DOI:
10.1039/C3RA44828B
(Paper)
RSC Adv., 2014,
4, 358-364
Catalyst-free synthesis of benzofuran-fused pyrido[4,3-d]pyrimidines from 2-(2-hydroxyaryl)acetonitrile and 4,6-dichloropyrimidine-5-carbaldehyde through domino condensation reactions†
Received
2nd September 2013
, Accepted 6th November 2013
First published on
7th November 2013
Abstract
A rapid, one-pot, catalyst-free approach to novel benzofuran-fused pyrido[4,3-d]pyrimidines with good antitumor activities via a cascade SNAr/cyclization/condensation reaction through 2-(2-hydroxyphenyl)acetonitriles and 4,6-dichloropyrimidine-5-carbaldehyde was developed.
Introduction
The pursuit of large diversified compound libraries in drug discovery and other organic synthesis continues to stimulate the design and development of new concepts and innovative synthetic strategies. The use of cascade reactions is one of the most efficient strategies for rapid generation of complex molecules from simple starting materials and multiple-step reactions are occurred in on pot.1 What is more, it is easier to operate with only one single reaction solvent, workup procedure, and purification step than traditional synthetic methods with several individual steps.2 In addition, it is well accepted that cascade reactions were associated with economies of time, labour, cost, and waste generation, which is advantageous from the viewpoint of green chemistry and sustainability.3 Therefore, cascade reactions are ideal synthetic tools to address the demand of constructing diverse heterocycles with high efficiency and environmentally benign reaction conditions.
Heterocyclic rings are of remarkable biological and chemical significance in many fields. Benzofurans or fused benzofurans are ubiquitous in compounds displaying interesting biological properties such as antifungal agents4 and inhibitors of tubulin polymerization,5 hepatitis C virus RNA-dependent RNA polymerase6 and mycobacterium protein tyrosine phosphatase B (mPTPB).7 Moreover, fused-polycyclic compounds also occur in many natural products and biologically active molecules with potent bioactivities (Fig. 1).8 Thus a simple and facile synthetic method for generation of these heterocyclic molecules is highly desirable.
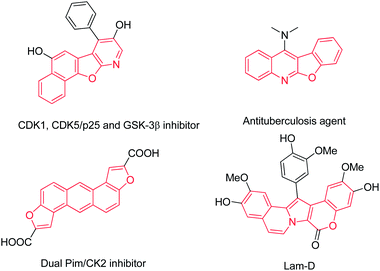 |
| Fig. 1 Fused-polycyclic compounds in natural and medicinal compounds. | |
Over the last few decades, both (2-hydroxyphenyl)acetonitrile9 and 4,6-dichloropyrimidine-5-carboxaldehyde10 have been widely applied in drug discovery owing to their potential for the synthesis of various bioactive compounds as precursors, especially (2-hydroxyphenyl)acetonitrile and related compounds have been used to construct bioactive 2-aminobenzofurans.9a,11
Recently, our group and others have reported a similar method for preparation of dibenzo[b,f]oxepins and their analogues via a one-pot cascade reaction through 2-(2-hydroxyphenyl)acetonitriles and 2-bromobenzaldehydes (Scheme 1).9f,i Herein, we treated 4,6-dichloropyrimidine-5-carboxaldehyde with 2-(2-hydroxyphenyl)acetonitrile under similar conditions. Much to our surprise, 2-aminobenzofuran-containing tetracyclic product B, determined by X-ray crystallography (Fig. 2), was obtained but not dibenzo[b,f]oxepin product A, even though only one equivalent 2-(2-hydroxyphenyl)acetonitrile was used. The reaction might go through a cascade SNAr/cyclization/condensation reaction pathway (scheme 1, path B) rather than a sequential aldol condensation and intramolecular ether formation reaction pathway (scheme 1, path A).
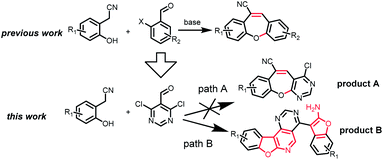 |
| Scheme 1 Established methods for synthesis of novel fused-polycyclic heterocycles. | |
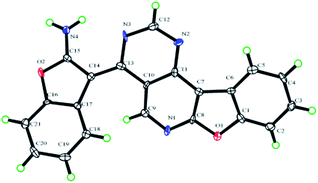 |
| Fig. 2 ORTEP plot of 3a. | |
Therefore, a highly efficient and eco-friendly synthetic method to construct various benzofuran-containing tetracyclic compounds was developed from inexpensive, readily available starting materials via a cascade SNAr/cyclization/condensation reaction and most of these fused-tetracyclic compounds showed antiproliferative activity in vitro. Among the compounds tested, compound 3k and the control etoposide (VP-16) were almost equally potent in SRB assay (Table 3).
Results and discussion
At the outset of our study, the reaction of 2-(2-hydroxyphenyl)acetonitrile (1a) and 4,6-dichloropyrimidine-5-carbaldehyde (2) was used to optimize the reaction conditions (Table 1). Firstly, we wondered whether the catalyst was necessary in the reaction, and finally we were pleased to find that the catalyst-free reaction afforded the product (3a) in an even higher yield compared to the yield with catalyst (Table 1, entries 1 and 2). Then, different bases were tested. Among the base screened, Cs2CO3 offered the best yield (Table 1, entries 2–5). Next, the solvent THF and toluene were examined and found to provide moderate yields (Table 1, entries 6 and 7). The amount of base was modulated. By decreasing the amount of Cs2CO3 (Table 1, entries 8 and 9), a slightly lower yield was obtained. Finally, when the reaction temperature was raised to 120 °C, the reaction was completed within 1 h yet with a decreased yield (Table 1, entry 10). The yield of product reduced to 73% when the reaction temperature was lowered to 80 °C (Table 1, entry 11). In addition, the reaction time was assessed (Table 1, entries 12 and 13) and we found the reaction couldn't perform completely in 0.5 h. Neither did longer reaction times afford higher yields. Hence, the optimal reaction involved 1a (0.4 mmol), 2 (0.2 mmol), DMF (3 mL) and Cs2CO3 (3.0 equiv.) at 100 °C for 1 h under a nitrogen atmosphere.
Table 1 Optimization of the reaction conditionsa
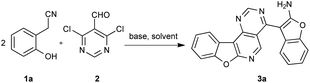
|
Entry |
Base (eq.) |
CuI (eq.) |
Solvent |
Time (h) |
Temp. (°C) |
Yieldb (%) |
Reaction conditions: 1a (53 mg, 0.4 mmol), 2 (35 mg, 0.2 mmol), solvent (3 mL) under N2.
Isolated yield of 3a.
|
1 |
Cs2CO3(3) |
0.05 |
DMF |
1 |
100 |
74 |
2 |
Cs2CO3(3) |
0 |
DMF |
1 |
100 |
84 |
3 |
K2CO3(3) |
0 |
DMF |
1 |
100 |
50 |
4 |
EtONa(3) |
0 |
DMF |
1 |
100 |
40 |
5 |
DBU(3) |
0 |
DMF |
1 |
100 |
63 |
6 |
Cs2CO3(3) |
0 |
toluene |
1 |
100 |
43 |
7 |
Cs2CO3(3) |
0 |
THF |
1 |
65 |
46 |
8 |
Cs2CO3(2) |
0 |
DMF |
1 |
100 |
73 |
9 |
Cs2CO3(1.2) |
0 |
DMF |
1 |
100 |
63 |
10 |
Cs2CO3(3) |
0 |
DMF |
1 |
120 |
74 |
11 |
Cs2CO3(3) |
0 |
DMF |
1 |
80 |
73 |
12 |
Cs2CO3(3) |
0 |
DMF |
0.5 |
100 |
66 |
13 |
Cs2CO3(3) |
0 |
DMF |
2 |
100 |
79 |
The scope and limitations of the reaction were investigated under the optimized reaction conditions. As illustrated in Table 2, both electron-donating and electron-withdrawing groups substituted 2-(2-hydroxyphenyl)acetonitrile could be transformed into the desired products. The yields ranged from 51% to 85%. The positions of substituent had no significant influence on this transformation. Substrates possessing weak electron-withdrawing groups such as chloride and bromine were successfully employed in the reaction (3d, 3i–j), while the fluoride substituent gave a slightly lower yield (3c, 3h). Functional groups at the 3-position included –OCH3 and –F gave similar results (3b, 3c). Both alkyl and aromatic groups on the 2-(2-hydroxyphenyl)acetonitriles were suitable for substrates (3k–l, 3o–p).
Table 2 Synthesis of 3a–3p under optimized conditionsa
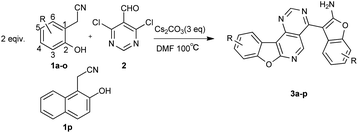
|
Entry |
Substrate |
R |
Product |
Yieldb (%) |
Reaction conditions: 1a–1p (0.4 mmol), 2 (35 mg, 0.2 mmol), Cs2CO3 (195 mg, 0.6 mmol), DMF (3 mL) at 100 °C for 1 h under N2.
Isolated yield of 3.
|
1 |
1a
|
H |
3a
|
84 |
2 |
1b
|
3-OCH3 |
3b
|
68 |
3 |
1c
|
3-F |
3c
|
64 |
4 |
1d
|
4-Br |
3d
|
73 |
5 |
1e
|
4-N(CH3)2 |
3e
|
58 |
6 |
1f
|
4-OCH3 |
3f
|
51 |
7 |
1g
|
4-CF3 |
3g
|
57 |
8 |
1h
|
5-F |
3h
|
53 |
9 |
1i
|
5-Cl |
3i
|
85 |
10 |
1j
|
5-Br |
3j
|
72 |
11 |
1k
|
5-CH3 |
3k
|
55 |
12 |
1l
|
5-CH(CH3)2 |
3l
|
78 |
13 |
1m
|
5-NO2 |
3m
|
69 |
14 |
1n
|
5-CN |
3n
|
51 |
15 |
1o
|
5-phenyl |
3o
|
74 |
16 |
1p
|
— |
3p
|
79 |
Next, the scope of compound 2 was expanded to other halogen substituted aromatic aldehydes. No desired product was obtained when 2,6-dibromobenzaldehyde/3,5-dichloroisonicotinaldehyde was employed even under copper-assisted conditions. 2,6-Dibromobenzaldehyde gave condensation products and only a trace product could be detected by LC-MS when 3,5-dibromoisonicotinaldehyde was used in this reaction.
A mechanistic proposal for the synthesis is depicted in Scheme 2. The starting material 1a and 2 underwent a nucleophilic aromatic substitution (SNAr) reaction to obtain intermediate A, followed by sequential cyclization to form the intermediate B, which could undergo isomerization to afford C. The mechanism of cyclization was similar to the literature.4a Finally, the intermediate C underwent dehydrated aromatization to form the product 3a.
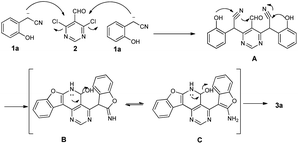 |
| Scheme 2 Proposed reaction mechanism. | |
Subsequently, some of these products have been evaluated for their antitumor activity against human hepatic carcinoma cell line BEL-7402, colon cancer cell line HT29 and stomach cancer cell line SGC-7901. The results were summarized in Table 3. It suggested that compounds 3g–k showed certain antitumor activities, especially the compound 3k had almost equally potency with VP-16.
Table 3 Inhibitory activity (IC50) of some compounds in vitroa
Compd |
IC50b,c [μM] |
BEL-7402 |
HT-29 |
SGC-7901 |
The experiments were based on using the SRB assay.
Exposure time: 72 h.
The average IC50 values were determined by at least three independent tests.
|
3a
|
95.50 |
78.73 |
>100 |
3e
|
>100 |
85.17 |
>100 |
3f
|
4.36 |
7.38 |
>100 |
3g
|
50.23 |
24.00 |
74.10 |
3h
|
12.59 |
13.67 |
11.04 |
3i
|
13.24 |
17.59 |
11.73 |
3j
|
8.20 |
16.57 |
7.45 |
3k
|
5.36 |
4.63 |
4.45 |
3l
|
>100 |
51.87 |
>100 |
3n
|
>100 |
>100 |
>100 |
VP-16 |
2.99 |
4.84 |
10.03 |
Conclusions
In summary, we have completed the catalyst-free synthesis of novel benzofuran-fused pyrido[4,3-d]pyrimidines from two simple substrates via one-pot domino reaction under mild conditions. The flexibility of usable substrates indicated that various substituted 2-(2-hydroxyphenyl)acetonitriles were suitable for this reaction. To our satisfaction, we could obtain novel benzofuran-fused pyrido[4,3-d]pyrimidines with good antitumor activity and the synthetic method involved the formation of at least ten new bonds and three new rings through a one pot, eco-friendly cascade reaction.
Experimental
General information
Analytical thin layer chromatography (TLC) was HSGF254 (0.15–0.2 mm thickness, Yantai Huiyou Company, China). Column chromatography was carried out on silica gel (200–300 mesh). Proton and carbon magnetic resonance spectra (1H NMR and 13C NMR) were recorded on Varian Mercury-300/400 and Varian Mercury-400/500 spectrometers. Tetramethylsilane (TMS) was used as internal standard. Chemical shifts (δ = ) are reported in parts per million (ppm). Data are reported as follows: chemical shift, multiplicity (brs = broad singlet, d = doublet, dd = doublet of doublet, dt = doublet of triplet, td = triplet of doublet, m = multiple, s = singlet and t = triplet), coupling constants (Hz). IR spectra were recorded on a FTIR instrument. HRMS spectra were recorded on a Finnigan/MAT-95 spectrometer. Melting points were measured by Büchi 510 melting point apparatus and were uncorrected.
General procedure of biology
The sulforhodamine B (SRB) assay: cells were seeded into 96-well plates on day 0 and exposed to 2-fold serial drug dilutions on day 1. On day 4, the cells were fixed by adding 10% pre-cooled trichloroacetic acid. After 1 h at 4 °C, the plates were washed with distilled water, dried, and then stained with SRB (Sigma, MO) in 1% acetic acid. SRB in the cells was dissolved in 10 mM Tris–HCl and was measured at 515 nm using spectra-MAX190 (Molecular Devices, CA). The cell proliferation inhibition rate was calculated as: proliferation inhibition (%) = [1 − (A515treated/A515control)] × 100%.
General procedure for the synthesis of compounds 3a–3p
2-(2-Hydroxyphenyl)acetonitrile 1a (53 mg, 0.4 mmol), 4,6-dichloropyrimidine-5-carbaldehyde 2 (35 mg, 0.2 mmol), Cs2CO3 (195 mg, 0.6 mmol) and anhydrous DMF (3 mL) were added to an oven-dried 25 mL two-necked reaction flask. Then the system was degassed and filled with nitrogen. The reaction mixture was stirred and heated at 100 °C for 1 h. After completion of the reaction, the resulting solution was cooled to room temperature, the mixture was filtrated and the solvent was removed under reduced pressure. The residue was purified by column chromatography on silica gel using petroleum ether/ethyl acetate (5
:
1) as the eluent to provide the desired products 3a. (3a) contains the supplementary crystallographic data for this paper.
3-(Benzofuro[3′,2′:5,6]pyrido[4,3-d]pyrimidin-4-yl)benzofuran-2-amine (3a)
Yellow solid (84%), m.p. 276–278 °C; 1H NMR (400 MHz, DMSO-d6) δ = 9.48 (s, 1H), 9.29 (s, 1H), 8.51 (d, J = 7.7 Hz, 1H), 8.46 (brs, 2H), 7.89 (d, J = 8.2 Hz, 1H), 7.64 (td, J = 7.8, 1.4 Hz, 1H), 7.60–7.54 (m, 2H), 7.43 (d, J = 8.0 Hz, 1H), 7.20 (td, J = 7.5, 1.1 Hz, 1H), 7.12 (td, J = 7.8, 1.1 Hz, 1H); 13C NMR (125 MHz, DMSO-d6) δ = 164.43, 163.94, 163.34, 158.38, 153.92, 151.30, 149.97, 149.84, 128.55, 128.37, 124.93, 124.12, 123.88, 122.57, 122.10, 118.09, 114.63, 112.53, 110.37, 108.63, 89.12; IR (KBr) 3386, 3069, 2952, 2922, 1658, 1602, 1562, 1520, 1496, 1452, 1377, 1315, 1196, 1053, 742 cm−1; HRMS (EI): m/z [M+] calcd for C21H12O2N4: 352.0960, found: 352.0954.
7-Methoxy-3-(8-methoxybenzofuro[3′,2′:5,6]pyrido[4,3-d]pyrimidin-4-yl)benzofuran-2-amine (3b)
Yellow solid (68%), m.p. 288–290 °C; 1H NMR (300 MHz, DMSO-d6) δ = 9.46 (s, 1H), 9.27 (s, 1H), 8.35 (brs, 2H), 8.06 (d, J = 7.6 Hz, 1H), 7.47 (t, J = 8.0 Hz, 1H), 7.27 (d, J = 8.2 Hz, 1H), 7.17–7.07 (m, 2H), 6.81 (d, J = 7.2 Hz, 1H), 4.03 (s, 3H), 3.92 (s, 3H); 13C NMR (125 MHz, DMSO-d6) δ = 164.21, 163.69, 163.35, 158.32, 151.37, 149.99, 145.55, 144.34, 142.82, 138.12, 130.01, 125.66, 124.80, 123.90, 115.59, 114.58, 110.87, 110.83, 108.79, 105.93, 89.48, 56.53, 56.31; IR (KBr) 3431, 3317, 2964, 1624, 1597, 1562, 1537, 1520, 1493, 1377, 1180, 1057, 766, 727 cm−1; HRMS (EI): m/z [M+] calcd for C23H16O4N4: 412.1172, found: 412.1167.
7-Fluoro-3-(8-fluorobenzofuro[3′,2′:5,6]pyrido[4,3-d]pyrimidin-4-yl)benzofuran-2-amine (3c)
Yellow solid (64%), m.p. 278–280 °C; 1H NMR (400 MHz, DMSO-d6) δ = 9.49 (s, 1H), 9.35 (s, 1H), 8.51 (brs, 2H), 8.31 (dd, J = 6.9, 1.9 Hz, 1H), 7.62–7.54 (m, 2H), 7.37 (dd, J = 7.8, 0.6 Hz, 1H), 7.21–7.14 (m, 1H), 7.05–6.99 (m, 1H); 13C NMR (125 MHz, DMSO-d6) δ = 164.46, 164.05, 163.24, 158.71, 151.40, 150.99, 147.53 (d, 1JCF = 248.43 Hz), 146.74 (d, 1JCF = 244.41 Hz), 140.49 (d, 2JCF = 10.26 Hz), 135.95 (d, 2JCF = 10.56 Hz), 132.39 (d, 4JCF = 2.28 Hz), 126.04 (d, 3JCF = 6.64 Hz), 125.84 (d, 4JCF = 2.22 Hz), 125.12 (d, 3JCF = 6.64 Hz), 119.85, 114.98, 114.77 (d, 2JCF = 14.18 Hz), 114.12 (d, 4JCF = 3.00 Hz), 108.80 (d, 2JCF = 16.00 Hz), 108.60 (d, 4JCF = 2.28 Hz), 89.18; IR (KBr) 3444, 3327, 1633, 1597, 1564, 1520, 1493, 1437, 1381, 1315, 1257, 1194, 1051, 771 cm−1; HRMS (EI): m/z [M+] calcd for C21H10O2N4F2: 388.0772, found: 388.0744.
6-Bromo-3-(9-bromobenzofuro[3′,2′:5,6]pyrido[4,3-d]pyrimidin-4-yl)benzofuran-2-amine (3d)
Yellow solid (73%), m.p. 278–280 °C; 1H NMR (400 MHz, DMSO-d6) δ = 9.44 (s, 1H), 9.31 (s, 1H), 8.49 (brs, 2H), 8.40 (d, J = 8.3 Hz, 1H), 8.24 (d, J = 1.3 Hz, 1H), 7.74 (dd, J = 8.3, 1.5 Hz, 1H), 7.71 (d, J = 1.6 Hz, 1H), 7.50 (d, J = 8.3 Hz, 1H), 7.35 (dd, J = 8.3, 1.6 Hz, 1H); 13C NMR (125 MHz, DMSO-d6) δ = 164.49, 164.02, 163.08, 158.53, 154.20, 151.16, 150.52, 150.19, 128.23, 126.94, 125.12, 121.85, 120.72, 119.29, 115.94, 114.86, 113.49, 113.35, 108.27, 88.79; IR (KBr) 3429, 3070, 1633, 1600, 1560, 1523, 1489, 1373, 1186, 1047, 889, 808 cm−1; HRMS (EI): m/z [M+] calcd for C21H10O2N4Br2: 507.9171, found: 507.9153.
3-(9-(Dimethylamino)benzofuro[3′,2′:5,6]pyrido[4,3-d]pyrimidin-4-yl)-N6,N6-dimethylbenzofuran-2,6-diamine (3e)
Brown solid (58%), m.p. > 300 °C; 1H NMR (400 MHz, DMSO-d6) δ = 9.30 (s, 1H), 9.11 (s, 1H), 8.49 (brs, 2H), 8.21 (d, J = 8.7 Hz, 1H), 7.41 (d, J = 8.6 Hz, 1H), 7.06 (d, J = 2.1 Hz, 1H), 6.94 (dd, J = 8.9, 2.2 Hz, 1H), 6.83 (d, J = 2.1 Hz, 1H), 6.65 (dd, J = 8.7, 2.1 Hz, 1H), 3.05 (s, 6H), 2.91 (s, 6H); 13C NMR (125 MHz, DMSO-d6) δ = 164.22, 163.32, 162.54, 157.41, 156.47, 151.70, 151.38, 149.86, 147.79, 145.73, 123.96, 118.80, 117.18, 114.48, 111.21, 110.60, 109.75, 109.48, 95.69, 94.49, 89.29, 41.28, 40.80; IR (KBr) 3323, 3234, 3062, 2887, 2806, 1630, 1614, 1599, 1556, 1506, 1437, 1385,1319, 1227, 1105, 989, 808 cm−1; HRMS (EI): m/z [M+] calcd for C25H22O2N6: 438.1804, found: 438.1791.
6-Methoxy-3-(9-methoxybenzofuro[3′,2′:5,6]pyrido[4,3-d]pyrimidin-4-yl)benzofuran-2-amine (3f)
Brown solid (51%), m.p. 234–236 °C; 1H NMR (400 MHz, DMSO-d6) δ = 9.37 (s, 1H), 9.18 (s, 1H), 8.50 (brs, 1H), 8.34 (d, J = 8.3 Hz, 1H), 7.51–7.43 (m, 2H), 7.18–7.07 (m, 2H), 6.82 (d, J = 8.1 Hz, 1H), 3.90 (s, 3H), 3.80 (s, 3H); 13C NMR (125 MHz, DMSO-d6) δ = 164.62, 163.86, 162.75, 160.41, 157.63, 156.16, 155.45, 150.85, 147.67, 124.23, 121.01, 118.60, 115.35, 114.37, 113.38, 113.29, 110.80, 108.85, 97.36, 97.15, 89.27, 56.33, 56.15; IR (KBr) 3431, 2939, 2188, 1620, 1604, 1560, 1437, 1377, 1311, 1142, 1032, 823 cm−1; HRMS (EI): m/z [M+] calcd for C23H16O4N4: 412.1172, found: 412.1154.
6-(Trifluoromethyl)-3-(9-(trifluoromethyl)benzofuro[3′,2′:5,6]pyrido[4,3-d]pyrimidin-4-yl)benzofuran-2-amine (3g)
Yellow solid (57%), m.p. > 300 °C; 1H NMR (300 MHz, DMSO-d6) δ = 9.46 (s, 1H), 9.36 (s, 1H), 8.63 (d, J = 8.1 Hz, 1H), 8.59 (brs, 2H), 8.35 (s, 1H), 7.88 (d, J = 7.2 Hz, 1H), 7.77 (s, 1H), 7.67 (d, J = 8.2 Hz, 1H), 7.50 (d, J = 7.3 Hz, 1H); 13C NMR (125 MHz, DMSO-d6) δ = 165.52, 164.81, 162.98, 158.75, 153.05, 151.59, 151.41, 149.02, 132.71, 128.20 (d, 2JCF = 31.66 Hz), 125.90, 125.16 (d,1JCF = 270.97 Hz),124.60 (d,1JCF = 273.18 Hz), 124.52, 121.90 (d, 2JCF = 32.53 Hz), 121.65, 121.24, 117.89, 114.78, 110.21 (d, 3JCF = 4.17 Hz), 107.77, 107.38 (d, 3JCF = 4.17 Hz), 88.88; IR (KBr) 3419, 3300, 1637, 1624, 1606, 1566, 1512, 1479, 1433, 1325, 1117, 1057, 910 cm−1; HRMS (EI): m/z [M+] calcd for C23H10O2N4F6: 488.0708, found: 488.0693.
5-Fluoro-3-(10-fluorobenzofuro[3′,2′:5,6]pyrido[4,3-d]pyrimidin-4-yl)benzofuran-2-amine (3h)
Yellow solid (53%), m.p. 274–276 °C; 1H NMR (500 MHz, DMSO-d6) δ = 9.42 (s, 1H), 9.29 (s, 1H), 8.45 (brs, 2H), 8.08 (dd, J = 8.0, 2.7 Hz, 1H), 7.93 (dd, J = 9.0, 4.0 Hz, 1H), 7.49 (td, J = 9.2, 2.7 Hz, 2H), 7.41 (dd, J = 8.8, 4.3 Hz, 1H), 7.31 (dd, J = 9.6, 2.6 Hz, 1H), 6.90 (td, J = 9.1, 2.6 Hz, 1H); 13C NMR (125 MHz, DMSO-d6) δ = 165.29, 164.71, 163.02, 160.55 (d, 1JCF = 236.43 Hz), 159.38 (d, 1JCF = 240.09 Hz), 158.49, 151.18, 150.70, 150.08, 146.00, 130.22 (d, 3JCF = 11.65 Hz), 123.33 (d, 3JCF = 11.06 Hz), 115.86 (d, 2JCF = 25.80 Hz), 114.54, 114.08 (d, 3JCF = 9.88 Hz), 111.03 (d, 3JCF = 9.73 Hz), 109.24 (d, 2JCF = 25.85 Hz), 108.53 (d, 4JCF = 3.32 Hz), 108.28 (d, 2JCF = 25.52 Hz), 104.51 (d, 2JCF = 26.83 Hz), 89.39 (d, 4JCF = 2.73 Hz); IR (KBr) 3406, 3213, 3138, 2920, 1712, 1637, 1606, 1558, 1520, 1502, 1446, 1356, 1311, 1188, 1047, 793 cm−1; HRMS (EI): m/z [(M − H)+] calcd for C21H9O2N4F2: 387.0694, found: 387.0684.
5-Chloro-3-(10-chlorobenzofuro[3′,2′:5,6]pyrido[4,3-d]pyrimidin-4-yl)benzofuran-2-amine (3i)
Yellow solid (85%), m.p. 280–282 °C; 1H NMR (400 MHz, DMSO-d6) δ = 9.36 (s, 1H), 9.25 (s, 1H), 8.45 (brs, 2H), 8.26 (d, J = 2.2 Hz, 1H), 7.86 (d, J = 8.8 Hz, 1H), 7.61 (dd, J = 8.8, 2.3 Hz, 1H), 7.54 (d, J = 2.1 Hz, 1H), 7.40 (d, J = 8.5 Hz, 1H), 7.08 (dd, J = 8.5, 2.2 Hz, 1H); 13C NMR (125 MHz, DMSO-d6) δ = 164.92, 164.27, 162.90, 158.50, 152.23, 151.05, 150.75, 148.35, 130.63, 129.07, 128.46, 128.16, 123.82, 122.73, 121.41, 117.43, 114.62, 114.25, 111.54, 107.83, 88.72; IR (KBr), 3485, 3398, 2922, 1647, 1604, 1564, 1522, 1500, 1464, 1365, 1317, 1165, 795 cm−1; HRMS (EI): m/z [(M − H)+] calcd for C21H9O2N4Cl2: 419.0103, found: 419.0085.
5-Bromo-3-(10-bromobenzofuro[3′,2′:5,6]pyrido[4,3-d]pyrimidin-4-yl)benzofuran-2-amine (3j)
Yellow solid (72%), m.p. 262–264 °C; 1H NMR (300 MHz, DMSO-d6) δ = 9.43 (d, J = 1.7 Hz, 1H), 9.32 (d, J = 1.6 Hz, 1H), 8.53 (s, 1H), 8.41 (s, 2H), 7.88 (dd, J = 7.4, 1.4 Hz, 1H), 7.80–7.76 (m, 1H), 7.71 (d, J = 2.0 Hz, 1H), 7.37 (d, J = 8.6 Hz, 1H), 7.22 (dd, J = 8.5, 2.0 Hz, 1H); 13C NMR (125 MHz, DMSO-d6) δ = 164.73, 164.12, 162.93, 158.55, 152.62, 151.07, 150.81, 148.74, 131.20, 130.86, 125.71, 124.39, 124.20, 120.28, 116.93, 116.45, 114.68, 112.06, 107.69, 88.58; IR (KBr) 3442, 3136, 2920, 1633, 1606, 1560, 1516, 1495, 1442, 1356, 1311, 1186, 1039, 795, 667 cm−1; HRMS (EI): m/z [M+] calcd for C21H10O2N4Br2: 507.9171, found: 507.9136.
5-Methyl-3-(10-methylbenzofuro[3′,2′:5,6]pyrido[4,3-d]pyrimidin-4-yl)benzofuran-2-amine (3k)
Yellow solid (55%), m.p. 220–222 °C; 1H NMR (300 MHz, CDCl3) δ = 9.69 (s, 1H), 9.27 (s, 1H), 8.42 (brs, 2H), 7.63 (d, J = 8.4 Hz, 1H), 7.42–7.37 (m, 2H), 7.21 (d, J = 8.3 Hz, 1H), 6.98–6.93 (m, 2H), 2.59 (s, 3H), 2.39 (s, 3H); 13C NMR (125 MHz, CDCl3) δ = 164.37, 163.74, 163.58, 157.26, 152.61, 151.39, 149.31, 148.36, 134.02, 133.65, 128.90, 127.46, 123.82, 123.24, 122.60, 118.87, 114.56, 111.45, 109.82, 109.15, 90.79, 21.69, 21.48; IR (KBr) 3421, 2922, 1852, 2185, 1643, 1601, 1564, 1520, 1496, 1469, 1360. 1317, 1188, 1047, 798 cm−1; HRMS (EI): m/z [M+] calcd for C23H16O2N4: 380.1273, found: 380.1251.
5-Isopropyl-3-(10-isopropylbenzofuro[3′,2′:5,6]pyrido[4,3-d]pyrimidin-4-yl)benzofuran-2-amine (3l)
Yellow solid (78%), m.p. 238–240 °C; 1H NMR (300 MHz, CDCl3) δ = 9.72 (s, 1H), 9.30 (s, 1H), 8.49 (d, J = 1.9 Hz, 1H), 7.68 (d, J = 8.5 Hz, 1H), 7.49–7.45 (m, 2H), 7.29–7.25 (m, 1H), 7.04 (dd, J = 8.3, 1.7 Hz, 1H), 6.94 (brs, 2H), 3.25–3.14 (m, 1H), 3.02-2.92 (m, 1H), 1.42 (s, 3H), 1.40 (s, 3H), 1.28 (s, 3H), 1.26 (s, 3H); 13C NMR (125 MHz, CDCl3) δ = 164.52, 163.76, 163.57, 157.32, 152.80, 151.50, 149.33, 148.53, 145.32, 145.04, 127.45, 126.40, 122.65, 121.46, 120.63, 116.48, 114.63, 111.62, 109.92, 109.39, 91.00, 34.41, 34.32, 24.57; IR (KBr) 3388, 3213, 2958, 2925, 2868, 1633, 1601, 1560, 1495, 1469, 1365, 1315, 1190, 1043, 808 cm−1; HRMS (EI): m/z [M+] calcd for C27H24O2N4: 436.1899, found:436.1884.
5-Nitro-3-(10-nitrobenzofuro[3′,2′:5,6]pyrido[4,3-d]pyrimidin-4-yl)benzofuran-2-amine (3m)
Yellow solid (69%), m.p. > 300 °C; 1H NMR (300 MHz, DMSO-d6) δ = 9.49 (s, 1H), 9.39 (s, 1H), 9.08 (d, J = 2.5 Hz, 1H), 8.57 (brs, 2H), 8.46 (dd, J = 9.0, 2.4 Hz, 1H), 8.37 (d, J = 2.5 Hz, 1H), 8.11 (d, J = 9.1 Hz, 1H), 7.97 (dd, J = 8.8, 2.2 Hz, 1H), 7.59 (d, J = 8.9 Hz, 1H); 13C NMR (125 MHz, Py-d5) δ = 164.54, 159.95, 158.53, 154.92, 153.16, 152.95, 152.91, 146.45, 146.41, 131.35, 121.33, 119.41, 116.54, 114.90, 114.34, 111.79, 110.43, 90.92; IR (KBr) 3384, 3095, 1651, 1612, 1564, 1516, 1448, 1342, 1194, 1047, 818 cm−1; HRMS (EI): m/z [M+] calcd for C21H10O6N6: 442.0662, found:442.0600.
4-(2-Amino-5-cyanobenzofuran-3-yl)benzofuro[3′,2′:5,6]pyrido[4,3-d]pyrimidine-10-carbonitrile (3n)
Yellow solid (51%), m.p. > 300 °C; 1H NMR (300 MHz, DMSO-d6) δ = 9.47 (s, 1H), 9.37 (s, 1H), 8.76 (s, 1H), 8.53 (brs, 2H), 8.16–8.06 (m, 2H), 7.96 (s, 1H), 7.63–7.51 (m, 2H); 13C NMR (125 MHz, DMSO-d6) δ = 164.90, 164.42, 162.82, 158.78, 155.62, 151.90, 151.55, 151.09, 132.11, 130.03, 127.80, 126.30, 123.12, 121.55, 119.92, 119.05, 114.95, 114.25, 111.45, 107.80, 107.59, 106.84, 88.23; IR (KBr) 3365, 3240, 3078, 2229, 1651, 1604, 1562, 1495, 1518, 1460, 1358, 1317, 1196, 1047, 820 cm−1; HRMS (EI): m/z [M+] calcd for C23H10O2N6: 402.0865, found:402.0827.
5-Phenyl-3-(10-phenylbenzofuro[3′,2′:5,6]pyrido[4,3-d]pyrimidin-4-yl)benzofuran-2-amine (3o)
Yellow solid (74%), m.p. 188–190 °C; 1H NMR (300 MHz, DMSO-d6) δ = 9.53 (s, 1H), 9.31 (s, 1H), 8.65 (s, 1H), 8.48 (brs, 2H), 7.96–7.85 (m, 2H), 7.82 (s, 1H), 7.74 (d, J = 7.4 Hz, 2H), 7.60 (d, J = 7.7 Hz, 2H), 7.54–7.46 (m, 3H), 7.45–7.30 (m, 5H); 13C NMR (125 MHz, CDCl3) δ = 164.51, 163.71, 163.47, 157.35, 153.75, 151.45, 149.58, 149.50, 141.26, 140.90, 137.87, 137.85, 128.83, 128.01, 127.59, 127.42, 127.26, 127.14, 123.13, 122.45, 121.80, 117.21, 114.65, 112.03, 110.37, 109.38, 90.85; IR (KBr) 3431, 3059, 2920, 1727, 1628, 1601, 1560, 1493, 1462, 1358, 1315, 1192, 1047, 762, 698 cm−1; HRMS (EI): m/z [M+] calcd for C33H20O2N4: 504.1586, found:504.1585.
1-(Naphtho[1′′,2′′:4′,5′]furo[3′,2′:5,6]pyrido[4,3-d]pyrimidin-4-yl)naphtho[2,1 b]furan-2-amine (3p)
Yellow solid (79%), m.p. 230–232 °C; 1H NMR (400 MHz, DMSO-d6) δ = 10.66 (d, J = 3.2 Hz, 1H), 10.36 (d, J = 8.2 Hz, 1H), 10.22 (s, 1H), 10.03 (s, 1H), 8.19–8.07 (m, 2H), 8.01–7.92 (m, 4H), 7.89 (d, J = 8.4 Hz, 1H), 7.65 (t, J = 7.6 Hz, 1H), 7.61–7.51 (m, 2H), 7.43–7.34 (m, 2H); 13C NMR (125 MHz, DMSO-d6) δ = 164.27, 155.01, 152.75, 148.97, 147.71, 144.61, 144.43, 133.40, 131.60, 131.22, 131.18, 129.42, 129.39, 129.19, 129.06, 128.84, 127.81, 127.49, 125.64, 123.68, 123.46, 122.69, 119.57, 117.75, 114.05, 112.78, 112.11, 109.66, 72.59; IR (KBr) 3354, 3060, 2181, 1612, 1576, 1516, 1400, 1362, 1255, 1188, 1032, 814, 750 cm−1; HRMS (EI): m/z [M+] calcd for C29H16O2N4:452.1273, found:452.1271.
Acknowledgements
This work was supported by the “Interdisciplinary Cooperation Team” Program for Science and Technology Innovation of the Chinese Academy of Sciences, and the National Natural Science Foundation of China (21072205, 81025020) and SIMM1203ZZ-0103.
Notes and references
-
(a) R. K. Shiroodi and V. Gevorgyan, Chem. Soc. Rev., 2013, 42, 4991–5001 RSC;
(b) C. J. Ball and M. C. Willis, Eur. J. Org. Chem., 2013, 425–441 CrossRef CAS;
(c) Q.-Q. Yang, C. Xiao, L.-Q. Lu, J. An, F. Tan, B.-J. Li and W.-J. Xiao, Angew. Chem., Int. Ed., 2012, 51, 9137–9140 CrossRef CAS PubMed;
(d) L.-Q. Lu, J.-R. Chen and W.-J. Xiao, Acc. Chem. Res., 2012, 45, 1278–1293 CrossRef CAS PubMed;
(e) C. Grondal, M. Jeanty and D. Enders, Nat. Chem., 2010, 2, 167–178 CrossRef CAS PubMed.
- S. F. Kirsch, Synthesis-stuttgart, 2008, 3183–3204 CrossRef CAS PubMed.
-
(a) K. C. Nicolaou and J. S. Chen, Chem. Soc. Rev., 2009, 38, 2993–3009 RSC;
(b) K. C. Nicolaou, D. J. Edmonds and P. G. Bulger, Angew Chem., Int. Ed., 2006, 45, 7134–7186 CrossRef CAS PubMed.
-
(a) C. K. Ryu, Y. H. Kim, J. H. Nho, J. A. Hong, J. H. Yoon and A. Kim, Bioorg. Med. Chem. Lett., 2011, 21, 952–955 CrossRef CAS PubMed;
(b) C. K. Ryu, Y. H. Kim, H. A. Im, J. Y. Kim, J. H. Yoon and A. Kim, Bioorg. Med. Chem. Lett., 2012, 22, 500–503 CrossRef CAS PubMed.
- B. L. Flynn, G. S. Gill, D. W. Grobelny, J. H. Chaplin, D. Paul, A. F. Leske, T. C. Lavranos, D. K. Chalmers, S. A. Charman, E. Kostewicz, D. M. Shackleford, J. Morizzi, E. Hamel, M. K. Jung and G. Kremmidiotis, J. Med. Chem., 2011, 54, 6014–6027 CrossRef CAS PubMed.
- R. Haudecoeur, A. Ahmed-Belkacem, W. Yi, A. Fortune, R. Brillet, C. Belle, E. Nicolle, C. Pallier, J. M. Pawlotsky and A. Boumendjel, J. Med. Chem., 2011, 54, 5395–5402 CrossRef CAS PubMed.
- Y. He, J. Xu, Z. H. Yu, A. M. Gunawan, L. Wu, L. Wang and Z. Y. Zhang, J. Med. Chem., 2013, 56, 832–842 CrossRef CAS PubMed.
-
(a) C. L. Yang, C. H. Tseng, Y. L. Chen, C. M. Lu, C. L. Kao, M. H. Wu and C. C. Tzeng, Eur. J. Med. Chem., 2010, 45, 602–607 CrossRef CAS PubMed;
(b) V. Tell, M. Holzer, L. Herrmann, K. A. Mahmoud, C. Schachtele, F. Totzke and A. Hilgeroth, Bioorg. Med. Chem. Lett., 2012, 22, 6914–6918 CrossRef CAS PubMed;
(c) D. Drygin, M. Haddach, F. Pierre and D. M. Ryckman, J. Med. Chem., 2012, 55, 8199–8208 CrossRef CAS PubMed;
(d) G. Rodriguez-Berna, M. J. D. Cabañas, V. Mangas-Sanjuán, M. Gonzalez-Alvarez, I. Gonzalez-Alvarez, I. Abasolo, S. Schwartz, M. Bermejo and A. Corma, ACS Med. Chem. Lett., 2013, 4, 651–655 CrossRef CAS;
(e) R. Chaniyara, S. Tala, C. W. Chen, X. G. Zang, R. Kakadiya, L. F. Lin, C. H. Chen, S. I. Chien, T. C. Chou, T. H. Tsai, T. C. Lee, A. Shah and T. L. Su, J. Med. Chem., 2013, 56, 1544–1563 CrossRef CAS PubMed;
(f) M. Conda-Sheridan, P. V. Reddy, A. Morrell, B. T. Cobb, C. Marchand, K. Agama, A. Chergui, A. Renaud, A. G. Stephen, L. K. Bindu, Y. Pommier and M. Cushman, J. Med. Chem., 2013, 56, 182–200 CrossRef CAS PubMed;
(g) C. Tardy, M. Facompre, W. Laine, B. Baldeyrou, D. Garcia-Gravalos, A. Francesch, C. Mateo, A. Pastor, J. A. Jimenez, I. Manzanares, C. Cuevas and C. Bailly, Bioorg. Med. Chem., 2004, 12, 1697–1712 CrossRef CAS PubMed.
-
(a) M. Murai, K. Miki and K. Ohe, Chem. Commun., 2009, 3466–3468 RSC;
(b) X. Wang, M. Liu, L. Xu, Q. Wang, J. Chen, J. Ding and H. Wu, J. Org. Chem., 2013, 78, 5273–5281 CrossRef CAS PubMed;
(c) H. J. Kim, J. W. Seo, M. H. Lee, D. S. Shin, H. B. Kim, H. Cho, B. S. Lee and D. Y. Chi, Tetrahedron, 2012, 68, 3942–3947 CrossRef CAS PubMed;
(d) J. Chen, M. Liu, X. Wang, X. Wang, J. Ding and H. Wu, Synthesis, 2013, 45, 2241–2244 CrossRef PubMed;
(e) B. Anxionnat, D. Gomez Pardo, G. Ricci and J. Cossy, Eur. J. Org. Chem., 2012, 2012, 4453–4456 CrossRef CAS;
(f) Y. Wang, Y. Chen, Q. He, Y. Xie and C. Yang, Helv. Chim. Acta, 2013, 96, 296–308 CrossRef CAS;
(g) C. Yang, H. Xiang, Y. Xie and Y. Chen, Heterocycles, 2011, 83, 1355 CrossRef PubMed;
(h) K. Aplander, O. Hidestål, K. Katebzadeh and U. M. Lindström, Green Chem., 2006, 8, 22 RSC;
(i) Y. L. Choi, H. S. Lim, H. J. Lim and J. N. Hee, Org. Lett., 2012, 14, 5102–5105 CrossRef CAS PubMed;
(j)
A. J. Whittle, Brit. UK Pat. Appl., GB 2388595 A 20031119, 2003;
(k) M. Mąkosza, W. Danikiewicz and K. Wojciechowski, Liebigs Ann. Chem., 1987, 711–715 CrossRef.
-
(a) S. Babu, C. Morrill, N. G. Almstead and Y. C. Moon, Org. Lett., 2013, 15, 1882–1885 CrossRef CAS PubMed;
(b) P. A. Brough, X. Barril, J. Borgognoni, P. Chene, N. G. Davies, B. Davis, M. J. Drysdale, B. Dymock, S. A. Eccles, C. Garcia-Echeverria, C. Fromont, A. Hayes, R. E. Hubbard, A. M. Jordan, M. R. Jensen, A. Massey, A. Merrett, A. Padfield, R. Parsons, T. Radimerski, F. I. Raynaud, A. Robertson, S. D. Roughley, J. Schoepfer, H. Simmonite, S. Y. Sharp, A. Surgenor, M. Valenti, S. Walls, P. Webb, M. Wood, P. Workman and L. Wright, J. Med. Chem., 2009, 52, 4794–4809 CrossRef CAS PubMed;
(c) D. J. Richard, J. C. Verheijen, K. Curran, J. Kaplan, L. Toral-Barza, I. Hollander, J. Lucas, K. Yu and A. Zask, Bioorg. Med. Chem. Lett., 2009, 19, 6830–6835 CrossRef CAS PubMed;
(d) J. M. Harris, B. R. Neustadt, H. Zhang, J. Lachowicz, M. Cohen-Williams, G. Varty, J. Hao and A. W. Stamford, Bioorg. Med. Chem. Lett., 2011, 21, 2497–2501 CrossRef CAS PubMed;
(e) J. Das, R. V. Moquin, S. Pitt, R. Zhang, D. R. Shen, K. W. McIntyre, K. Gillooly, A. M. Doweyko, J. S. Sack, H. Zhang, S. E. Kiefer, K. Kish, M. McKinnon, J. C. Barrish, J. H. Dodd, G. L. Schieven and K. Leftheris, Bioorg. Med. Chem. Lett., 2008, 18, 2652–2657 CrossRef CAS PubMed;
(f) S. Brumfield, P. Korakas, L. S. Silverman, D. Tulshian, J. J. Matasi, L. Qiang, C. E. Bennett, D. A. Burnett, W. J. Greenlee, C. E. Knutson, W. L. Wu, T. K. Sasikumar, M. Domalski, R. Bertorelli, M. Grilli, G. Lozza, A. Reggiani and C. Li, Bioorg. Med. Chem. Lett., 2012, 22, 7223–7226 CrossRef CAS PubMed.
-
(a) N. Desideri, F. Manna, M. L. Stein, G. Bile, W. Filippelli and E. Marmo, Eur. J. Med. Chem., 1983, 18, 295–299 CAS;
(b)
A. J. Whittle, J. J. Swanborough, D. R. Parry and R. L. Sunley, GB2388595A, 2003.
Footnote |
† Electronic supplementary information (ESI) available: Experimental section, characterization data and NMR spectra data. CCDC 945367 and 945368. For ESI and crystallographic data in CIF or other electronic format see DOI: 10.1039/c3ra44828b |
|
This journal is © The Royal Society of Chemistry 2014 |
Click here to see how this site uses Cookies. View our privacy policy here.