DOI:
10.1039/C3RA45921G
(Paper)
RSC Adv., 2014,
4, 1-7
SO3H-functionalized Brønsted acidic ionic liquids as efficient catalysts for the synthesis of isoamyl salicylate†
Received
18th October 2013
, Accepted 5th November 2013
First published on 5th November 2013
Abstract
Six Brønsted acidic ionic liquids (BAILs) composed of [HSO4] were prepared, characterized, and used as catalysts of low dosage in the synthesis of isoamyl salicylate. The effects of various parameters such as the kind of BAILs, temperature, catalyst loading, and molar ratio of the reactants on the conversion of salicylic acid were also examined in detail. The results suggested that the catalytic performances of BAILs were of close relevance to their Hammett acidities. The SO3H-functionalized BAILs 1-(4-sulfonic acid) butyl-3-methylimidazolium hydrogen sulfate ([BSmim][HSO4]) and N-(4-sulfonic acid) butyl triethylammonium hydrogen sulfate ([BSEt3N][HSO4]) of strong acidities exhibited excellently catalytic activities and selectivities in the esterification of salicylic acid with isoamyl alcohol. The fully optimized geometries of [BSmim][HSO4] and [BSEt3N][HSO4] further manifest that their strong acidities are derived from the strong interactions between the anion with the sulfonic acid group. In addition, it was found that [BSmim][HSO4] could be also recovered easily and used repetitively at least six times without obvious decline in activity and quantity.
Introduction
Isoamyl salicylate is an important intermediate and fine chemicals in the fields of food, pharmaceuticals, flavors, and perfumes, owing to its distinctive fragrance of clover.1 At present, isoamyl salicylate is mostly synthesized by using concentrated sulfuric acid as homogenous catalyst through the esterification reaction of salicylic acid with isoamyl alcohol in the spice industry. However, salicylic acid with the structure of hydroxyl and carboxyl groups was easy to oxidize because of the strong oxidization of concentrated sulfuric acid, which led to increase the side-effects and decrease the esterification rate. Besides, conventional mineral acids would cause severe equipment corrosion and environmental pollution. Although solid acid catalysts such as mesoporous materials,2,3 metal oxides,4,5 and zeolites6,7 overcame some shortcomings compared with traditional concentrated sulfuric acid in a certain extent, there were also other defects such as relatively low activity, high mass transfer resistance, uneven acid strength distribution, easy carbon deposition on the surface and so on, which all limited their industrial applications in the synthesis of isoamyl salicylate. Therefore, there is an urgent need to develop environmentally friendly, high efficient and easily reusable catalysts for the isoamyl salicylate synthesis process.
In recent years, ionic liquids (ILs) are regarded as new type of green efficient catalyst and reaction media. They present a broad application prospect and have been successfully introduced into the field of catalysis owing to their unique chemical properties such as negligible vapor pressure, excellent thermal stability, remarkable solubility, and effortless reusability.8–10 Till now, ILs has been extensively used in alkylation,11 esterification,12 acetalization,13 ketalization,14 nitration,15 and so on, which obviously exhibits high catalytic activity and selectivity. Thus, acid-catalyzed esterification reactions based on ILs have received more and more attention.16–20 In 2001, Deng et al.12 reported their pioneering work on the esterification of carboxylic acids with alcohols in the presence of acidic chloroaluminate ILs as catalyst. The catalyst ILs can be recycled and reused by decanting simply the produced ester that existed mainly in the upper phase of the resulting mixture. After that, Cole et al.16 had firstly synthesized SO3H-functionalized Brønsted acidic ionic liquids (BAILs) and used them for the esterification of acetic acid with ethanol. They found that these BAILs showed a good catalytic performance and reusability in the esterification reactions. Yu et al.18 had further illustrated the merits of using SO3H-functionalized BAILs for the synthesis of plasticizer ester. Li et al.19 reported the synthesis of methyl salicylate in BAILs under microwave irradiation. Despite the researches on BAILs used in the esterification reaction had been reported a lot, the study on synthesis of isoamyl salicylate using BAILs as catalysts has not been seen. In addition, despite the excellent yields, the microwave-accelerated strategy often formed side products (such as phenol) and decreased the selectivity of methyl salicylate.19 Herein, we believe that further investigation is needed to explore the synthesis of isoamyl salicylate in BAILs with high activity and selectivity.
Therefore, several BAILs had been prepared and their catalytic activities for the synthesis of isoamyl salicylate were investigated in this present work. The reaction parameters such as kind of BAILs, temperature, catalyst loading, and molar ratio of reactants were explored in detail to obtain the optimum conditions. For comparison, the catalytic activities of the two conventional heterogeneous catalysts (phosphorictungs acid and Amberlyst-15 resin) in the synthesis of isoamyl salicylate were also carried out. Additionally, the Hammett acidity and reusability of BAILs was studied, and their acidity–catalytic activity relationships were further discussed.
Experimental
Materials
1-Methylimidazole (purity ≥99%), and 1,4-butyl sultone (purity ≥99%) were purchased from Aladdin Chemical Reagent Co. Ltd. (Shanghai, China). The ion-exchange resin Amberlyst-15 was obtained from Rohm and Haas. Other reagents such as salicylic acid, ethanol, n-propanol, n-butanol, n-pentanol, isoamyl alcohol, n-hexanol, sulfuric acid, phosphorictungs acid, triethylamine, 1-bromobutane, sodium hydrogen sulfate, and acetone were of analytical grade and used without any further purification.
Preparation and characterization of BAILs
Six BAILs composed of [HSO4] anion and different cations (Fig. 1) were synthesized by a two-step method, which is similar to the procedure described in our previous work and other ref. 21–25. The detailed preparation procedure and 1H NMR spectrum (Bruker DPX-300) of these BAILs were as follows:
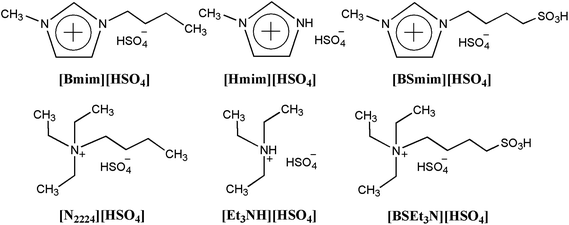 |
| Fig. 1 Structure of six Brønsted acidic ionic liquids. | |
1-(4-Sulfonic acid) butyl-3-methylimidazolium hydrogen sulfate ([BSmim][HSO4]): the preparation of this BAIL was similar to those used in the previous literature.21 1-Methylimidazole (0.1 mol) and 1,4-butane sultone (0.1 mol) were charged into a 100 mL round-bottom flask. Then, the mixtures were stirred at 80 °C for 12 h. The white solid zwitterion was washed repeatedly with ether (2 × 5 mL) to remove any unreacted starting materials and dried in vacuum. Then, a stoichiometric amount of concentrated sulfuric acid was added and the mixture stirred for six hours at 60 °C during which time the solid zwitterion dissolved/liquefied, resulted in the formation of [BSmim][HSO4]. The BAIL phase was then washed repeatedly with dichloromethane and ether to remove non-ionic residues, and dried in vacuum. Then [BSmim][HSO4] of yellow viscous liquid was obtained with a yield of 90%. 1H NMR (300 MHz, D2O-d2, 25 °C, TMS); δ (ppm) 1.605 (m, 2H), 1.888 (m, 2H), 2.809 (t, 2H), 3.754 (s, 3H), 4.110 (t, 2H), 7.305 (s, 1H), 7.364 (s, 1H), 8.60 (s, 1H).
N-(4-Sulfonic acid) butyl triethylammonium hydrogen sulfate ([BSEt3N][HSO4]): the synthesis of [BSEt3N][HSO4] was carried out according to the procedure described for [BSMim][HSO4] from triethylamine and 1,4-butane sultone. [BSEt3N][HSO4] of colorless viscous liquid was obtained with a yield of 92%. 1H NMR (300 MHz, D2O-d2, 25 °C, TMS): δ 1.122 (t, 9H), 1.679 (m, 4H), 2.825 (t, 2H), 3.08 (t, 2H), 3.178 (q, 6H).
1-Methylimidazole hydrogen sulfate ([Hmim][HSO4]): the preparation method was according to the previous ref. 22 the 1-methylimidazole (0.1 mol) was charged into a 100 mL round-bottom flask in a water bath. Sulfuric acid (0.1 mol) aqueous solution was added slowly into the reaction solution at 0 °C under stirring. The mixture was stirred for 10 h to complete the reaction. The resulting solution was then dried in vacuum at 95 °C to remove the water until the weight of the residue remained constant. The yield of [Hmim][HSO4] was 98%. 1H NMR (300 MHz, D2O-d2, 25 °C, TMS): δ (ppm) 3.563 (s, 3H), 7.083 (s, 2H), 8.307 (s, 1H).
N-Triethylammonium hydrogen sulfate ([Et3NH][HSO4]): the preparation process was similar to the procedure of [Hmim][HSO4].23 The yield of [Et3NH][HSO4] was 96%. 1H NMR (300 MHz, DMSO-d6, 25 °C, TMS): δ (ppm) 1.17 (t, 9H), 3.08 (m, 6H), 8.89 (s, 1H).
1-Butyl-3-methylimidazolium hydrogen sulfate ([Bmim][HSO4]): the synthetic method was in accordance with the previous literature.24 1-Methylimidazole (0.1 mol), 1-bromobutane (0.1 mol) and ethanol (50 mL) were charged into a 100 mL round-bottom flask. Then, the mixtures were vigorous stirring at 80 °C for 6 h. After that, the solvent and unreacted components were removed by rotary evaporation, and [Bmim][Br] (about 20 g) white solid was obtained after being dried at 60 °C under vacuum for 5 h. Then, a stoichiometric amount of sodium hydrogen sulfate was added to the mixture solution of [Bmim][Br] and anhydrous acetone. The metathesis reaction was stirred for 24 h at room temperature and the white solid formed was then filtered off. The volatiles were finally removed in vacuum, and the BAIL [Bmim][HSO4] of light yellow liquid was obtained with a yield of 85%. The concentration of Br− in [Bmim][HSO4] was measured by Mohr titration, and the related impurity was less than 0.02 wt%. 1H NMR (300 MHz, D2O-d2, 25 °C, TMS); δ (ppm) 1.058 (t, 3H), 1.656 (m, 2H), 1.921 (m, 2H), 3.785 (s, 3H), 4.144 (t, 2H), 7.327 (s, 1H), 7.398 (s, 1H), 8.637 (s, 1H).
Butyltriethylammonium hydrogen sulfate ([N2224][HSO4]): the preparation method is referenced to our previous work.25 the synthesis of [N2224][HSO4] was carried out according to the procedure described for [Bmim][HSO4] above from triethylamine and 1-bromobutane. [N2224][HSO4] of colorless viscous liquid was then obtained with a yield of 88%. The concentration of Br− in [N2224][HSO4] was measured by Mohr titration, and the related impurity was less than 0.02 wt%. 1H NMR (300 MHZ, D2O-d2, 25 °C, TMS): δ (ppm) 0. 81 (t, 3H), 1.09 (t, 9H), 1.26 (m, 2H), 1.49 (m, 2H), 3.00 (m, 2H), 3.14 (q, 6H).
UV-vis determination of acidity
The Brønsted acidity is an essential property of BAILs, and Hammett function (H0) is often an effective way to assess the acidity of BAILs in organic solvents, which is calculated by evaluating the protonation extent of indicator base using an Agilent 8453 UV-visible spectroscopy.19,26,27 In our experiment, the Hammett acidity of six BAILs in ethanol solutions (80 mmol L−1) had been investigated at 25 °C, and 4-nitroaniline was used as indicator (0.06 mmol L−1). The maximal absorbance of unprotonated form of 4-nitroaniline was observed at 371 nm, and the pK(I)aq. value of 4-nitroaniline is 0.99. The results are then listed in Table 1, and the acidity order is as follows: [BSmim][HSO4] > [BSEt3N][HSO4] > [Hmim][HSO4] > [Et3NH][HSO4] > [Bmim][HSO4] > [N2224][HSO4].
Table 1 Hammett acidity function (H0) values of H2SO4 and different BAILsa
Entry |
IL |
A
max
|
[I] (%) |
[IH+] (%) |
H
0
|
The Hammett function (H0) is written as: H0 = pK(In)aq. + log([I]/[IH+]), where pK(I)aq. is the pKa value of the indicator referred to an aqueous solution. [I] and [IH+] are the molar concentrations of the unprotonated and protonated forms of the indicator, respectively.
|
1 |
— |
1.03 |
100 |
0 |
— |
2 |
[BSmim][HSO4] |
0.530 |
51.5 |
48.5 |
1.02 |
3 |
[BSEt3N][HSO4] |
0.538 |
52.2 |
47.8 |
1.03 |
4 |
[Hmim][HSO4] |
0.927 |
90 |
10 |
1.94 |
5 |
[Et3NH][HSO4] |
0.963 |
93.5 |
6.5 |
2.15 |
6 |
[Bmim][HSO4] |
0.976 |
94.8 |
5.2 |
2.25 |
7 |
[N2224][HSO4] |
0.990 |
96.1 |
3.9 |
2.38 |
Computational methods
Density functional theory (DFT) was employed to perform the geometry optimizations at the 6-31++G** level using the Gaussian 09 program package.28 Each final optimized structure of BAILs was checked to be a true minimum through frequency calculation at the corresponding levels.
General procedure for the esterification reaction
In a typical run, the esterification reaction was performed in a 25 mL round-bottom flask with a magnetic stirrer. The temperature of the reaction mixture was controlled using a temperature controller with an accuracy of ±0.1 °C. Weighed amounts of salicylic acid, isoamyl alcohol and catalyst (BAILs, phosphotungstic acid or Amberlyst-15 resin) were mixed and typically allowed to proceed for 0.5–2.5 h with the vigorous stirring and heating at the designed temperature. About 0.2 mL of liquid sample was taken out from the reactor at regular intervals, and the composition of products was analyzed by gas chromatography-flame ionization detector (GC-FID) (Agilent 6890, HP-1 column, 30 m × 0.32 mm × 1 μm) using N2 as the carrier gas at a flow rate of about 3 mL min−1. The GC-FID conditions were as follows: detector temperature, 250 °C; injection port temperature, 200 °C; oven temperature, 180 °C. Then the conversion and selectivity were calculated according to the area of chromatograph peak. After the reaction, the esterification system often forms a liquid–liquid biphase. The upper phase, containing mainly the desired ester, can be isolated simply by decantation, whereas the lower phase composed of BAIL and water can be easily recovered and reused after treatment under vacuum to remove water. After that, the as synthesized isoamyl salicylate was characterized by 1H NMR (Bruker DPX-300) and FT-IR (Thermo Nicolet 870): 1H NMR (300 MHz, CDCl3-d, 25 °C, TMS); δ (ppm) 1.01 (d, 6H), 1.67 (q, 2H), 1.80 (m, 1H), 4.38 (t, 2H), 6.87 (t, 1H), 6.96 (d, 1H), 7.44 (t, 1H), 7.82 (d, H), 10.86 (s, 1H). IR ν = 3185, 2960, 2873, 1676, 1614, 1486, 1302, 1089, 756 cm−1. Therefore, it was validated from these characterization results that isoamyl salicylate was successfully synthesized by using BAILs as catalysts.
Results and discussion
Catalyst performance
The esterification reaction of salicylic acid with isoamyl alcohol in the presence of six BAILs composed of various cations was conducted to assess their catalytic activities at the temperature of 130 °C, with salicylic acid to isoamyl alcohol molar ratio of 1
:
2, and catalyst loading of 20 wt% (based on the mass of salicylic acid). The results are then summarized in Table 2. Combined with the Brønsted acidity values from Table 1, it was obvious that these six BAILs catalysts exhibited different catalytic activities, and the catalytic performance of a BAIL was mainly relevance to its Brønsted acidity. The BAIL possessing strong acidity shows the relatively high catalytic activity.27,29 For example, [BSmim][HSO4] and [BSEt3N][HSO4] beared with an butyl sulfonic acid exhibited the strongest acidities among all the BAILs investigated. Thus, the SO3H-functionalized BAILs [BSEt3N][HSO4] and [BSmim][HSO4] catalyzed the esterification reaction to have high conversions of 90.5% and 90.8% at 2 h, respectively. By comparison, in the presence of weak acidic catalyst [Hmim][HSO4] and [Et3NH][HSO4], the conversions of salicylic acid at 2 h were only 25.6% and 20.3%, respectively, being significantly less than those of [BSEt3N][HSO4] and [BSmim][HSO4] under identical conditions. [Bmim][HSO4] and [N2224][HSO4] also induced very low conversions of salicylic acid at 2 h, due to their neutral or weakly acidic cations. Therefore, it was demonstrated that varying the cationic structure of BAIL can change its acidity conveniently, and the strong acidity enables the BAIL to perform excellent catalytic activities in esterification.
Table 2 Results of the esterification reaction of salicylic acid with isoamyl alcohol in the presence of different catalystsa
Entry |
Catalyst |
Conversion of salicylic acid (%) |
Reaction conditions: the esterification reaction was conducted at the temperature of 130 °C, with salicylic acid to isoamyl alcohol molar ratio of 1 : 2, and catalyst dosage of 20 wt% for 2 h. No byproducts were found by GC.
|
1 |
[BSmim][HSO4] |
90.8 |
2 |
[BSEt3N][HSO4] |
90.5 |
3 |
[Hmim][HSO4] |
25.6 |
4 |
[Et3NH][HSO4] |
20.3 |
5 |
[Bmim][HSO4] |
17.4 |
6 |
[N2224][HSO4] |
18.7 |
7 |
Amberlyst-15 |
55.1 |
8 |
Phosphorictungs acid |
87.2 |
9 |
Blank |
1.2 |
In addition, in order to understand the role of cations in the acidities of BAILs, DFT calculations were performed using the Gaussian 09 program. The fully optimized geometries of BAILs were then presented in Fig. S1–S4 in the ESI.† As seen from calculation results, the SO3H-functionalized BAILs [BSmim][HSO4] and [BSEt3N][HSO4] are found to have much stronger intramolecular hydrogen bonds than [Hmim][HSO4] and [Et3NH][HSO4]. For example, the strong interactions between the anion with the sulfonic acid proton exhibit in [BSmim][HSO4], with H24⋯O23 distance of 1.607 Å and H35⋯O25 distance of 1.638 Å (Fig. S1†). As such, the O⋯H distances of hydrogen bonds H37⋯O35 and H45⋯O38 in [BSEt3N][HSO4] are 1.722 Å and 1.652 Å, respectively (Fig. S2†). Due to these strong interactions, the H–O bond of the sulfonic acid group has been lengthened and thus the SO3H-functionalized BAILs are inclined to exist in a form of the zwitterion and H2SO4.30 As a result, the SO3H-functionalized BAILs containing two adjacent acid sites show strong acidities as well as H2SO4 does, and then they catalyze the esterification reaction to have the considerable conversions.
For comparison, the esterification reaction without catalyst or in the presence of Amberlyst-15 resin and phosphorictungs acid was also investigated. As seen from Table 2, it was indicated that the conversion of salicylic acid at 2 h in the blank reaction was less than 5%, showing that the esterification reaction is very difficult to occur without catalyst. In addition, it was found that the conversion of salicylic acid in the presence of Amberlyst-15 resin was lower than that in the cases of [BSEt3N][HSO4] and [BSmim][HSO4], whereas phosphorictungs acid showed the catalytic activity almost as high as those two SO3H-functionalized BAILs. This is because that phosphorictungs acid can be dissolved in water or alcohol and act as homogeneous catalyst in the esterification reaction. Nevertheless, the heterogeneous catalysis of Amberlyst-15 resin existed high transfer resistance and resulted in relative lower catalytic performance. Thus, [BSEt3N][HSO4] and [BSmim][HSO4] are considered to be promising substitutes in the synthesis of isoamyl salicylate, and then they were chosen as the catalysts for the following factor experiments.
Optimization of reaction conditions
The investigation on the effect of temperature is very important because this information is essential to the synthesis of isoamyl salicylate. Thus, the esterification reaction of salicylic acid was performed using [BSEt3N][HSO4] and [BSmim][HSO4] as catalysts at 110 °C, 120 °C, 130 °C and 140 °C, with salicylic acid to isoamyl alcohol molar ratio of 1
:
2, and catalyst loading of 20 wt%. As seen from Fig. 2, the conversion of salicylic acid increased rapidly with the increase in the reaction temperature. For [BSmim][HSO4], the conversion of salicylic acid at 2 h increased obviously from 71% to 90% with the rise of temperature from 110 °C to 130 °C. This means that high temperature is facilitated to improve the conversion of salicylic acid. However, while the temperature was further increased to 140 °C, the conversion of salicylic acid at 2 h was improved with a limited extent, due to the limitation of chemical reaction equilibrium. This phenomenon was also similarly observed in the esterification system containing [BSEt3N][HSO4] catalyst. In addition, side product (such as phenol) would be probably produced, whilst the temperature was higher than 150 °C.2,6 This illustrated that the over high reaction temperature was negative to the conversion of salicylic acid and it was unnecessary for the esterification reaction. Therefore, an optimized reaction temperature would choose at 130 °C for the SO3H-functionalized BAILs to reach the best catalytic activity.
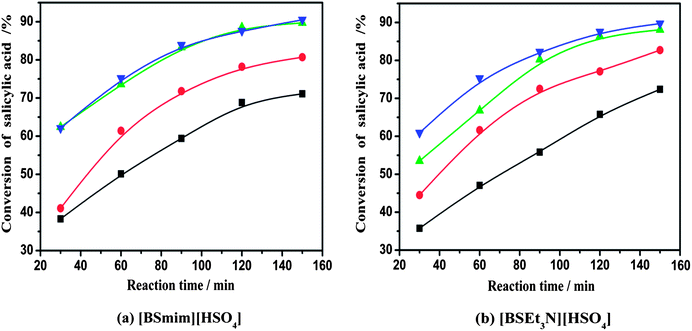 |
| Fig. 2 Effect of temperature on the conversion of salicylic acid with time using (a) [BSmim][HSO4] and (b) [BSEt3N][HSO4] as catalysts at (■) 110 °C; (●) 120 °C; (▲) 130 °C; (▼) 140 °C, with salicylic acid to isoamyl alcohol molar ratio of 1 : 2, and catalyst loading of 20 wt%. | |
The catalyst loading was expressed as the weight ratio of the catalyst to the weight of salicylic acid. Catalyst loading was varied from 10 wt% to 25 wt% at a temperature of 130 °C and with salicylic acid to isoamyl alcohol molar ratio of 1
:
2. The effect of different catalyst loadings on the conversion of salicylic acid was then given in Table 3. It was demonstrated that, with an increase in the relative amount of BAILs, the total number of acid sites available for the reaction increased and thus the rate of esterification reaction was enhanced, resulting in a higher conversion of salicylic acid. For example, the conversion of salicylic acid increased from 77.4% to 90.8% with the increase in the amount of [BSmim][HSO4] from 10 wt% to 20 wt%. However, only a fair change was obtained in the conversion of salicylic acid when the amount of [BSmim][HSO4] catalyst was further added to 25 wt%, 30 wt%, and 35 wt%, respectively. Beyond a certain catalyst loading, the conversion of salicylic acid has not a large degree of improvement. Therefore, it suggests that too much dosage of BAILs is not very necessary for the synthesis of isoamyl salicylate. In this work, considering the reaction rate and the cost of BAILs, 20 wt% was taken as the optimum catalyst loading and used in most of the esterification experiments.
Table 3 Effect of catalyst loading on the conversion of salicylic acida
Entry |
Catalyst loading |
Conversion of salicylic acid (%) |
[BSmim][HSO4] |
[BSEt3N][HSO4] |
Reaction conditions: the esterification reaction was conducted at the temperature of 130 °C with salicylic acid to isoamyl alcohol molar ratio of 1 : 2 for 2 h. No byproducts were found by GC.
|
1 |
10 wt% |
77.4 |
79.1 |
2 |
15 wt% |
85.1 |
86.9 |
3 |
20 wt% |
90.8 |
90.5 |
4 |
25 wt% |
91.7 |
91.3 |
5 |
30 wt% |
91.8 |
91.5 |
6 |
35 wt% |
91.4 |
91.1 |
Fig. 3 represents the effect of salicylic acid to isoamyl alcohol molar ratio on the esterification reaction in the presence of [BSmim][HSO4] as catalyst. It was illustrated that as the amount of isoamyl alcohol increased, the conversion of salicylic acid was rapidly improved. For example, the conversion was obviously increased from 76% to 91% in 2 h, while the initial molar ratio of salicylic acid to isoamyl alcohol increased from 1
:
1 to 1
:
2. Higher conversions were obtained equally with the molar ratio of 1
:
3 and 1
:
4 in comparison to the case of 1
:
1. Thus, it was demonstrated that increasing the amount of isoamyl alcohol was beneficial to the forward reaction since the esterification of salicylic acid with isoamyl alcohol is a reversible reaction. In addition, it was also found that the reaction rates in the initial acid to alcohol ratios of 1
:
3 and 1
:
4 were just comparable to the case of 1
:
2, indicating that a further increase in the amount of isoamyl alcohol can not continue to improve the conversion of salicylic acid. This means that an initial excess of isoamyl alcohol is not necessary for the esterification of salicylic acid. In summary, the optimal molar ratio of salicylic acid to isoamyl alcohol was preferred to be 1
:
2.
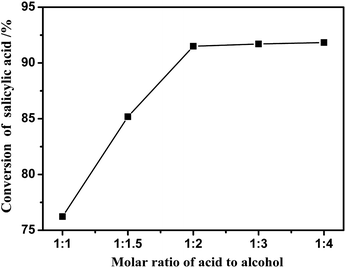 |
| Fig. 3 Effect of molar ratio on the conversion of salicylic acid in the presence of [BSmim][HSO4] at the temperature of 130 °C with catalyst loading of 20 wt% for 2 h. | |
Esterification of salicylic acid with other alcohols
Using the above-mentioned optimized conditions, we had further investigated the esterification of salicylic acid with other alcohols in the presence of the BAILs [BSmim][HSO4] and [Bmim][HSO4] as catalysts, and the experimental results are given in Table 4. It was showed that [Bmim][HSO4] was of very poor activity in these other esterification reactions, whereas [BSmim][HSO4] performed considerable conversions owing to its strong acidity. For example, the conversions of salicylic acid in the esterification systems containing n-butanol, n-pentanol, and n-hexanol were 89.5%, 89.6%, and 80.6%, respectively. In addition, it was also found that the conversion of salicylic acid increases as the carbon chain of alcohol prolongs (entries 1–6). This was because the change in the formation of liquid–liquid biphases, which was pointed out in our previous work.25 For example, in the cases of ethanol, iso-propanol, and n-propanol, both of them have better solubility in the mixture of reactants and products owing to their good hydrophilicity. These esterification systems then are difficult to form phase splitting, leading to the decrease in the reaction rates and thus the conversions. By contrast, the alcohols n-butanol, n-pentanol, and n-hexanol have relatively long carbon chain and are partly immiscible in the BAIL catalyst and water. Thus, their esterification systems could induce fast phase splitting. The product of ester forms a light phase, while water and BAIL are combined to be a heavy phase. Subsequently, the formation of liquid–liquid biphases facilitates the shift of chemical equilibrium to the product side and thus high conversions.25,31
Table 4 Results of the esterification reaction of salicylic acid with various alcoholsa
Entry |
Alcohol |
Conversion of salicylic acid (%) |
[BSmim][HSO4] |
[Bmim][HSO4] |
Reaction conditions: [BSmim][HSO4] and [Bmim][HSO4] as catalysts with a dosage of 20 wt% at 130 °C for 2 h, molar ratio of salicylic acid to alcohol = 1 : 2. No byproducts were found by GC.
|
1 |
Isopropyl alcohol |
51.1 |
1.2 |
2 |
Ethanol |
63.4 |
4.3 |
3 |
n-Propanol |
73.5 |
6.8 |
4 |
n-Butanol |
89.5 |
10.1 |
5 |
n-Pentanol |
89.6 |
11.6 |
6 |
n-Hexanol |
80.6 |
6.5 |
Catalyst recycling test
The recovery and reuse of catalysts is highly preferable for a greener chemical process. Thus, the reusability of the BAIL [BSmim][HSO4] catalyst was investigated in the esterification reaction of salicylic acid with isoamyl alcohol (temperatures of 130 °C, initial molar ratio of 1
:
2, catalyst loading of 20% w/w, and reaction time of 0.5 h and 2 h). In each cycle, the esterification system often forms a liquid–liquid biphase at the end of the reaction. The lower phase composed of BAIL and water can be easily separated from the reaction mixture by simple decantation. Then the catalyst BAIL could be recovered and reused in the next run after removing the water under vacuum at 95 °C for 12 h. The conversions of salicylic acid from six consecutive runs thus are as shown in Fig. 4. The experimental results implied that the BAIL [BSmim][HSO4] exhibited nearly no obvious change in activity and 88.3% conversion was maintained after six recycle, which shows that the BAIL catalyst is stable enough and the presence of water produced in the esterification reaction has less impact on the catalytic activity of [BSmim][HSO4]. The slight decrease in the activity may be ascribed to the slight loss of [BSmim][HSO4] due to the transferring of samples during the regeneration.
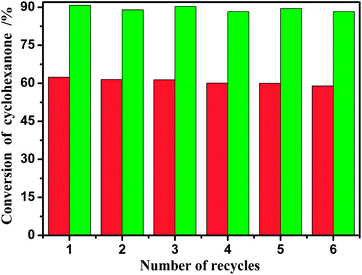 |
| Fig. 4 The recycle test of [BSmim][HSO4] catalyst (red column for 0.5 h and green column for 2 h). | |
Conclusions
Six BAILs composed of [HSO4] had been prepared successfully and applied in the synthesis of isoamyl salicylate in this study. The experimental results demonstrated that the SO3H-functionalized BAILs [BSmim][HSO4] and [BSEt3N][HSO4] exhibited extremely strong acidities and played excellently catalytic activities and selectivities in the esterification of salicylic acid with isoamyl alcohol and other alcohols such as n-butanol, n-pentanol, and n-hexanol. The fully optimized geometries of [BSmim][HSO4] and [BSEt3N][HSO4] also manifest that their strong acidities are derived from the strong interactions between the anion with the sulfonic acid group. In addition, it was found that increasing the reaction temperature, catalyst loading and the amount of isoamyl alcohol could improve the conversion of salicylic acid effectively, and the optimal condition was set as follows: temperature of 130 °C, initial molar ratio of 1
:
2, and catalyst loading of 20% w/w. [BSmim][HSO4] also could be recovered easily and used repetitively at least six times without significant losses of activity and quantity. Therefore, the catalyst SO3H-functionalized BAILs prepared in this study was proved to be an excellent recyclable homogeneous catalyst for the synthesis of isoamyl salicylate and showed potential application in industry.
Acknowledgements
This work was supported by the National Natural Science Foundations of China (no. 21206063), the National High Technology Research and Development Program of China (no. 2012AA03A609), and the Science & Technology Supporting Programs and the International Technological Cooperation Programms of Jiangxi Provincial Department of Science and Technology (no. 20123BBE50081 and 20132BDH80003).
Notes and references
-
R. C. Larock, Comprehensive Organic Transformations, 1999, pp. 1932–1940 Search PubMed.
- Y. Zheng, J. Li, N. Zhao, W. Wei and Y. Sun, Microporous Mesoporous Mater., 2006, 92, 195–200 CrossRef CAS.
- X. Su, J. Li, F. Xiao, W. Wei and Y. Sun, Ind. Eng. Chem. Res., 2009, 48, 3685–3691 CrossRef CAS.
- J. D' Souza and N. Nagaraju, Indian J. Chem. Technol., 2007, 14, 292–300 Search PubMed.
- T. Jiang, Q. Zhao, M. Li and H. Yin, J. Hazard. Mater., 2008, 159, 204–209 CrossRef CAS PubMed.
- S. R. Kirumakki, N. Nagaraju, K. Murthy and S. Narayanan, Appl. Catal., A, 2002, 226, 175–182 CrossRef CAS.
- S. R. Kirumakki, N. Nagaraju, K. V. R. Chary and S. Narayanan, Appl. Catal., A, 2003, 248, 161–167 CrossRef CAS.
- H. Olivier-Bourbigou, L. Magna and D. Morvan, Appl. Catal., A, 2010, 373, 1–56 CrossRef CAS.
- J. P. Hallett and T. Welton, Chem. Rev., 2011, 111, 3508–3576 CrossRef CAS PubMed.
- Q. Zhang, S. Zhang and Y. Deng, Green Chem., 2011, 13, 2619–2637 RSC.
- X. Nie, X. Liu, L. Gao, M. Liu, C. Song and X. Guo, Ind. Eng. Chem. Res., 2010, 49, 8157–8163 CrossRef CAS.
- Y. Q. Deng, F. Shi, J. J. Beng and K. Qiao, J. Mol. Catal. A: Chem., 2001, 165, 33–36 CrossRef CAS.
- D. Li, F. Shi, J. Peng, S. Guo and Y. Q. Deng, J. Org. Chem., 2004, 69, 3582–3585 CrossRef CAS PubMed.
- D. J. Tao, Z. M. Li, Z. Cheng, N. Hu and X. S. Chen, Ind. Eng. Chem. Res., 2012, 51, 16263–16269 CrossRef CAS.
- K. Smith, S. Liu and G. A. El-Hiti, Ind. Eng. Chem. Res., 2005, 44, 8611–8615 CrossRef CAS.
- A. C. Cole, J. L. Jensen, I. Ntai, K. L. T. Tran, K. J. Weave, D. C. Forbes and J. H. Davis, J. Am. Chem. Soc., 2002, 124, 5962–5963 CrossRef CAS PubMed.
- H. Xing, T. Wang, Z. Zhou and Y. Dai, Ind. Eng. Chem. Res., 2005, 44, 4147–4150 CrossRef CAS.
- C. Xie, H. Li, L. Li, S. Yu and F. J. Liu, J. Hazard. Mater., 2008, 151, 847–850 CrossRef CAS PubMed.
- H. Shi, W. Zhu, H. Li, H. Liu, M. Zhang, Y. Yan and Z. Wang, Catal. Commun., 2010, 11, 588–591 CrossRef CAS.
-
(a) D. A. Kotadia and S. S. Soni, Catal. Sci. Technol., 2013, 3, 469–474 RSC;
(b) M. Vafaeezadeh, Z. B. Dizicheh and M. M. Hashemi, Catal. Commun., 2013, 41, 96–100 CrossRef CAS;
(c) F. Han, L. Yang, Z. Li and C. Xia, Adv. Synth. Catal., 2012, 354, 1052–1060 CrossRef CAS;
(d) D. A. Kotadia and S. S. Soni, J. Mol. Catal. A: Chem., 2012, 353–354 Search PubMed , 44–49.
- J. Gui, X. Cong, D. Liu, X. Zhang, Z. Hu and Z. Sun, Catal. Commun., 2004, 5, 473–477 CrossRef CAS.
- A. R. Hajipour, L. Khazdooz and A. E. Ruoho, Catal. Commun., 2008, 9, 89–96 CrossRef CAS.
- C. Wang, L. Guo, H. Li, Y. Wang, J. Weng and L. Wu, Green Chem., 2006, 8, 603–607 RSC.
- V. Singh, S. Kaur, V. Sapehiyia, J. Singh and G. L. Kad, Catal. Commun., 2005, 6, 57–60 CrossRef CAS.
- D. Tao, X. Lu, J. Lu, K. Huang, Z. Zhou and Y. Wu, Chem. Eng. J., 2011, 171, 1333–1339 CrossRef CAS.
- C. Thomazeau, H. O. Bourbigou, L. Magna, S. Luts and B. Gilbert, J. Am. Chem. Soc., 2003, 125, 5264–5265 CrossRef CAS PubMed.
- H. Xing, T. Wang, Z. Zhou and Y. Dai, J. Mol. Catal. A: Chem., 2007, 264, 53–59 CrossRef CAS.
-
M. J. Frisch, G. W. Trucks, H. B. Schlegel, G. E. Scuseria, M. A. Robb, J. R. Cheeseman, G. Scalmani, V. Barone, B. Mennucci, G. A. Petersson, H. Nakatsuji, M. Caricato, X. Li, H. P. Hratchian, A. F. Izmaylov, J. Bloino, G. Zheng, J. L. Sonnenberg, M. Hada, M. Ehara, K. Toyota, R. Fukuda, J. Hasegawa, M. Ishida, T. Nakajima, Y. Honda, O. Kitao, H. Nakai, T. Vreven, J. A. Montgomery, J. E. Peralta, Jr, F. Ogliaro, M. Bearpark, J. J. Heyd, E. Brothers, K. N. Kudin, V. N. Staroverov, R. Kobayashi, J. Normand, K. Raghavachari, A. Rendell, J. C. Burant, S. S. Iyengar, J. Tomasi, M. Cossi, N. Rega, J. M. Millam, M. Klene, J. E. Knox, J. B. Cross, V. Bakken, C. Adamo, J. Jaramillo, R. Gomperts, R. E. Stratmann, O. Yazyev, A. J. Austin, R. Cammi, C. Pomelli, J. W. Ochterski, R. L. Martin, K. Morokuma, V. G. Zakrzewski, G. A. Voth, P. Salvador, J. J. Dannenberg, S. Dapprich, A. D. Daniels, O. Farkas, J. B. Foresman, J. V. Ortiz, J. Cioslowski and D. J. Fox, GAUSSIAN09 (Revision. C.01), Gaussian, Inc., Wallingford, CT, 2009 Search PubMed.
- Y. Zhao, J. Long, F. Deng, X. Liu, Z. Li, C. Xia and J. Peng, Catal. Commun., 2009, 10, 732–736 CrossRef CAS.
- X. Liu, Z. Song and H. Wang, Struct. Chem., 2009, 20, 509–515 CrossRef CAS.
- H. Zhou, J. Yang, L. Ye, H. Lin and Y. Yuan, Green Chem., 2010, 12, 661–665 RSC.
Footnote |
† Electronic supplementary information (ESI) available: Details on the fully optimized geometries structures and molecular coordinates of BAILs. See DOI: 10.1039/c3ra45921g |
|
This journal is © The Royal Society of Chemistry 2014 |