DOI:
10.1039/C3RA46709K
(Paper)
RSC Adv., 2014,
4, 10514-10518
Basic isoreticular nanoporous metal–organic framework for Biginelli and Hantzsch coupling: IRMOF-3 as a green and recoverable heterogeneous catalyst in solvent-free conditions†
Received
15th November 2013
, Accepted 20th December 2013
First published on 20th December 2013
Abstract
The IRMOF-3 metal–organic framework (MOF) acts as a suitable green catalyst for the one-pot synthesis of dihydropyrimidinone and dihydropyridine derivatives through the Biginelli and Hantzsch reactions, and the desired products were obtained in high yields with short reaction times under mild solvent-free conditions. The MOFs can be isolated from the reaction mixture and reused.
Introduction
Organic–inorganic hybrid porous coordination polymers, which are called metal–organic frameworks (MOFs), have received much attention from both scientific and commercial aspects.1 MOF nanoreactor-assisted organic synthesis using nano-channels has been utilized not only to accelerate a number of synthetic reactions, but also is a green catalyst to increase reaction rate and yields.2 MOF-based structures demonstrate various advantages such as large surface areas, adjustable pore sizes, and the simplicity of processability, tunability and stable alternative materials.2,3 MOFs have been utilized as solid catalysts in Friedel–Crafts alkylation and acylation, Sonogashira, alkene epoxidation, aldol, Suzuki, aza-Michael and Knoevenagel condensation or reactions.4–7 Recently, thermally and chemically stable MOFs/NH2 have been reported and used as heterogeneous catalysts by Gascon, Xamena, Garcia, Corma and others.7–9 In these investigations, IRMOF-3 with non-coordinated amino groups demonstrates that the basicity of the aniline-like amino group is enhanced when incorporated inside the pores of MOF channels.8,9
Dihydropyrimidinones (DHPMs) have emerged as integral backbones of several calcium channel blockers, antihypertensives and a1a adrenergic antagonists. In addition, dihydropyridines (DHPs) are among the most widely used drugs (Fig. 1). These heterocyclic rings are also known as neuroprotectants, are involved in the anti-platelet treatment of aggregators and are important in Alzheimer's disease as anti-ischemic agents.10
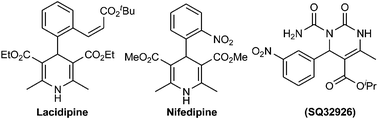 |
| Fig. 1 Examples of biologically active DHPM and DHP derivatives. | |
For these reasons, and after our recent report about the interesting catalytic properties of IRMOF-3 in our continuing interest in the synthesis and application of these organic–inorganic hybrid nanoporous materials in catalysis and coupling reactions,7,11 we herein report our results for the application of IRMOF-3 as a suitable, efficient and green catalyst for the multicomponent Biginelli and Hantzsch condensation of aldehydes and acyclic β-diketones with ammonia or urea without any solvent, salts and additives, with good to excellent yields of DHPs and DHPMs of biological and medicinal interest (Fig. 2).
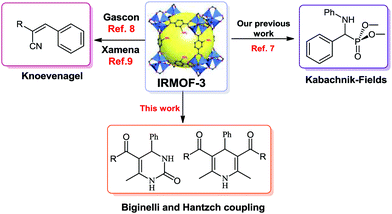 |
| Fig. 2 IRMOF-3 as a porous solid organic–inorganic hybrid catalyst. | |
Experimental
Chemicals and apparatus
All reagents were obtained from Merck (Germany) and Fluka (Switzerland) and were used without further purification. Melting points were measured on an Electrothermal 9100 apparatus. The progress of reactions was monitored by Thin Layer Chromatography (TLC). 1H and 13C NMR spectra were measured (DMSO-d6 and CDCl3 respectively) with a Bruker DRX-500 AVANCE spectrometer at 500.13 and 125.7 MHz, respectively. IR spectra were recorded on a Shimadzu IR-460 spectrometer.
Preparation of IRMOF-3
IRMOF-3 (Zn4O(H2N-TA)3) was synthesized according to a procedure from the literature with slight modifications.7,8 In a typical catalyst preparation, Zn(NO3)2 (12.5 mmol) and H2ATA (4.1 mmol) were dissolved in dry DMF and stirred for 20 min at room temperature. The solution was transferred and sealed in a teflon-lined autoclave, and kept at 100 °C for 20 h. The resulting brown solid was collected and washed with DMF and CHCl3, and the solid was finally dried in a vacuum at 60 °C.
Experimental procedure and selected data for DHPMs or DHPs
A mixture of an aldehyde (1 mmol), β-dicarbonyl compound (1 mmol for DHPMs and 2 mmol for DHPs) and urea or NH4OAc (1.2 mmol) with a catalytic amount of IRMOF-3 (0.04 g, 5 mol%), under solvent-free conditions, was stirred at 80 °C. After the complete disappearance of the starting material as indicated by TLC, the resulting mixture was diluted with hot ethyl acetate or ethanol (10 mL) and filtered. The catalyst was completely recovered from the residue. Then the reaction mixture was cooled to 25 °C and the solid was washed with cooled water and petroleum ether–ether. The products are known compounds, and their structures were deduced by comparison of their physical and spectroscopic data with those previously reported.10
Methyl 6-methyl-2-oxo-4-phenyl-1,2,3,4-tetrahydropyrimidine-5-carboxylate (3a)
IR (KBr, νmax/cm−1): 3320, 3315, 1700, 1661, 1585, 1420. 1H NMR: δ 2.36 (s, CH3), 3.63 (s, OCH3), 5.41 (d, 3JHH = 2.1 Hz), 5.53 (br, NH), 7.25–7.34 (m, C6H5), 7.56 (br, NH). 13C NMR: δ 14.08, 55.78, 60.36, 101.43, 126.49, 128.03, 128.81, 143.55, 146.21, 152.79, 166.04.
Ethyl 4-(4-chlorophenyl)-6-methyl-2-oxo-1,2,3,4-tetrahydropyrimidine-5-carboxylate (3h)
IR (KBr, νmax/cm−1): 3290, 3185, 1692, 1641, 1533, 1433. 1H NMR: δ 1.17 (d, 3JHH = 7.1 Hz, OCH2CH3), 2.33 (s, CH3), 4.08 (d, 3JHH = 7.1 Hz, OCH2CH3), 5.37 (d, 3JHH = 2.0 Hz, CHNH), 6.08 (broad, NH), 7.24 (d, 3JHH = 8.1 Hz, 2 CH of C6H4), 7.28 (d, 3JHH = 8.1 Hz, 2 CH of C6H4), 8.26 (broad, NHCH). 13C NMR: δ 14.17, 18.64, 55.12, 60.14, 101.17, 128.03, 128.88, 132.16, 142.24, 146.47, 153.39, 165.44.
Methyl 6-methyl-4-(4-nitrophenyl)-2-oxo-1,2,3,4-tetrahydropyrimidine-5-carboxylate (3i)
IR (KBr, νmax/cm−1): 3345, 3090, 1706, 1631, 1507, 1425. 1H NMR: δ 2.45 (s, CH3), 3.73 (s, OCH3), 5.60 (s, CHNH), 5.75 (broad, NH), 7.58 (d, 3JHH = 7.6 Hz, 2 CH of C6H4), 7.26 (d, 3JHH = 7.6 Hz, 2 CH of C6H4), 8.37 (broad, NHCH).
Dimethyl 2,6-dimethyl-4-phenyl-1,4-dihydropyridine-3,5-dicarboxylate (4a)
IR (KBr, νmax/cm−1): 3287, 1700, 1656, 1463, 1213, 1105. 1H NMR: δ 2.28 (s, 6H), 3.54 (s, 6H), 4.89 (s, 1H), 7.09–7.45 (m, 5H), 8.87 (s, 1H). 13C NMR: δ 18.47, 37.27, 51.10, 101.23, 127.27, 127.31, 128.37, 129.69, 146.13, 148.29, 168.64.
Dimethyl 4-(4-chlorophenyl)-2,6-dimethyl-1,4-dihydropyridine-3,5-dicarboxylate (4d)
IR (KBr, νmax/cm−1): 3329, 1697, 1650, 1470, 1219, 1127. 1H NMR: δ 2.35 (s, 6H), 3.67 (s, 6H), 4.99 (s, 1H), 5.73 (s, 1H), 7.19–7.28 (m, 4H). 13C NMR: δ 19.56, 39.01, 50.99, 103.73, 128.14, 129.07, 131.84, 144.22, 145.98, 167.81.
Diethyl 2,6-dimethyl-4-phenyl-1,4-dihydropyridine-3,5-dicarboxylate (4f)
IR (KBr, νmax/cm−1): 3342, 1700, 1657, 1473, 1198, 1129. 1H NMR: δ 1.23 (t, 3JHH = 7.0 Hz, 6H), 2.34 (s, 6H), 4.12 (q, 3JHH = 7.0 Hz, 4H), 4.91 (s, 1H), 5.68 (s, 1H), 7.07–7.43 (m, 5H). 13C NMR: δ 14.91, 19.56, 39.43, 59.82, 103.14, 127.25, 127.32, 128.34, 129.61, 146.19, 148.25, 167.34.
Diethyl 4-(3-chlorophenyl)-2,6-dimethyl-1,4-dihydropyridine-3,5-dicarboxylate (4h)
IR (KBr, νmax/cm−1): 3320, 1690, 1642, 1475, 1200, 1136. 1H NMR: δ 1.25 (t, 3JHH = 7.1 Hz, 6H), 2.37 (s, 6H), 4.13 (q, 3JHH = 7.1 Hz, 4H), 4.99 (s, 1H), 5.62 (s, 1H), 7.11–7.29 (m, 4H). 13C NMR: δ 15.18, 19.61, 39.74, 59.81, 103.82, 126.25, 126.31, 128.29, 129.04, 1433.60, 144.02, 149.73, 167.30.
Diethyl 4-(4-chlorophenyl)-2,6-dimethyl-1,4-dihydropyridine-3,5-dicarboxylate (4i)
IR (KBr, νmax/cm−1): 3345, 1695, 1649, 1480, 1210, 1137. 1H NMR: δ 1.22 (t, 3JHH = 7.2 Hz, 6H), 2.33 (s, 6H), 4.09 (q, 3JHH = 7.2 Hz, 4H), 4.96 (s, 1H), 5.65 (s, 1H), 7.17 (d, 3JHH = 8.4 Hz, 2H), 7.21 (d, 3JHH = 8.4 Hz, 2H). 13C NMR: δ 14.26, 19.58, 39.31, 59.80, 103.10, 128.31, 129.42, 131.73, 143.88, 146.32, 167.39.
Diethyl 2,6-dimethyl-4-(4-nitrophenyl)-1,4-dihydropyridine-3,5-dicarboxylate (4j)
IR (KBr, νmax/cm−1): 3324, 1695, 1647, 1473, 1205, 1131. 1H NMR: δ 1.24 (t, 3JHH = 7.1 Hz, 6H), 2.31 (s, 6H), 4.19 (q, 3JHH = 7.1 Hz, 4H), 4.76 (s, 1H), 5.67 (s, 1H), 7.53 (d, 3JHH = 7.9 Hz, 2H), 8.12 (d, 3JHH = 8.4 Hz, 2H). 13C NMR: δ 14.81, 19.56, 39.37, 59.84, 103.41, 124.10, 128.73, 145.50, 146.92, 156.30, 167.32%.
Catalyst synthesis and characterization
IRMOF-3 was prepared by refluxing 2-aminoterephthalic acid (H2ATA) and zinc nitrate hexahydrate in dry DMF by a solvothermal method, according to a literature procedure.7–9 In a typical catalyst preparation, Zn(NO3)2 (3.75 g) and H2ATA (0.75 g) were dissolved in N,N-dimethylformamide (DMF) and the mixture was transferred to a teflon-lined autoclave. The mixture of the reaction was kept at 25 °C for 1 h and then at 100 °C for 17 h. The resulting brown solid was collected and washed thrice with CHCl3, and the solid was finally dried in a vacuum at 60 °C. The IRMOF-3 structure is made of Zn4O tetranuclear clusters connected by rigid NH2–benzenedicarboxylic linkers to generate a cubic framework (Fig. 3).
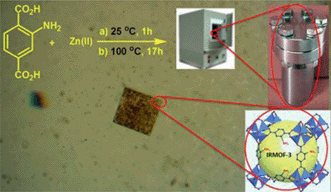 |
| Fig. 3 Preparation process of the IRMOF-3 nanoreactor. | |
IRMOF-3 was then characterized using a variety of different techniques. The IR spectrum of IRMOF-3 shows two peaks at 3470 and 3359 cm−1 due to the existence of the amino groups of the NH2–TA ligand.7 The two sharp bands at 1575 and 1385 cm−1 correspond to asymmetric (υas(C–O)) and symmetric (υs(C–O)) vibrations of the carboxyl groups, respectively,7 and the peaks centered at 1656, 1496, and 1421 cm−1 can be ascribed to C
C stretching vibrations of the aromatic ring. The 1257 cm−1 frequency can be assigned to C–N vibrations (Fig. 4c).
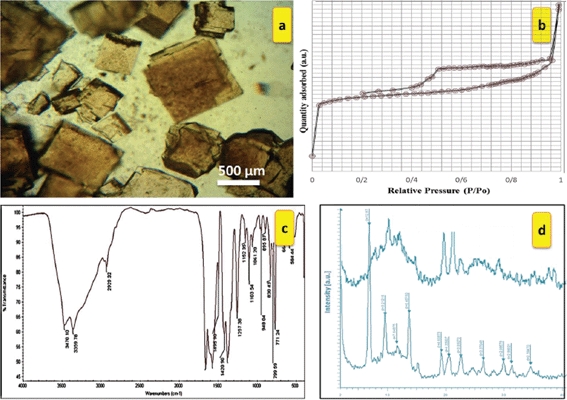 |
| Fig. 4 IRMOF-3: (a) optical microscope photograph, (b) nitrogen adsorption–desorption isotherms, (c) FT-IR pattern, and (d) XRD patterns (red: newly prepared; black: used four times to prepare 3a]. | |
Diffraction peaks at around 2θ = 6.8° and 9.6° are readily recognized from the XRD pattern (Fig. 3d). The observed diffraction peaks agree with those for IRMOF-3 reported previously.7–9 The crystal structure of IRMOF-3 seems to change to amorphous after being reused four times. The nitrogen adsorption–desorption isotherms of the solvothermally synthesized IRMOF-3 are displayed in Fig. 3b. The optical microscope photograph and SEM images (Fig. 4a and 5a), together with the XRD patterns, show that the IRMOF-3 is highly crystalline.
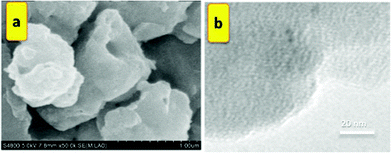 |
| Fig. 5 IRMOF-3: (a) scanning electron microscopy (SEM) and (b) transmission electron microscopy (TEM) images. | |
Results and discussion
A few multicomponent reactions using MOF catalysts have been reported.7,12 Among the new catalytic systems, Cd-MOFs as catalytic candidates have been employed under heterogeneous conditions for the synthesis of DHPMs.12 In addition, channels of MOF materials acting as nanoscale reaction vessels (nanoreactors) have been used in the last decade.2–7 First, we fabricated IRMOF-3 and then we investigated it in the multicomponent coupling reactions both for reactivity and efficiency, and also for heterogeneity and reusability capacities based on green chemistry principles. To our surprise, IRMOF-3 acts as a heterogeneous catalyst for the synthesis of DHPMs and DHPs in high yields with a short reaction time under mild solvent-free conditions. Moreover, the IRMOF-3 catalysts may be readily recovered after the reaction and reused for many cycles. As expected, when the adduct of the β-diketone and aldehyde was treated with urea under the reaction conditions in the presence of IRMOF-3, DHPMs were obtained as the products, as the Biginelli coupling reaction.10 For our study, methyl acetoacetate, benzaldehyde and urea were chosen as the benchmark substrates in the model reaction (Fig. 6).
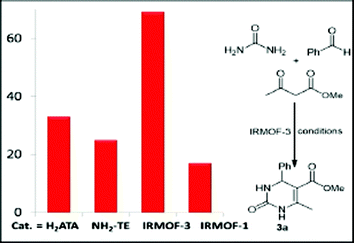 |
| Fig. 6 Reaction scale: urea (2.2 mmol), benzaldehyde (2 mmol), methyl acetoacetate (2 mmol) and catalyst (4 mol%) for 2 h. | |
Due to the fact that the nature of the amine-decorated MOFs (IRMOF-3) or its synthetic components may play an important role in the synthesis of 3a, in comparison with the similar-structured non-amine isostructural MOF (IRMOF-1), the initial reaction was carried out using 4 mol% H2ATA, 2-aminoterephthalic ester (NH2–TE), IRMOF-3 and IRMOF-1 catalysts at 60 °C. The IRMOF-3 was assessed for its catalytic activity in the Biginelli coupling by studying the model reaction to produce 3a as the principal product. When the model reaction was run using IRMOF-3, the product 3a was obtained at 69% yield in 2 h.
In the model reaction, to obtain the desired product (3a), we tested the reaction using different conditions. The model reaction was screened in polar and nonpolar solvents using 4% mol IRMOF-3. The obtained results are summarized in Table 1. It may be emphasized that in this green waste-free combined catalytic system, not a trace of byproducts was observed in the reaction by TLC analysis.
Table 1 Different conditions for the synthesis of DHPM 3a
MOF |
Mol% cat. |
Solventa |
Time (h) |
Temp (°C) |
% Yieldb |
Solvents (3 mL). Isolated yield. Solvent-free conditions. |
IRMOF-3 |
— |
DMF |
8 |
70 |
13 |
IRMOF-3 |
10 |
DMF |
8 |
70 |
73 |
IRMOF-3 |
10 |
Cyclohexane |
8 |
70 |
45 |
IRMOF-3 |
10 |
EtOH |
8 |
70 |
63 |
IRMOF-3 |
10 |
n-Hexane |
8 |
70 |
40 |
IRMOF-3 |
10 |
MeOH |
8 |
70 |
65 |
IRMOF-3 |
2 |
Solvent-freec |
8 |
60 |
85 |
IRMOF-3 |
6 |
Solvent-freec |
8 |
60 |
93 |
IRMOF-3 |
4 |
Solvent-freec |
6 |
60 |
93 |
IRMOF-3 |
4 |
Solvent-freec |
5 |
60 |
93 |
IRMOF-3 |
4 |
Solvent-freec |
3 |
60 |
81 |
IRMOF-1 |
4 |
Solvent-freec |
5 |
60 |
21 |
IRMOF-1 |
4 |
Solvent-freec |
8 |
60 |
27 |
In order to examine the scope of this process, and to demonstrate the diversity of IRMOF-3, the optimized conditions were applied to a series of substrates, as shown in Table 2. The catalytic activity of IRMOF-3 in the three-component synthesis of DHPMs, by the condensation of aldehydes, β-dicarbonyl compounds and urea, was studied and led to the dihydropyrimidinones (3).
Table 2 The IRMOF-3-catalyzed three-component Biginelli couplinga
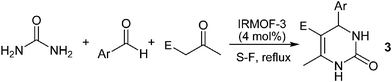
|
3 |
E |
Ar |
Time (h) |
% Yield |
Isolated yields. |
a |
CO2Me |
C6H5 |
5 |
93 |
b |
CO2Et |
C6H5 |
5 |
90 |
c |
CO2Me |
2-Cl–C6H4 |
5 |
88 |
d |
CO2Et |
2-Cl–C6H4 |
5 |
87 |
e |
CO2Me |
3-Cl–C6H4 |
4.5 |
90 |
f |
CO2Et |
3-Cl–C6H4 |
4.5 |
86 |
g |
CO2Me |
4-Cl–C6H4 |
4 |
92 |
h |
CO2Et |
4-Cl–C6H4 |
4 |
94 |
i |
CO2Me |
4-NO2–C6H4 |
4 |
94 |
j |
CO2Et |
4-NO2–C6H4 |
4 |
95 |
It is noteworthy that dihydropyridines manifest a number of important and therapeutically useful biological activities.10 DHPs have received significant attention in organic synthesis, and various methods have been developed for their synthesis. Here, we explored the effectiveness of the MOF nanoreactor catalytic system as an ultra-fast, waste-free and reusable method for the generation of DHPs 4 via multicomponent coupling (Table 3).
Table 3 IRMOF-3-catalyzed Hantzsch condensationa
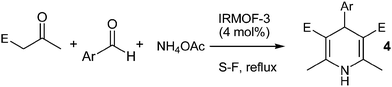
|
4 |
E |
Ar |
Time (h) |
%Yield |
Isolated yields. |
a |
CO2Me |
C6H5 |
5 |
90 |
b |
CO2Me |
2-OMe–C6H4 |
6 |
79 |
c |
CO2Me |
3-OMe–C6H4 |
6 |
76 |
d |
CO2Me |
4-Cl–C6H4 |
4 |
93 |
e |
CO2Me |
4-NO2–C6H4 |
4 |
94 |
f |
CO2Et |
C6H5 |
5 |
89 |
g |
CO2Et |
2-Cl–C6H4 |
4 |
86 |
h |
CO2Et |
3-Cl–C6H4 |
5 |
90 |
i |
CO2Et |
4-Cl–C6H4 |
4 |
92 |
j |
CO2Et |
4-NO2–C6H4 |
4 |
94 |
The possibility of recycling and reusing the catalyst was then examined for model compound 3a. In this case, after completion of the reactions (TLC), hot ethanol or CH2Cl2 was added and the catalyst was filtered and reused four times (Fig. 7).
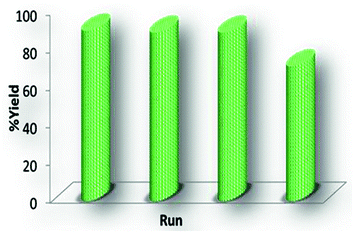 |
| Fig. 7 Recyclability of IRMOF-3 in the synthesis of 3a. | |
To investigate the size selectivity of IRMOF-3, the Biginelli reaction was performed using an equimolecular mixture of benzaldehyde (1 mmol) and p-nitrobenzaldehyde (1 mmol) in the same reaction conditions as described in the Experimental section. After 30 min of reaction, the yield of 3b was 65%, whereas the yield of 3j was 27% (Scheme 1). This indicates that there is some amount of reactivity that occurs outside of the framework, or via defects present in the crystal domains which facilitates guest diffusion.
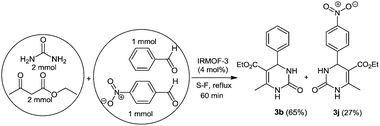 |
| Scheme 1 Test for the size selectivity of IRMOF-3. | |
Conclusions
In summary, we reported the catalytic application of the porous IRMOF-3 nanomaterials for the multicomponent coupling of aldehydes, β-diketones and urea or ammonia to produce DHPMs and DHPs of pharmacological and biological interest in the Biginelli and Hantzsch coupling reactions, in high yields under solvent-free conditions. This method has many advantages such as short reaction times, small amount of catalyst used and facile recyclability, and especially the lack of toxic organic solvents in the reaction, which provides a green and effective method for the synthesis of DHPMs and DHPs.
Notes and references
- H. Li, M. Eddaoudi, M. O'Keeffe and O. Yaghi, Nature, 1999, 402, 276 CrossRef CAS PubMed.
-
(a) J. L. C. Rowsell and O. M. Yaghi, Microporous Mesoporous Mater., 2004, 73, 3 CrossRef CAS PubMed;
(b) F. X. L. I. Xamena, A. Abad, A. Corma and H. Garcia, J. Catal., 2007, 250, 294 CrossRef PubMed.
- M. Savonnet, S. Aguado, U. Ravon, D. Bazer-Bachi, V. Lecocq, N. Bats, C. Pinel and D. Farrusseng, Green Chem., 2009, 11, 1729 RSC.
-
(a) N. T. S. Phan, K. K. A. Le and T. D. Phan, Appl. Catal., A, 2010, 382, 246 CrossRef CAS PubMed;
(b) S. Gao, N. Zhao, M. Shu and S. Che, Appl. Catal., A, 2010, 388, 196 CrossRef CAS PubMed.
- F. Song, C. Wang, J. M. Falkowski, L. Ma and W. Lin, J. Am. Chem. Soc., 2010, 132, 15390 CrossRef CAS PubMed.
- T. Dewa, T. Saiki and Y. Aoyama, J. Am. Chem. Soc., 2001, 123, 502 CrossRef CAS.
- S. Rostamnia, H. Xin and N. Nouruzi, Microporous Mesoporous Mater., 2013, 179, 99 CrossRef CAS PubMed.
-
(a) J. Gascon, U. Aktay, M. D. Hernandez-Alonso, G. P. M. van Klink and F. Kapteijn, J. Catal., 2009, 261, 75 CrossRef CAS PubMed;
(b) P. Serra-Crespo, E. V. Ramos-Fernandez, J. Gascon and F. Kapteijn, Chem. Mater., 2011, 23, 2565 CrossRef CAS;
(c) M. D-Lange, J. Gutierrez-Sevillano, S. Hamad, T. Vlugt, S. Calero, J. Gascon and F. Kapteijn, J. Phys. Chem. C, 2013, 117, 7613 CrossRef;
(d) P. Serra-Crespo, E. Gobechiya, E. V. Ramos-Fernandez, J. Juan-Alcañiz, A. Martinez-Joaristi, E. Stavitski, C. Kirschhock, J. Martens, F. Kapteijn and J. Gascon, Langmuir, 2012, 28, 12916 CrossRef CAS PubMed.
- F. X. L. Xamena, F. G. Cirujano and A. Corma, Microporous Mesoporous Mater., 2012, 157, 112 CrossRef PubMed.
-
(a) A. Alizadeh and S. Rostamnia, Synthesis, 2010, 4057 CrossRef CAS PubMed;
(b) K. S. Atwal, B. N. Swanson, S. E. Unger, D. M. Floyd, S. Moreland, A. Hedberg and B. C. Reilly, J. Med. Chem., 1991, 34, 806 CrossRef CAS;
(c) C. O. Kappe, Eur. J. Med. Chem., 2000, 35, 1043 CrossRef CAS;
(d) R. A. Janis and D. J. Triggle, J. Med. Chem., 1983, 26, 775 CrossRef CAS.
-
(a) S. Rostamnia and A. Hassankhani, RSC Adv., 2013, 3, 18626 RSC;
(b) S. Rostamnia, K. Lamei, M. Mohammadquli, M. Sheykhan and A. Heydari, Tetrahedron Lett., 2012, 53, 5257 CrossRef CAS PubMed;
(c) S. Rostamnia and K. Lamei, Synthesis, 2011, 3080 CrossRef CAS PubMed;
(d) S. Rostamnia and A. Zabardasti, J. Fluorine Chem., 2012, 144, 69 CrossRef CAS PubMed;
(e) S. Rostamnia, E. Doustkhah and A. Nuri, J. Fluorine Chem., 2013, 153, 1 CAS;
(f) S. Rostamnia, A. Nuri, H. Xin, A. Pourjavadi and S. H. Hosseini, Tetrahedron Lett., 2013, 54, 3344 CrossRef CAS PubMed;
(g) S. Rostamnia, H. Xin, X. Liu and K. Lamei, J. Mol. Catal. A: Chem., 2013, 85, 374–375 Search PubMed;
(h) S. Rostamnia and H. Xin, Appl. Organomet. Chem., 2013, 27, 348 CrossRef CAS.
- P. Li, S. Regati, R. J. Butcher, H. D. Arman, Z. Chen, S. Xiang, B. Chen and C. G. Zhao, Tetrahedron Lett., 2011, 52, 6220 CrossRef CAS PubMed.
Footnote |
† Electronic supplementary information (ESI) available. See DOI: 10.1039/c3ra46709k |
|
This journal is © The Royal Society of Chemistry 2014 |
Click here to see how this site uses Cookies. View our privacy policy here.