Na]+; anal. calcd for C14H13ClN2O4: C, 54.47; H, 4.24; N, 9.07. Found: C, 54.70; H, 4.07; N, 9.21%.
5-(1-(4-Nitrophenyl)-3-oxobutyl)pyrimidine-2,4,6(1H,3H,5H)-trione (4af)
Yield, 0.284 g (89%). White solid, mp 214–216 °C (from EtOH/DCM). 1H NMR (300 MHz, DMSO-d6): δH (ppm) 2.02 (s, 3H), 3.03–3.12 (m, 1H), 3.73–3.77 (m, 1H), 3.84 (d, 1H, J = 3.6 Hz), 4.08–4.11 (m, 1H), 7.37–7.48 (m, 2H), 8.09–8.20 (m, 2H), 11.24 (s, 1H), 11.30 (s, 1H); 13C NMR (75 MHz, DMSO-d6): δC (ppm) 24.4, 42.4, 49.4, 70.6, 117.1, 123.5, 124.3, 139.3, 143.5, 164.4, 166.0, 200.4; IR (KBr) (νmax/cm−1) 3437, 3078, 2924, 1710, 1703, 1682, 1658, 1558; MS (ESI): m/z calcd for C14H13N3O6: 319.08; found 319.92 [M + H]+, 342.01 [M + Na]+; anal. calcd for C14H13N3O6: C, 52.67; H, 4.10; N, 13.16. Found: C, 52.83; H, 3.95; N, 13.29%.
5-(3-Oxo-1,3-diphenylpropyl)pyrimidine-2,4,6(1H,3H,5H)-trione (4ag)23
Yield, 0.292 g (87%). White solid, mp 176–178 °C (from EtOH/DCM). 1H NMR (300 MHz, DMSO-d6): δH (ppm) 3.58–3.66 (m, 1H), 3.79 (d, 1H, J = 3.6 Hz), 4.00–4.09 (m, 1H), 4.13–4.19 (m, 1H), 7.23 (d, 2H, J = 9.0 Hz), 7.28–7.31 (m, 3H), 7.53 (t, 2H, J = 7.3 Hz), 7.65 (t, 1H, J = 6.9 Hz), 7.98 (d, 2H, J = 7.5 Hz), 11.06 (s, 1H), 11.11 (s, 1H); 13C NMR (75 MHz, DMSO-d6): δC (ppm) 45.8, 46.8, 56.9, 132.6, 133.0, 133.1, 133.6, 134.0, 138.6, 141.8, 144.7, 155.7, 175.1, 175.5, 203.5; IR (KBr) (νmax/cm−1) 3424, 3017, 2923, 1721, 1705, 1682, 1662, 1561; MS (ESI): m/z calcd for C19H16N2O4: 336.11; found 337.01 [M + H]+, 359.01 [M + Na]+; anal. calcd for C19H16N2O4: C, 67.85; H, 4.79; N, 8.33. Found: C, 67.71; H, 4.67; N, 8.49%.
5-(1-(2-Chlorophenyl)-3-oxo-3-phenylpropyl)pyrimidine-2,4,6(1H,3H,5H)-trione (4ah)23
Yield, 0.333 g (90%). White solid, mp 196–198 °C (from EtOH/DCM). 1H NMR (300 MHz, DMSO-d6): δH (ppm) 3.55–3.64 (m, 1H), 3.73–3.82 (m, 1H), 3.88 (d, 1H, J = 3.6 Hz), 4.65–4.71 (m, 1H), 7.21–7.29 (m, 2H), 7.40 (d, 2H, J = 7.2 Hz), 7.48–7.53 (m, 2H), 7.63 (t, 1H, J = 7.2 Hz), 7.93 (d, 2H, J = 7.2 Hz), 11.10 (s, 1H), 11.13 (s, 1H); 13C NMR (75 MHz, DMSO-d6): δC (ppm) 30.3, 41.2, 45.1, 120.2, 121.3, 122.0, 122.8, 123.5, 127.1, 127.6, 130.4, 132.7, 144.8, 145.9, 161.9, 192.9; IR (KBr) (νmax/cm−1) 3411, 3071, 2923, 1720, 1709, 1688, 1664, 1552; MS (ESI): m/z calcd for C19H15ClN2O4: 370.07; found 371.00 [M + H]+, 393.10 [M + Na]+; anal. calcd for C19H15ClN2O4: C, 61.55; H, 4.08; N, 7.56. Found: C, 61.64; H, 3.93; N, 7.67%.
5-(1-(3-Methoxyphenyl)-3-oxo-3-phenylpropyl)pyrimidine-2,4,6(1H,3H,5H)-trione (4ai)
Yield, 0.304 g (83%). White solid, mp 167–169 °C (from EtOH/DCM). 1H NMR (300 MHz, CDCl3): δH (ppm) 3.44–3.52 (m, 1H), 3.71 (s, 3H), 3.97 (d, 1H, J = 3.6 Hz), 4.09–4.18 (m, 1H), 4.34–4.37 (m, 1H), 6.75–6.84 (m, 3H), 7.14–7.20 (m, 1H), 7.37–7.57 (m, 3H), 7.94–7.99 (m, 2H), 8.88 (s, 1H), 8.94 (s, 1H); 13C NMR (75 MHz, CDCl3): δC (ppm) 40.5, 42.2, 51.6, 55.2, 113.1, 114.0, 120.2, 128.1, 128.6, 130.0, 133.4, 136.5, 140.5, 149.5, 159.6, 168.9, 169.0, 198.7; IR (KBr) (νmax/cm−1) 3391, 3019, 2924, 1719, 1702, 1682, 1660, 1559; MS (ESI): m/z calcd for C20H18N2O5: 366.12; found 367.10 [M + H]+, 389.00 [M + Na]+; anal. calcd for C20H18N2O5: C, 65.57; H, 4.95; N, 7.65. Found: C, 65.69; H, 5.12; N, 7.53%.
5-(1-(4-Chlorophenyl)-3-oxo-3-phenylpropyl)pyrimidine-2,4,6(1H,3H,5H)-trione (4aj)
Yield, 0.333 g (90%). White solid, mp 175–177 °C (from EtOH/DCM). 1H NMR (300 MHz, CDCl3): δH (ppm) 3.47–3.55 (m, 1H), 4.01 (d, 1H, J = 3.6 Hz), 4.10–4.19 (m, 1H), 4.39–4.45 (m, 1H), 7.23–7.30 (m, 4H), 7.45–7.50 (m, 2H), 7.57–7.62 (m, 1H), 7.97 (d, 2H, J = 8.1 Hz), 8.21 (s, 1H), 8.25 (s, 1H); 13C NMR (75 MHz, CDCl3): δC (ppm) 40.5, 41.2, 51.6, 128.1, 128.7, 129.2, 129.4, 133.6, 134.0, 137.7, 148.4, 152.4, 153.7, 168.1, 203.6; IR (KBr) (νmax/cm−1) 3380, 3067, 2924, 1719, 1705, 1687, 1661, 1552; MS (ESI): m/z calcd for C19H15ClN2O4: 370.07; found 370.93 [M + H]+, 393.00 [M + Na]+; anal. calcd for C19H15ClN2O4: C, 61.55; H, 4.08; N, 7.56. Found: C, 61.69; H, 3.95; N, 7.68%.
5-(1-(3,5-Dimethoxyphenyl)-3-oxo-3-phenylpropyl)pyrimidine-2,4,6(1H,3H,5H)-trione (4ak)
Yield, 0.301 g (76%). White solid, mp 206–208 °C (from EtOH). 1H NMR (300 MHz, methanol-d4): δH (ppm) 3.56–3.69 (m, 1H), 3.74 (s, 6H), 3.82 (m, 1H), 4.05–4.12 (m, 1H), 4.23–4.26 (m, 1H), 6.38 (s, 1H), 6.71 (s, 2H), 7.51–7.54 (m, 2H), 7.60–7.63 (m, 1H), 8.03–8.05 (m, 2H); 13C NMR (75 MHz, methanol-d4): δC (ppm) 34.2, 36.9, 48.0, 92.9, 99.3, 121.2, 122.1, 126.7, 130.8, 134.5, 154.4, 161.5, 206.5; IR (KBr) (νmax/cm−1) 3384, 3066, 2924, 1714, 1701, 1686, 1656, 1542; MS (ESI): m/z calcd for C21H20N2O6: 396.13; found 397.21 [M + H]+, 419.14 [M + Na]+; anal. calcd for C21H20N2O6: C, 63.63; H, 5.09; N, 7.07. Found: C, 63.77; H, 5.26; N, 6.92%.
5-(1-(Furan-2-yl)-3-oxobutyl)pyrimidine-2,4,6(1H,3H,5H)-trione (4al)31
Yield, 0.206 g (78%). Yellow solid, mp 186–188 °C (from EtOH/DCM). 1H NMR (300 MHz, DMSO-d6): δH (ppm) 2.12 (s, 3H), 3.13–3.25 (m, 2H), 3.71 (m, 1H), 4.01–4.06 (m, 1H), 6.03 (m, 1H), 6.30 (m, 1H), 7.46 (m, 1H), 11.09 (s, 1H), 11.20 (s, 1H); 13C NMR (75 MHz, DMSO-d6): δC (ppm) 24.1, 28.4, 37.9, 44.0, 100.3, 104.5, 136.1, 144.7, 147.6, 163.3, 163.8, 200.6; IR (KBr) (νmax/cm−1) 3205, 3001, 2916, 1720, 1705, 1690, 1655, 1522; MS (ESI): m/z calcd for C12H12N2O5: 264.07; found 264.90 [M + H]+, 286.90 [M + Na]+; anal. calcd for C12H12N2O5: C, 54.55; H, 4.58; N, 10.60. Found: C, 54.44; H, 4.70; N, 10.75%.
5-(3-Oxo-1-(thiophen-2-yl)butyl)pyrimidine-2,4,6(1H,3H,5H)-trione (4am)
Yield, 0.227 g (81%). Yellow solid, mp 77–79 °C (from EtOH). 1H NMR (300 MHz, DMSO-d6): δH (ppm) 2.11 (s, 3H), 3.15–3.24 (m, 1H), 3.32–3.35 (m, 1H), 3.77 (d, 1H, J = 3.0 Hz), 4.24–4.30 (m, 1H), 6.76–6.77 (m, 1H), 6.90–6.93 (m, 1H), 7.35–7.37 (m, 1H), 11.18 (s, 1H), 11.22 (s, 1H); 13C NMR (75 MHz, DMSO-d6): δC (ppm) 29.0, 34.9, 45.5, 50.8, 123.4, 124.1, 125.6, 140.9, 149.4, 168.3, 168.8, 205.4; IR (KBr) (νmax/cm−1) 3310, 3012, 2919, 1716, 1702, 1677, 1658, 1551; MS (ESI): m/z calcd for C12H12N2O4S: 280.05; found 281.00 [M + H]+, 303.00 [M + Na]+; anal. calcd for C12H12N2O4S: C, 51.42; H, 4.32; N, 9.99. Found: C, 51.31; H, 4.47; N, 9.91%.
5-(1-(Furan-2-yl)-3-oxo-3-phenylpropyl)pyrimidine-2,4,6(1H,3H,5H)-trione (4an)31
Yield, 0.247 g (76%). Brown gummy solid (after column chromatography). 1H NMR (300 MHz, CDCl3): δH (ppm) 3.50–3.55 (m, 1H), 3.97–4.13 (m, 2H). 4.51 (m, 1H), 6.09–6.18 (m, 2H), 6.93–7.51 (m, 4H), 7.94–7.96 (m, 2H), 9.39 (s, 1H), 9.53 (s, 1H); 13C NMR (75 MHz, CDCl3): δC (ppm) 35.6, 39.1, 49.8, 107.1, 110.5, 128.1, 128.6, 133.4, 136.3, 142.2, 150.3, 152.5, 168.7, 169.1, 198.1; IR (KBr) (νmax/cm−1) 3410, 3078, 2921, 1721, 1709, 1688, 1654, 1556; MS (ESI): m/z calcd for C17H14N2O5: 326.09; found 326.91 [M + H]+, 348.90 [M + Na]+; anal. calcd for C17H14N2O5: C, 62.57; H, 4.32; N, 8.59. Found: C, 62.71; H, 4.42; N, 8.44%.
5-(3-Oxo-3-phenyl-1-(thiophen-2-yl)propyl)pyrimidine-2,4,6(1H,3H,5H)-trione (4ao)
Yield, 0.273 g (80%). Brown solid, mp > 300 °C (from EtOH); 1H NMR (300 MHz, DMSO-d6): δH (ppm) 3.68–3.76 (m, 1H), 3.91 (m, 1H), 3.97–4.05 (m, 1H), 4.50 (m, 1H), 6.84–6.91 (m, 2H), 7.33–7.35 (m, 1H), 7.53–7.64 (m, 3H), 7.95–7.97 (m, 2H), 11.16 (s, 1H), 11.22 (s, 1H); 13C NMR (75 MHz, DMSO-d6): δC (ppm) 35.9, 41.8, 51.4, 124.3, 125.0, 126.3, 127.4, 128.3, 132.9, 136.0, 141.8, 150.0, 169.1, 169.4, 197.4; IR (KBr) (νmax/cm−1) 3380, 3078, 2919, 1718, 1702, 1689, 1657, 1547; MS (ESI): m/z calcd for C17H14N2O4S: 342.07; found 343.11 [M + H]+, 365.09 [M + Na]+; anal. calcd for C17H14N2O4S: C, 59.64; H, 4.12; N, 8.18. Found: C, 59.77; H, 3.98; N, 8.30%.
5-(5-Oxohexan-3-yl)pyrimidine-2,4,6(1H,3H,5H)-trione (4ap)
Yield, 0.160 g (71%). Red gummy solid (after column chromatography). 1H NMR (300 MHz, methanol-d4): δH (ppm) 0.83–0.96 (m, 3H), 1.55–1.60 (m, 2H), 2.16 (s, 3H), 2.51–2.59 (m, 1H), 2.71–2.77 (m, 2H), 3.59 (m, 1H), 11.03 (s, 2H); 13C NMR (75 MHz, methanol-d4): δC (ppm) 13.5, 22.7, 26.5, 30.8, 37.8, 84.1, 145.3, 161.7, 203.5; IR (KBr) (νmax/cm−1) 3391, 2924, 1719, 1702, 1682, 1655; MS (ESI): m/z calcd for C10H14N2O4: 226.10; found 226.23 [M]+, 249.01 [M + Na]+; anal. calcd for C10H14N2O4: C, 53.09; H, 6.24; N, 12.38. Found: C, 52.97; H, 6.11; N, 12.51%.
5-(2-Oxooctan-4-yl)pyrimidine-2,4,6(1H,3H,5H)-trione (4aq)
Yield, 0.185 g (73%). Red gummy solid (after column chromatography). 1H NMR (300 MHz, methanol-d4): δH (ppm) 0.91 (m, 3H), 1.31–1.59 (m, 6H), 2.16 (s, 3H), 2.68–2.72 (m, 1H), 2.86–2.91 (m, 2H), 3.59–3.65 (m, 1H), 10.94 (s, 2H); 13C NMR (75 MHz, methanol-d4): δC (ppm) 6.8, 22.6, 22.7, 24.7, 29.0, 32.1, 38.1, 84.5, 150.2, 161.7, 164.2, 203.4; IR (KBr) (νmax/cm−1) 3127, 2923, 1721, 1708, 1682, 1670; MS (ESI): m/z calcd for C12H18N2O4: 254.13; found 255.10 [M + H]+, 277.01 [M + Na]+; anal. calcd for C12H18N2O4: C, 56.68; H, 7.13; N, 11.02. Found: C, 56.81; H, 7.00; N, 10.89%.
5-(1-Oxo-1-phenylheptan-3-yl)pyrimidine-2,4,6(1H,3H,5H)-trione (4ar)
Yield, 0.234 g (74%). Red gummy solid (after column chromatography). 1H NMR (300 MHz, CDCl3): δH (ppm) 0.90 (m, 3H), 1.13–1.15 (m, 2H), 1.26–1.46 (m, 4H), 3.18–3.25 (m, 1H), 3.39 (m, 1H), 3.42–3.54 (m, 1H), 3.75 (m, 1H), 7.46–7.49 (m, 2H), 7.58 (m, 1H), 7.94 (d, 2H, J = 7.5 Hz), 8.25 (s, 1H), 8.29 (s, 1H); 13C NMR (75 MHz, CDCl3): δC (ppm) 7.5, 23.3, 25.6, 33.1, 42.1, 85.0, 121.7, 122.3, 127.0, 143.1, 162.3, 162.5, 162.8, 193.7; IR (KBr) (νmax/cm−1) 3210, 3052, 2923, 1722, 1709, 1687, 1661, 1558; MS (ESI): m/z calcd for C17H20N2O4: 316.14; found 316.96 [M + H]+, 339.10 [M + Na]+; anal. calcd for C17H20N2O4: C, 64.54; H, 6.37; N, 8.86. Found: C, 64.68; H, 6.27; N, 9.05%.
1,3-Dimethyl-5-(3-oxo-1-phenylbutyl)pyrimidine-2,4,6(1H,3H,5H)-trione (4ba)30
Yield, 0.251 g (83%). Yellow gummmy solid (after column chromatography). 1H NMR (300 MHz, CDCl3): δH (ppm) 2.18 (s, 3H), 2.89–2.91 (m, 1H), 2.96 (s, 3H), 3.10 (s, 3H), 3.42–3.51 (m, 1H), 3.84 (d, 1H, J = 4.2 Hz), 4.02–4.09 (m, 1H), 6.94–6.97 (m, 2H), 7.19–7.22 (m, 3H); 13C NMR (75 MHz, CDCl3): δC (ppm) 27.8, 27.9, 30.3, 44.0, 44.7, 52.5, 127.1, 128.3, 128.4, 137.5, 150.8, 167.5, 168.2, 206.4; IR (KBr) (νmax/cm−1) 3072, 2924, 1710, 1701, 1688, 1658, 1553; MS (ESI): m/z calcd for C16H18N2O4: 302.13; found 303.12 [M + H]+, 325.00 [M + Na]+; anal. calcd for C16H18N2O4: C, 63.56; H, 6.00; N, 9.27. Found: C, 63.72; H, 5.86; N, 9.38%.
5-(1-(2-Chlorophenyl)-3-oxobutyl)-1,3-dimethylpyrimidine-2,4,6(1H,3H,5H)-trione (4bb)
Yield, 0.292 g (87%). White solid, mp 93–95 °C (from EtOH/DCM).1H NMR (300 MHz, CDCl3): δH (ppm) 2.13 (s, 3H), 2.89–2.97 (m, 1H), 3.10 (s, 6H), 3.29–3.38 (m, 1H), 3.75 (d, 1H, J = 4.5 Hz), 4.59–4.65 (m, 1H), 7.11–7.29 (m, 4H); 13C NMR (75 MHz, CDCl3): δC (ppm) 28.2, 28.4, 30.0, 38.7, 45.5, 52.6, 126.9, 128.2, 128.7, 129.7, 133.8, 136.5, 151.8, 167.4, 167.5, 206.1; IR (KBr) (νmax/cm−1) 3090, 2919, 1718, 1706, 1688, 1659, 1554; MS (ESI): m/z calcd for C16H17ClN2O4: 336.09; found 337.01 [M + H]+, 359.00 [M + Na]+. Anal. calcd for C16H17ClN2O4: C, 57.06; H, 5.09; N, 8.32. Found: C, 57. 18; H, 4.95; N, 8.47%.
5-(1-(3-Chlorophenyl)-3-oxobutyl)-1,3-dimethylpyrimidine-2,4,6(1H,3H,5H)-trione (4bc)
Yield, 0.263 g (78%). Yellow gummy solid (after column chromatography). 1H NMR (300 MHz, CDCl3): δH (ppm) 2.20 (s, 3H), 2.90–3.00 (m, 1H), 3.03 (s, 3H), 3.12 (s, 3H), 3.42–3.51 (m, 1H), 3.84 (d, 1H, J = 3.9 Hz), 4.04–4.10 (m, 1H), 6.89 (d, 1H, J = 6.9 Hz), 7.01 (s, 1H), 7.14–7.26 (m, 2H); 13C NMR (75 MHz, CDCl3): δC (ppm) 28.0, 28.1, 30.3, 43.2, 44.7, 52.3, 125.5, 127.4, 128.4, 129.9, 134.6, 140.0, 150.7, 167.4, 167.9, 206.1; IR (KBr) (νmax/cm−1) 3055, 2924, 1727, 1710, 1687, 1660, 1552; MS (ESI): m/z calcd for C16H17ClN2O4: 336.09; found 337.01[M + H]+, 359.01 [M + Na]+. Anal. calcd for C16H17ClN2O4: C, 57.06; H, 5.09: N, 8.32. Found: C, 56.93; H, 4.96; N, 8.45%.
5-(1-(3-Methoxyphenyl)-3-oxobutyl)-1,3-dimethylpyrimidine-2,4,6(1H,3H,5H)-trione (4bd)
Yield, 0.282 g (85%). Yellow gummy solid (after column chromatography). 1H NMR (300 MHz, CDCl3): δH (ppm) 2.17 (s, 3H), 2.87–2.95 (m, 1H), 2.96 (s, 3H), 3.06 (s, 3H), 3.08–3.47 (m, 1H), 3.69 (s, 3H), 3.81 (d, 1H, J = 3.9 Hz), 4.01–4.02 (m, 1H), 6.50–6.53 (m, 2H), 6.72–6.74 (m, 1H), 7.09–7.14 (m, 1H); 13C NMR (75 MHz, CDCl3): δC (ppm) 27.8, 27.9, 30.2, 43.8, 44.7, 52.3, 55.0, 113.0, 113.2, 119.2, 129.5, 139.1, 150.8, 159.5, 167.5, 168.1, 206.3; IR (KBr) (νmax/cm−1) 3067, 2923, 1719, 1707, 1680, 1651, 1561; MS (ESI): m/z calcd for C17H20N2O5: 332.14; found 333.15 [M + H]+; anal. calcd for C17H20N2O5: C, 61.44; H, 6.07; N, 8.43. Found: C, 61.59; H, 6.19; N, 8.27%.
1,3-Dimethyl-5-(1-(3-nitrophenyl)-3-oxobutyl)pyrimidine-2,4,6(1H,3H,5H)-trione (4be)
Yield, 0.291 g (84%). Yellow gummy solid (after column chromatography). 1H NMR (300 MHz, CDCl3): δH (ppm) 2.18 (s, 3H), 2.83–2.91 (m, 1H), 3.02 (s, 3H), 3.11 (s, 3H), 3.48–3.57 (m, 1H), 3.89 (d, 1H, J = 3.9 Hz), 4.20–4.27 (m, 1H), 7.43–7.51 (m, 2H), 7.92 (s, 1H), 8.05–8.07 (m, 1H); 13C NMR (75 MHz, CDCl3): δC (ppm) 28.1, 28.2, 30.1, 42.2, 44.8, 52.0, 122.0, 122.9, 129.6, 134.1, 140.8, 148.1, 150.6, 167.1, 167.4, 206.0; IR (KBr) (νmax/cm−1) 3086, 2924, 1711, 1701, 1688, 1657, 1531; MS (ESI): m/z calcd for C16H17N3O6: 347.11; found 348.34 [M + H]+, 370.15 [M + Na]+; anal. calcd for C16H17N3O6: C, 55.33; H, 4.93; N, 12.10. Found: C, 55.17; H, 5.06; N, 11.98%.
5-(1-(4-Bromophenyl)-3-oxobutyl)-1,3-dimethylpyrimidine-2,4,6(1H,3H,5H)-trione (4bf)
Yield, 0.331 g (87%). Yellow gummy solid (after column chromatography). 1H NMR (300 MHz, CDCl3): δH (ppm) 2.18 (s, 3H), 2.88–2.96 (m, 1H), 3.04 (s, 3H), 3.12 (s, 3H), 3.42–3.52 (m, 1H), 3.85 (d, 1H, J = 3.9 Hz), 4.05–4.11 (m, 1H), 6.89 (d, 2H, J = 8.7 Hz), 7.34 (d, 2H, J = 8.4 Hz); 13C NMR (75 MHz, CDCl3): δC (ppm) 28.1, 28.2, 30.4, 42.8, 45.1, 52.2, 122.2, 129.1, 131.8, 137.3, 150.8, 167.5, 167.9, 206.3; IR (KBr) (νmax/cm−1) 3029, 2924, 1718, 1710, 1693, 1674, 1516; MS (ESI): m/z calcd for C16H17BrN2O4: 380.04; found 381.12 [M + H]+, 403.00 [M + Na]+; anal. calcd for C16H17BrN2O4: C, 50.41; H, 4.49; N, 7.35. Found: C, 50.34; H, 4.61; N, 7.21%.
5-(1-(4-Hydroxyphenyl)-3-oxobutyl)-1,3-dimethylpyrimidine-2,4,6(1H,3H,5H)-trione (4bg)
Yield, 0.216 g (68%). White solid, mp 173–175 °C (from EtOH). 1H NMR (300 MHz, CDCl3): δH (ppm) 2.04 (s, 3H), 2.88–2.93 (m, 1H), 3.02 (s, 3H), 3.10 (s, 3H), 3.38–3.47 (m, 1H), 3.83 (d, 1H, J = 3.9 Hz), 4.02–4.03 (m, 1H), 6.45 (s, 1H), 6.67 (d, 2H, J = 8.4 Hz), 6.81 (d, 2H, J = 8.1 Hz); 13C NMR (75 MHz, CDCl3): δC (ppm) 28.0, 28.1, 30.5, 43.6, 45.2, 52.9, 115.6, 128.4, 128.9, 151.0, 156.0, 167.7, 168.6, 207.3; IR (KBr) (νmax/cm−1) 3433, 3012, 2927, 1710, 1700, 1678, 1671, 1554; MS (ESI): m/z calcd for C16H18N2O5: 318.12; found 318.97 [M + H]+, 341.00 [M + H]+; anal. calcd for C16H18N2O5: C, 60.37; H, 5.70; N, 8.80. Found: C, 60.21; H, 5.85; N, 8.97%.
1,3-Dimethyl-5-(1-(4-nitrophenyl)-3-oxobutyl)pyrimidine-2,4,6(1H,3H,5H)-trione (4bh)
Yield, 0.292 g (84%). Yellow gummy solid (after column chromatography). 1H NMR (300 MHz, CDCl3): δH (ppm) 2.19 (s, 3H), 2.83–3.00 (m, 1H), 3.08 (s, 3H), 3.15 (s, 3H), 3.50–3.59 (m, 1H), 3.91 (d, 1H, J = 3.3 Hz), 4.27–4.29 (m, 1H), 7.27 (d, 2H, J = 8.4 Hz), 8.08 (d, 2H, J = 8.4 Hz); 13C NMR (75 MHz, CDCl3): δC (ppm) 28.2, 28.3, 30.2, 42.1, 44.9, 51.9, 123.7, 128.7, 146.4, 147.3, 150.6, 167.1, 167.3, 206.1; IR (KBr) (νmax/cm−1) 3124, 2924, 1710, 1701, 1689, 1652, 1523; MS (ESI): m/z calcd for C16H17N3O6: 347.11; found 348.24 [M + H]+, 370.01 [M + Na]+; anal. calcd for C16H17N3O6: C, 55.33; H, 4.93; N, 12.10. Found: C, 55.19; H, 4.81; N, 12.27%.
5-(1-(2-Chlorophenyl)-3-oxobutyl)-1-methylpyrimidine-2,4,6(1H,3H,5H)-trione (4bi)
Yield, 0.284 g (88%). Yellow gummy solid (after column chromatography). 1H NMR (300 MHz, CDCl3): δH (ppm) 2.12/2.13 (s‡, 3H), 2.88–2.96 (m, 1H), 3.15/3.21 (s‡, 3H), 3.27–3.31/3.33–3.38 (m‡, 1H), 3.77/3.85 (d‡, 1H, J = 4.2 Hz), 4.67–4.68 (m, 1H), 7.14–7.32 (m, 4H), 9.38/9.39 (s‡, 1H); 13C NMR (75 MHz, CDCl3): δC (ppm) 27.5/27.6, 29.9, 37.5/37.6, 38.9, 44.9, 126.8/126.9, 127.0/127.1, 128.3/128.4, 129.8/129.9, 133.6/133.7, 137.0/137.3, 150.4, 167.7, 168.1/168.2, 207.0/207.1; IR (KBr) (νmax/cm−1) 3356, 3095, 2924, 1712, 1701, 1681, 1654, 1585; MS (ESI): m/z calcd for C15H15ClN2O4: 322.07; found 323.09 [M + H]+, 345.01 [M + Na]+; anal. calcd for C15H15ClN2O4: C, 55.82; H, 4.68; N, 8.68. Found: C, 56.07; H, 4.50; N, 8.56%.
5-(1-(2-Fluorophenyl)-3-oxo-3-phenylpropyl)-1-methylpyrimidine-2,4,6(1H,3H,5H)-trione (4bj)
Yield, 0.328 g (85%). Yellow gummy solid (after column chromatography). 1H NMR (300 MHz, CDCl3): δH (ppm) 3.04/3.13 (s‡, 3H), 3.47–3.49/3.53–3.55 (m‡, 1H), 3.95–3.97 (m, 1H), 3.99–4.03/4.05–4.09 (m‡, 1H), 4.61–4.62 (m, 1H), 6.98–7.08 (m, 2H), 7.20–7.26 (m, 2H), 7.41–7.55 (m, 3H), 7.94–7.97 (m, 2H), 9.04/9.14 (s‡, 1H); 13C NMR (75 MHz, CDCl3): δC (ppm) 27.5, 37.3/37.5, 39.8/39.9, 51.9/52.0, 115.6/115.7, 115.9/116.0, 124.5, 125.6/125.8, 128.0, 128.6, 129.5/129.6, 129.7/129.8, 133.4, 136.4, 150.3/150.4, 167.8/168.0, 168.3/168.6, 197.7/197.8; IR (KBr) (νmax/cm−1) 3321, 3092, 2923, 1721, 1709, 1684, 1657, 1552; MS (ESI): m/z calcd for C20H17FN2O4: 368.12; found 369.13 [M + H]+; anal. calcd for C20H17FN2O4: C, 65.21; H, 4.65; N, 7.60. Found: C, 65.35; H, 4.51; N, 7.76%.
5-(1-(3-Chlorophenyl)-3-oxobutyl)-1-methylpyrimidine-2,4,6(1H,3H,5H)trione (4bk)
Yield, 0.284 g (88%). Yellow gummy solid (after column chromatography). 1H NMR (300 MHz, CDCl3): δH (ppm) 2.20/2.21 (s‡, 3H), 2.92–3.00 (m, 1H), 3.04/3.13 (s‡, 3H), 3.50–3.59 (m, 1H), 3.88–3.92 (m‡, 1H), 4.08–4.16 (m, 1H), 7.01–7.29 (m, 4H), 9.16/9.24 (s‡, 1H); 13C NMR (75 MHz, CDCl3): δC (ppm) 27.3/27.4, 30.2, 42.0/42.1, 44.7/44.9, 51.7/51.8, 125.8/126.0, 127.7/127.8, 128.24, 130.0, 134.5, 140.5, 150.0/150.1, 167.7/168.0, 168.3/168.6, 206.9; IR (KBr) (νmax/cm−1) 3341, 3088, 2924, 1719, 1710, 1689, 1660, 1552; MS (ESI): m/z calcd for C15H15ClN2O4: 322.07; found 323.15 [M + H]+, 345.10 [M + Na]+; anal. calcd for C15H15ClN2O4: 55.82; H, 4.68; N, 8.68. Found: C, 55.95; H, 4.51; N, 8.74%.
5-(1-(4-Bromophenyl)-3-oxobutyl)-1-methylpyrimidine-2,4,6(1H,3H,5H)-trione (4bl)
Yield, 0.334 g (91%). Yellow gummy solid (after column chromatography). 1H NMR (300 MHz, CDCl3): δH (ppm) 2.17/2.18 (s‡, 3H), 2.88–2.96 (m, 1H), 3.03/3.12 (s‡, 3H), 3.47–3.52/3.53–3.58 (m‡, 1H), 3.87–3.91 (m‡, 1H), 4.07–4.14 (m, 1H), 6.96–7.02 (m‡, 2H), 7.36–7.43 (m‡, 2H), 9.11/9.15 (s‡, 1H); 13C NMR (75 MHz, CDCl3): δC (ppm) 28.6, 30.4, 41.8/41.9, 45.0/45.1, 51.7/51.8, 122.1, 129.5, 132.0, 137.5, 150.1/150.2, 167.9/168.2, 168.4/168.7, 207.2; IR (KBr) (νmax/cm−1): 3323, 3097, 2924, 1719, 1702, 1689, 1658, 1551; MS (ESI): m/z calcd for C15H15BrN2O4: 366.02; found 367.01 [M + H]+, 389.00 [M + Na]+; anal. calcd for C15H15BrN2O4: C, 49.06; H, 4.12; N, 7.63. Found: C, 48.91; H, 3.98; N, 7.78%.
Acknowledgements
H. M. and S. J. K. thank the DST, Govt. of India for the financial assistance under the DST-INSPIRE Faculty scheme. The authors also acknowledge SAIF-GU, SAIF-NEHU and IIT-Guwahati for sample analyses.
Notes and references
- For selected examples
(a) Y. Gu, Green Chem., 2012, 14, 2091–2128 RSC;
(b) M.-O. Simon and C.-J. Li, Chem. Soc. Rev., 2012, 41, 1415–1427 RSC;
(c) R. N. Butler and A. G. Coyne, Chem. Rev., 2010, 110, 6302–6337 CrossRef CAS PubMed;
(d) N. Shapiro and A. Vigalok, Angew. Chem., Int. Ed., 2008, 120, 2891–2894 CrossRef;
(e) H. C. Hailes, Org. Process Res. Dev., 2007, 11, 114–120 CrossRef CAS;
(f) C.-J. Li, Chem. Rev., 2005, 105, 3095–3165 CrossRef CAS PubMed;
(g) M. C. Pirrung and K. D. Sarma, J. Am. Chem. Soc., 2004, 126, 444–445 CrossRef CAS PubMed;
(h) U. M. Lindström, Chem. Rev., 2002, 102, 2751–2772 CrossRef PubMed;
(i) S. Kobayashi and K. Manabe, Acc. Chem. Res., 2002, 35, 209–217 CrossRef CAS PubMed;
(j) Organic Synthesis in Water, ed. P. A. Grieco, Thomson Science, Glassgow, Scotland, 1998 Search PubMed;
(k) C.-J. Li and T. H. Chan, Organic Reactions in Aqueous Media, Wiley, New York, 1997 Search PubMed.
- For representative examples see
(a) H. Pellisier, Chem. Rev., 2013, 113, 442–524 CrossRef PubMed;
(b) S. Brauch, S. S. van Berkel and B. Westermann, Chem. Soc. Rev., 2013, 42, 4948–4962 RSC;
(c) A. Dömling, W. Wang and K. Wang, Chem. Rev., 2012, 112, 3083–3135 CrossRef PubMed;
(d) E. Ruijter, R. Scheffelaar and R. V. A. Orru, Angew. Chem., Int. Ed., 2011, 50, 6234–6246 CrossRef CAS PubMed;
(e) J. D. Sunderhaus and S. F. Martin, Chem. – Eur. J., 2009, 15, 1300–1308 CrossRef CAS PubMed;
(f) N. Isambert and R. Lavilla, Chem. – Eur. J., 2008, 14, 8444–8454 CrossRef CAS PubMed;
(g) P. A. Clarke, S. Santos and W. C. H. Martin, Green Chem., 2007, 9, 438–440 RSC;
(h) A. Dömling, Chem. Rev., 2006, 106, 17–89 CrossRef PubMed.
-
(a) Y. Yang, W.-M. Shu, S.-B. Yu, F. Ni, M. Gao and A.-X. Wu, Chem. Commun., 2013, 49, 1729–1731 RSC;
(b) Y.-Q. Zhang, D.-Y. Zhu, B.-S. Li, Y.-Q. Tu, J.-X. Liu, Y. Lu and S.-H. Wang, J. Org. Chem., 2012, 77, 4167–4170 CrossRef CAS PubMed;
(c) M.-Z. Wang, C.-Y. Zhou and C.-M. Che, Chem. Commun., 2011, 47, 1312–1314 RSC;
(d) K. Takasu, T. Tanaka, T. Azuma and Y. Takemoto, Chem. Commun., 2010, 46, 8246–8248 RSC.
- For more examples see
(a) C. Jing, T. Shi, D. Xing, X. Gou and W.-H. Hu, Green Chem., 2013, 15, 620–624 RSC;
(b) P. P. Ghosh, G. Pal, S. Paul and A. R. Das, Green Chem., 2012, 14, 2691–2698 RSC;
(c) P. Prasanna, K. Balamurugan, S. Perumal and J. C. Menéndez, Green Chem., 2011, 13, 2123–2129 RSC;
(d) M. W. Powner, J. D. Sutherland and J. W. Szostak, J. Am. Chem. Soc., 2010, 132, 16677–16688 CrossRef CAS PubMed;
(e) K. Kumaravel and G. Vasuki, Curr. Org. Chem., 2009, 13, 1820–1841 CrossRef CAS.
- For special reviews see
(a) K. Gopalaiah, Chem. Rev., 2013, 113, 3248–3296 CrossRef CAS PubMed;
(b) C.-X. Wang and B.-S. Wan, Chin. Sci. Bull., 2012, 57, 2338–2351 CrossRef CAS;
(c) C.-L. Sun, B.-J. Li and Z.-J. Shi, Chem. Rev., 2011, 111, 1293–1314 CrossRef CAS PubMed;
(d) W. M. Czaplik, M. Mayer, J. Cvengroš and A. J. von Wangelin, ChemSusChem, 2009, 2, 396–417 CrossRef CAS PubMed;
(e) Iron Catalysis in Organic Chemistry: Reactions and Applications, ed. B. Plietker, Wiley-VCH, Weinheim, 2008 Search PubMed;
(f) B. D. Sherry and A. Fürstner, Acc. Chem. Res., 2008, 41, 1500–1511 CrossRef CAS PubMed;
(g) A. Correa, O. G. Mancheño and C. Bolm, Chem. Soc. Rev., 2008, 37, 1108–1117 RSC;
(h) C. Bolm, J. Legros, J. L. Paih and L. Zani, Chem. Rev., 2004, 104, 6217–6254 CrossRef CAS PubMed.
- For recent examples on FeCl3·6H2O catalyzed reactions
(a) A. R. Mohite and R. G. Bhat, Org. Lett., 2013, 15, 4564–4567 CrossRef CAS PubMed;
(b) Z. Wang, S. Li, B. Yu, H. Wu, Y. Wang and X. Sun, J. Org. Chem., 2012, 77, 8615–8620 CrossRef CAS PubMed;
(c) Q. Yang, L. Wang, T. Gou and Z. Yu, J. Org. Chem., 2012, 77, 8355–8361 CrossRef CAS PubMed;
(d) H. S. P. Rao and S. Vijjapu, RSC Adv., 2012, 2, 6773–6783 RSC;
(e) L. Yang, C.-H. Lei, D.-X. Wang, Z.-T. Huang and M.-X. Wang, Org. Lett., 2010, 12, 3918–3921 CrossRef CAS PubMed;
(f) G. Chai, Z. Lu, C. Fu and S. Ma, Adv. Synth. Catal., 2009, 351, 1946–1954 CrossRef CAS;
(g) P. O. Miranda, D. D. Díaz, J. I. Padrón, J. Bermejo and V. S. Martín, Org. Lett., 2003, 11, 1979–1982 CrossRef PubMed.
-
(a) P. R. Andrews, L. C. Mark, D. A. Winkler and G. P. Jones, J. Med. Chem., 1983, 26, 1223–1229 CrossRef CAS;
(b) P. R. Andrews, G. P. Jones and D. B. Poulton, Eur. J. Pharmacol., 1982, 79, 61–65 CrossRef CAS;
(c) A. Dhasmana, J. P. Barthwal, B. R. Pandey and B. Ali, J. Heterocycl. Chem., 1981, 18, 635–637 CrossRef CAS;
(d) R. L. Mcdonald and J. L. Barker, Neurology, 1979, 4, 432–437 CrossRef.
-
(a) F. López-Muñoz, R. Ucha-Udabe and C. Alamo, Neuropsychiatr. Dis. Treat., 2005, 4, 329–343 Search PubMed;
(b) W. W. Morgan, Adv. Alcohol Subst. Abuse, 1990, 9, 67–82 CrossRef CAS PubMed;
(c) D. E. McMillan and G. R. Wenger, J. Exp. Anal. Behav., 1983, 40, 133–142 CrossRef CAS PubMed.
-
(a) E. Maquoi, N. E. Sounni, L. Devy, F. Olivier, F. Frankenne, H.-W. Krell, F. Grams, J.-M. Foidart and A. Nöel, Clin. Cancer Res., 2004, 10, 4038–4047 CrossRef CAS PubMed;
(b) G. G. Gomez, R. B. Hutchison and C. A. Kruse, Cancer Treat. Rev., 2001, 27, 375–402 CrossRef CAS PubMed;
(c) K. S. Gulliya, US Pat., 5869494, 1999.
-
(a) H. Lee, M. Y. Berezin, K. Gou, J. Kao and S. Achilefu, Org. Lett., 2009, 11, 29–32 CrossRef CAS PubMed;
(b) R. J. Bartzatt, J. Pharm. Biomed. Anal., 2002, 29, 909–915 CrossRef CAS.
- For examples see
(a) B. B. Ivanova and M. Spitellar, Cryst. Growth Des., 2010, 10, 2470–2474 CrossRef CAS;
(b) R. Andreu, J. Garín, J. Orduna, R. Alcalá and B. Villacampa, Org. Lett., 2003, 5, 3143–3146 CrossRef CAS PubMed.
-
(a) S. Yagai, T. Karatsu and A. Kitamura, Langmuir, 2005, 21, 11048–11052 CrossRef CAS PubMed;
(b) N. D. McClenaghan, C. Absalon and D. M. Bassani, J. Am. Chem. Soc., 2003, 125, 13004–13005 CrossRef CAS PubMed;
(c) P. Timmerman and L. J. Prins, Eur. J. Org. Chem., 2001, 3191–3205 CrossRef CAS;
(d) L. J. Prins, K. A. Jolliffe, R. Hulst, P. Timmerman and D. N. Reinhoudt, J. Am. Chem. Soc., 2000, 122, 3617–3627 CrossRef CAS.
-
(a) J. C. Ruble, A. R. Hurd, T. A. Johnson, D. A. Sherry, M. R. Barbachyn, P. L. Toogood, G. L. Bundy, D. R. Graber and G. M. Kamilar, J. Am. Chem. Soc., 2009, 131, 3991–3997 CrossRef CAS PubMed;
(b) A. A. Miller, G. L. Bundy, J. E. Mott, J. E. Skepner, T. P. Boyle, D. W. Harris, A. E. Hromockyj, K. R. Marotti, G. E. Zurenko, J. B. Munzner, M. T. Sweeney, G. F. Bammert, J. C. Hamel, C. W. Ford, W.-Z. Zhong, D. R. Graber, G. E. Martin, F. Han, L. A. Dolak, E. P. Seest, J. C. Ruble, G. M. Kamilar, J. R. Palmer, L. S. Banitt, A. R. Hurd and M. R. Barbachyn, Antimicrob. Agents Chemother., 2008, 52, 2806–2812 CrossRef CAS PubMed.
-
(a) J. Wang, S. O'Sullivan, S. Harmon, R. Keaveny, M. W. Radomski, C. Medina and J. F. Gilmer, J. Med. Chem., 2012, 55, 2154–2162 CrossRef CAS PubMed;
(b) A. Faust, B. Waschkau, J. Waldeck, C. Höltke, H.-J. Breyholz, S. Wagner, K. Kopka, W. Heindel, M. Schäfers and C. Bremer, Bioconjugate Chem., 2008, 19, 1001–1008 CrossRef CAS PubMed;
(c) J. F. Fisher and S. Mobashery, Cancer Metastasis Rev., 2006, 25, 115–136 CrossRef CAS PubMed;
(d) R. Fridman, Cancer Metastasis Rev., 2006, 25, 7–8 CrossRef;
(e) H. Brandstetter, F. Grams, D. Glitz, A. Lang, R. Huber, W. Bode, H.-W. Krell and R. A. Engh, J. Biol. Chem., 2001, 276, 17405–17412 CrossRef CAS PubMed.
- G. Xia, R. Benmohamed, J. Kim, A. C. Arvanites, R. I. Morimoto, R. J. Ferrante, D. R. Kirsch and R. B. Silverman, J. Med. Chem., 2011, 54, 2409–2421 CrossRef CAS PubMed.
-
(a) I. Devi and P. J. Bhuyan, Tetrahedron Lett., 2005, 46, 5727–5729 CrossRef CAS PubMed;
(b) Weygand-Hilgetag Preparative Organic Chemistry, ed. G. Hilgetag and A. Martini, Wiley, New York, 1972, p. 493 Search PubMed.
- For examples see
(a) B. M. Trost and G. M. Schroeder, J. Org. Chem., 2000, 65, 1569–1573 CrossRef CAS;
(b) B. M. Trost and G. M. Schroeder, J. Am. Chem. Soc., 1999, 121, 6759–6760 CrossRef CAS;
(c) B. M. Trost, R. Radinov and E. M. Grenzer, J. Am. Chem. Soc., 1997, 119, 7879–7880 CrossRef CAS;
(d) H. Brunner and J. Fürst, Inorg. Chim. Acta, 1994, 220, 63–66 CrossRef CAS;
(e) A. P. Centolella, J. W. Nelson and H. G. Kolloff, J. Am. Chem. Soc., 1943, 65, 2091–2092 CrossRef CAS.
-
(a) B. S. Jursic and E. D. Stevens, Tetrahedron Lett., 2003, 44, 2203–2210 CrossRef CAS;
(b) D. M. Neumann, B. S. Jursic and K. L. Martin, Tetrahedron Lett., 2002, 43, 1603–1606 CrossRef CAS.
- C. Löfberg, R. Grigg, A. Keep, A. Derrick, V. Sridharan and C. Kilner, Chem. Commun., 2006, 5000–5002 RSC.
- P. Singh and K. Paul, J. Heterocycl. Chem., 2006, 43, 607–612 CrossRef CAS.
-
(a) A. Volonterio and M. Zanda, J. Org. Chem., 2008, 73, 7486–7497 CrossRef CAS PubMed;
(b) A. Volonterio and M. Zanda, Org. Lett., 2007, 9, 841–844 CrossRef CAS PubMed.
- L. P. Zalukaev and V. L. Trostyanetskaya, Khim. Geterotsikl. Soedin., 1971, 6, 836–837 (Chem. Heterocycl. Compd., 1971, 7, 781–782) Search PubMed.
- A. N. Osman, A. A. El-Gendy, M. M. Kandeel, E. M. Ahmed and M. M. M. Hussein, Bull. Fac. Pharm., 2003, 41, 59–68 (Chem. Abstr., 2004, 143, 286367) CAS.
-
(a) B. Myrboh, H. Mecadon, M. R. Rumum, M. Rajbangshi, I. Kharkongor, B. M. Laloo, I. Kharbangar and B. Kshiar, Org. Prep. Proced. Int., 2013, 45, 253–303 CrossRef CAS;
(b) M. R. Rumum, H. Mecadon, A. T. Khan and B. Myrboh, Tetrahedron Lett., 2012, 53, 5261–5264 CrossRef PubMed.
- For examples see
(a) M. J. Shanmugam and T. M. Das, Carbohydr. Res., 2013, 368, 40–46 CrossRef CAS PubMed;
(b) R. M. Shaker and M. A. Elrady, Z. Naturforsch., B: J. Chem. Sci., 2008, 63, 1431–1437 CAS;
(c) W. S. Hamama, M. A. Ismail, H. A. Al-Saman and H. H. Zoorob, Z. Naturforsch., B: J. Chem. Sci., 2007, 62, 104–110 CAS;
(d) V. A. Chebanov, V. E. Saraev, E. A. Gura, S. M. Desenko and V. I. Mustov, Collect. Czech. Chem. Commun., 2005, 70, 350–360 CrossRef CAS;
(e) T. J. Delia, The Chemistry of Heterocyclic Compounds: Fused Pyrimidines, Wiley and Sons, 1992, ch. 1, pp. 17–24 Search PubMed.
- ESI.†.
-
(a) S. Daqing, S. Jingwen and R. Shaofeng, Chin. J. Chem., 2010, 28, 791–796 CrossRef;
(b) N. N. Kolos, V. A. Chebanov and V. D. Orlov, Khim. Geterotsikl. Soedin., 1998, 9, 1230–1233 (Chem. Heterocycl. Compd., 1999, 35, 1085–1088) Search PubMed;
(c) E. C. Taylor Jr and C. K. Cain, J. Am. Chem. Soc., 1949, 71, 2282–2284 CrossRef.
- A. I. Vogel, in Vogel's Text Book of Practical Organic Chemistry, 5th edn, ed. B. S. Furniss, A. J. Hannaford, P. W. G. Smith and A. R. Tatchell, Longman Group UK, 1989, ch. 6.12, pp. 1033–1034 Search PubMed.
- A. I. Vogel, in Vogel's Text Book of Practical Organic Chemistry, 5th edn, ed. B. S. Furniss, A. J. Hannaford, P. W. G. Smith and A. R. Tatchell, Longman Group UK, 1989, ch. 8.4, p. 1176 Search PubMed.
- M. Fujii, Y. Terao and M. Sekiya, Chem. Pharm. Bull., 1974, 22, 2675–2679 CrossRef CAS.
- V. G. Kul'nevich, Nauchn. Tr., Kuban. Gos. Univ., 1977, 256, 57–64 (Chem Abstr., 1978, 89, 24245) CAS.
Footnote |
† Electronic supplementary information (ESI) available: Copies of 1H- and 13C-NMR spectra of all compounds. CCDC 959094. For ESI and crystallographic data in CIF or other electronic format see DOI: 10.1039/c4ra00093e |
|
This journal is © The Royal Society of Chemistry 2014 |