DOI:
10.1039/C4QO00231H
(Review Article)
Org. Chem. Front., 2014,
1, 1132-1139
Organometallic intermediate-based organic synthesis: organo-di-lithio reagents and beyond†
Received
18th August 2014
, Accepted 6th September 2014
First published on 12th September 2014
Abstract
Metal-mediated organic reactions have become one of the great frontiers of organic synthesis. These processes usually involve multiple transient or unobservable reactive intermediates. The isolation and study of these reactive organometallic intermediates would not only lead to a better understanding of their reactivity, but also guide the discovery of new reactions, culminating in the advancement of novel synthetic methods. Herein we provide a perspective of a research strategy which focuses on organometallic intermediate-based organic synthesis. Specifically, we highlight the applications of our organo-di-lithio reagents to the synthesis of a diverse range of reactive intermediates and small molecules. A series of organo-di-lithio reagents has been synthesized, isolated, and applied in organic and organometallic synthesis. These isolated dilithio reagents can be transformed into a variety of organo-di-metallic or metallacyclic compounds via transmetallation, which in turn provides access to organic and organometallic compounds with interesting and useful properties. Selected examples by other research groups are also briefly mentioned, in which reactive organometallic intermediates are isolated for a better understanding of reaction mechanisms, and in some cases are further applied to synthesis.
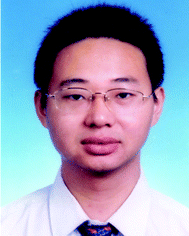 Wen-Xiong Zhang | Wen-Xiong Zhang was born in Hunan Province, China, in 1972. He received his B.Sc. from Hunan Normal University in 1996, his M.Sc. from Guangxi Normal University in 1999, and his Ph.D. from Nankai University with professor Li-Cheng Song in 2003. He carried out postdoctoral research at Peking University with Prof. Zhenfeng Xi and at Riken in Japan with Prof. Zhaomin Hou. In 2007, he joined the College of Chemistry at Peking University, where he is now an Associate Professor. His research focuses on the design, synthesis and small molecule activation of organolanthanides, and carbodiimide-based organic synthesis to construct N-containing heterocycles. |
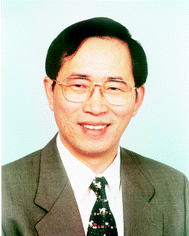 Zhenfeng Xi | Zhenfeng Xi was born in 1963 in Henan Province, China. He received his B.Sc. from Xiamen University in 1983, M.Sc. from Nanjing University, Zhengzhou University and the Henan Institute of Chemistry with Prof. Douman Jin in 1989, and Ph.D. from the Institute for Molecular Sciences, Japan, with Professor Tamotsu Takahashi in 1996. He became an Assistant Professor at Hokkaido University in 1997. In 1998, he joined the College of Chemistry at Peking University, where he is now a Professor. Prof. Xi's current research interests mainly include development of organo-di-metallic reagents, and reactive organometallic intermediate-based organic synthesis. |
1. Organometallic intermediate-based organic synthesis: the strategy
Organometallic compounds, either as reagents or catalysts, are widely applied in synthetic chemistry.1 Traditionally, the synthesis, isolation, spectroscopic properties, and structural characterization of organometallic compounds have been the main interests of inorganic chemists. On the other hand, organic chemists are more generally concerned with the application of inorganic and organometallic complexes to mediate or catalyze organic transformations. Despite what appears to be a fruitful middle ground for research between these two areas, there has been limited activity in organometallic intermediate-based organic synthesis in recent years. The identification of many of the intermediates in metal-mediated and catalyzed organic reactions has fallen between the cracks. Filling this gap should benefit both areas and advance the development of synthetic chemistry as a whole.2
Usually, as illustrated in Scheme 1, after the discovery of a new reaction, the researchers would (or should) be interested in the reaction mechanism. They may try to isolate and characterize the reactive organometallic intermediates, in addition to other ways of investigating the reaction mechanism. Elucidating a mechanism in most cases is much more challenging and may require much more diversified skills. Based on the study of organometallic intermediates and other mechanistic evidence, a possible reaction pathway will be formulated, and perhaps further improvement of the efficiency of catalysts and reactions of existing catalytic reactions will be made (Pathway A), as exemplified in many important catalytic processes developed over the years.3–11 Alternatively or ideally, further synthetic application of the isolated reactive organometallic intermediates, particularly when they are unprecedented ones, would lead to the development of completely new reactions and synthetic methods (Pathway B).
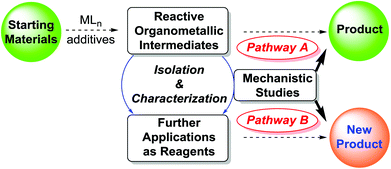 |
| Scheme 1 Organometallic intermediate-based organic synthesis: the strategy. | |
Metal-mediated stoichiometric reactions are widely applied in synthetic chemistry and chemical industry. Thus, in this perspective, we focus on metal-mediated stoichiometric reaction patterns to introduce the research strategy, using the isolated reactive organometallic intermediates as reagents (Scheme 1, Pathway B). The synthesis, structure and applications of organo-di-lithio reagents are highlighted. Transmetallation of these dilithium compounds leads to second-generation organometallic intermediates as reagents, which have also been applied to organic synthesis.
2. Reaction of dilithio reagents with CO: discovery of a new reaction, isolation of reactive organometallic intermediates, and further applications
Discovery of a new reaction
1,4-Dilithio-1,3-butadiene derivatives (2: dilithio reagents for short) shown in Scheme 2 have been known for a long time.12,13 Although some heterocycles (mainly metallacyclopentadienes) were prepared from dilithio reagents and metal salts,14,15 organic transformations and synthetic applications of dilithio reagents had been rarely investigated prior to our studies, which demonstrated unique reactivity and useful synthetic applications when treated with various organic substrates.16,17 In the early 2000s, we found that the reaction of dilithio reagents 2 with CO afforded trans-3-cyclopenten-1-ones 3 (Scheme 2).18 This reaction featured high efficiency and regio- and stereoselectivity, and was an unprecedented carbonylation of organolithium reagents.
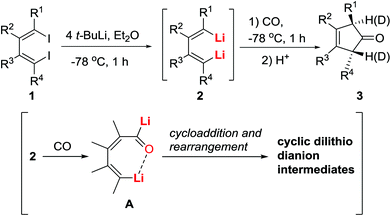 |
| Scheme 2
In situ generation of dilithio reagents 2 and their reaction with CO. | |
Initially, a reaction mechanism was proposed (Scheme 2) without the structural information of the dilithio intermediates 2 and their carbonylation reaction intermediates. This was based on the structures of the products 3 and the hypothesis that carbonylation of one C–Li bond in 2 would form the acyllithium intermediate A.19 It was also reasonable to propose an intramolecular stabilization of this entity by chelation. This intermediate A could undergo cycloaddition reaction/rearrangement to afford cyclic dilithio dianions. To shed light on the reaction pathway from the diiodo reagents, next we set out to isolate the reactive organometallic intermediates.
Isolation and characterization of dilithio reagents 2 and oxy-cyclopentadienyl dilithium intermediates 4
Attempts to isolate the intermediates formed between dilithio reagents 2 and CO directly from the reaction mixture generally failed, primarily due to the presence of a complicated reaction mixture, and the existence of LiI salts that prevented the isolation, purification, and crystallization of reactive intermediates. We, therefore, tried to establish a method for obtaining pure dilithio compounds 2 (Scheme 3) without the complication of LiI.13 The pure compound 2a was obtained in 65% isolated yield by extraction of 2a from the reaction mixture with hexane, filtration to remove LiI, and recrystallization in hexane.20 The X-ray structure determination indicated that 2a was a trimer with a Li6 pseudooctahedron.20a A variety of dilithio reagents 2 could be obtained as crystalline compounds on a gram-scale. They are stable as long as they are stored dry under N2 and can be directly used as reagents.
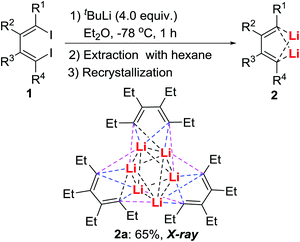 |
| Scheme 3 Isolation and characterization of pure organo-di-lithio reagents 2. | |
With pure isolated dilithio reagents 2 in hand, we then successfully isolated and characterized the reaction intermediate between the dilithio reagents 2 and CO. Thus, oxy-cyclopentadienyl dilithium intermediates 4 (OCp for short) were obtained (Scheme 4).21 X-ray structural analysis showed that it is a dimer in the solid state and two Cp rings are connected through a “Li2O2” four-membered ring. Each Cp ring is coordinated to a lithium atom in an η5-fashion.
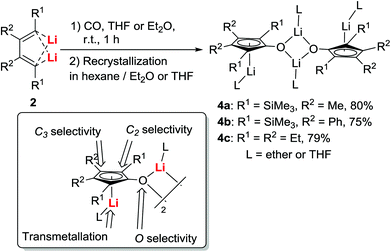 |
| Scheme 4 Isolation and characterization of dilithio OCp dianions 4. | |
These OCp dianions 4 are structurally unique (Scheme 4). There are two reactive sites: the CpLi moiety and the exocyclic OLi group. In addition, the CpLi moiety may have an issue of site-selectivity, e.g. C-2 or C-3 selectivity. Therefore, treatment of 4 with organic substrates or organometallic compounds might lead to interesting chemo- and regio-selectivity.21 Thus, the reaction chemistry and applications of the isolated intermediates 4 were subsequently investigated.
Application of oxy-cyclopentadienyl dilithium intermediates 4 (OCp dianions)
Since these OCp dianions 4 could be expected to act as precursors or ligands for transition metal complexes, we investigated the reactivity of dianions 4 with transition metal compounds. For example, treatment of 4 with Ni(dppe)Cl2 afforded the cyclopentadienone-nickel complexes 5 in good isolated yields (Scheme 5), demonstrating the unique utility of OCp dianions 4.21
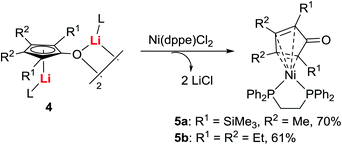 |
| Scheme 5 Application of OCp dianions 4: synthesis of η4-cyclopentadieneone-Ni(0) complexes 5. | |
It was also found that the reaction of 4 with electrophiles was dependent on the nature of electrophiles and the reaction conditions (Scheme 6).18,21 When 4 was treated with 2 equiv. of MeI, Me2SO4, benzyl halides, or propargyl halides, trans-3-C-2-alkylated cyclopentenone derivatives 6 were obtained with perfect chemo-, regio- and stereo-selectivity. When acid chlorides (RCOCl) were used, the reaction afforded double acylated cyclopentadienes 7a–c. When tBuCOCl/RCOCl was sequentially added to OCp dianions 4, mixed double acylated products such as 7d,e were obtained as the only products. These studies indicate that O-acylation is faster than C-acylation with tBuCOCl.
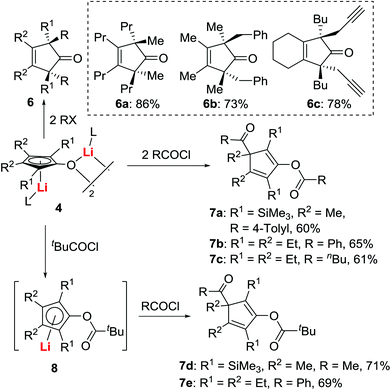 |
| Scheme 6 Site-selective reaction and synthetic applications of OCp dianions 4. | |
The high-yielding mono O-acylation intermediate 8 was especially interesting, since it might provide otherwise unavailable ester-substituted CpLi compounds.
Isolation and application of the monoacylated cyclopentadienyllithium intermediates 8
The O-acylated intermediate 8a was isolated successfully (Scheme 7).22 The structure of 8a was supported by X-ray structural analysis of its TMEDA-coordinated complex 9. Furthermore, it was found that the THF solvent triggered the silyl-shift of 8a to give the gem-bis(trimethylsilyl) complex 10, whose structure was also confirmed by X-ray structural analysis of the TMEDA-coordinated complex 11. Application of 8 for the synthesis of substituted metallocene type complexes is on-going research in our laboratory.23
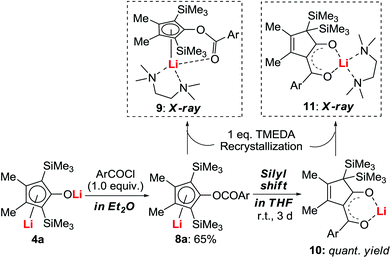 |
| Scheme 7 Isolation and reactivity of monoacylated intermediates 8. | |
3. Transmetallation of dilithio reagents to copper: discovery of new reactions, isolation of reactive organometallic intermediates, and further applications
Discovery of new reactions
The dilithio reagents 2 were expected to undergo transmetallation reactions to afford other organo-di-metallic or metallacyclic compounds.24 When dilithio reagents 2 were treated with CuCl, semibullvalene derivatives 12 were generated via a CuCl-promoted cyclodimerization (Scheme 8).25 This method provided the first practical synthesis of semibullvalenes using the metal-mediated C–C bond forming approach. Butadienyl dicopper intermediate was proposed as the first key intermediate upon transmetallation. When carbon monoxide was added, an unprecedented cycloaddition reaction was observed to afford head-to-head dimers 13 as well as the expected cyclopentadienones 14 (Scheme 8).26 It was also found that the proposed butadienyl dicopper intermediates underwent thermal decomposition–linear dimerization in the presence of additional LiI, providing octa-alkyl substituted all-cis octatetraene derivatives 15 following hydrolysis (Scheme 8).27
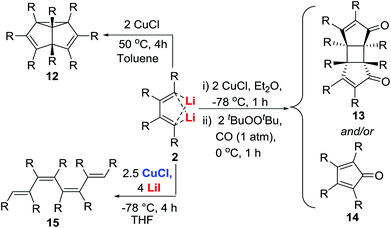 |
| Scheme 8 Transmetallation of dilithio reagents 2 to copper: new reactions. | |
Organocopper compounds are structurally interesting and synthetically useful. However, very few isolable and structurally well-defined alkenyl organocopper(I) compounds were known before our studies.28 The new reactions in Scheme 8 suggest the possibility of unique structures of the proposed butadienyl dicopper(I) intermediates.
Isolation of copper intermediates
Treatment of the dilithio reagent 2a′ (R = Pr) with 2.0 equiv. of CuCl in Et2O at −78 °C for 2 h afforded complex 16 (Scheme 9).29 The X-ray structural analysis of 16 revealed a tetrameric organo-dicopper aggregate. The four butadienyl moieties are linked to the copper cluster via two three-center–two-electron (3c–2e) bonds. The Cu–Cu stacking interaction stabilizes the organo-dicopper complex. In contrast, when 2a′ was treated with 2.5 equiv. of CuCl and 4.0 equiv. of LiI in THF, 1,8-dicopper-1,3,5,7-octatetraene 17 was obtained.29 The solid state structure shows three copper atoms forming a linear Cu–Cu–Cu geometry. Each terminal carbon is linked to two copper atoms forming three-center–two-electron (3c–2e) bonds.
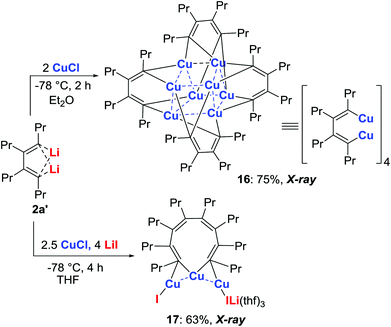 |
| Scheme 9 Isolation and characterization of 1,4-dicopper 16 and 1,8-dicopper 17. | |
Application of the copper intermediates
The reactivity of isolated copper intermediates 16 and 17 was explored next. Intramolecular homo-coupling of compounds 16 and subsequent [4 + 2] cycloaddition gave tricycle[4.2.0.02,5]octa-3,7-dienes 18 (Scheme 10).29 In the presence of LiI, compound 16 reacted with 2-methylphenyl isocyanide to give the iminocyclopentadiene 19via 1,1-insertion/intramolecular coupling. When the aggregate 17 was dissolved in THF (Scheme 10), an intramolecular C–C coupling reaction afforded the cyclooctatetraene 20. Interestingly, when the solvent was switched from THF to toluene, the semibullvalene derivative 21 was obtained.25,29
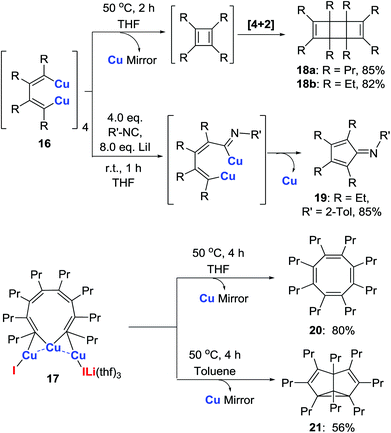 |
| Scheme 10 Applications of isolated copper aggregates. | |
Since these organocopper(I) compounds are structurally very interesting, the Cu–Cu interaction might result in novel applications when treated with organic substrates and small molecules in particular. Further applications are currently under investigation.
4. Synthesis, structural characterization, and synthetic application of reactive organometallic intermediates
The above research has generally followed the strategy of organometallic intermediate-based organic synthesis, in the order of discovery of new reactions, isolation of reactive organometallic intermediates, and further applications of these organometallic intermediates to new processes. In addition, dilithio reagents have also been directly applied to the synthesis of different organometallic species to obtain opportunities to develop novel chemistry and synthetic methods. Two recent examples from our group are presented below.
Synthesis, characterization and application of magnesiacyclopentadienes
Metallocyclopentadienes are a class of important organometallic compounds because they are very useful building blocks for synthetic chemistry, and are often proposed as key intermediates in metal-mediated or catalyzed reactions. However, metallocyclopentadienes of the alkaline-earth metals were not known until our study. When the dilithio reagent 2b (R1 = TMS, R2 = Me) was treated with one equiv. of MgCl2 in Et2O at room temperature for 12 h, the magnesiacyclopentadiene 22 was formed cleanly (Scheme 11).30 The structure of 22 was confirmed by X-ray structural analysis of its TMEDA-coordinated complex 23. Being nearly coplanar, the angle of C1–Mg–C4 (91.54°) is much smaller than the classic C–Mg–C angles in usual tetracoordinate magnesium compounds (115–130°). These magnesiacyclopentadienes showed excellent reactivity towards thioformamides, which provided an efficient synthetic method for the preparation of amino cyclopentadienes 24. In comparison, when the pure dilithio reagents 2 were treated with thioformamides, a mixture of unidentified products and amino cyclopentadienes was observed. This result demonstrated the unique reactivity of magnesiacyclopentadienes.
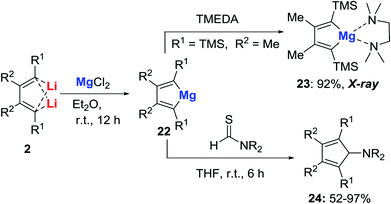 |
| Scheme 11 Synthesis, characterization and application of magnesiacyclopentadienes 22. | |
Synthesis, characterization and application of barium dibenzopentalenides
The first main-group metal η8 complex coordinated with a dibenzopentalene dianion was recently synthesized and structurally characterized by our group.31 The barium dibenzopentalenides 25 (Scheme 12), in which the single barium atom is coordinated to the parent pentalene in an η8 fashion as revealed by their X-ray structural analysis, were synthesized from 2,3-diphenyl 1,4-dilithio-1,3-butadienes and Ba[N(SiMe3)2]2via formation of Ba–C (sp2) bonds. Preliminary reaction chemistry of barium dibenzopentalenides 25 has confirmed that synthetically useful applications can be expected. As demonstrated in Scheme 12,31 dibenzopentalene derivatives, which have attracted considerable attention recently because of their unique planar structures and anti-aromatic character, could be obtained efficiently via further reactions of the isolated barium dibenzopentalenides 25.
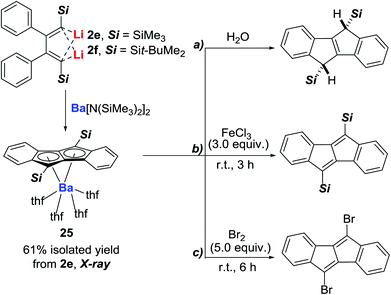 |
| Scheme 12 Synthesis, characterization and application of barium dibenzopentalenides 25. | |
5. Some other examples involving structurally characterized organometallic intermediates
Other representative examples including transition-metal or rare earth metal-mediated or catalyzed reactions, in which reactive organometallic intermediates are isolated and structurally characterized, are demonstrated in Schemes 13 and 14. The preparation and reaction chemistry of metallacyclic compounds have attracted much attention in recent years. In particular, a number of zirconacycles have been isolated and structurally characterized. One-pot generation of zirconacycles and sequential reactions with various substrates have been applied for organic synthesis (Scheme 13).32
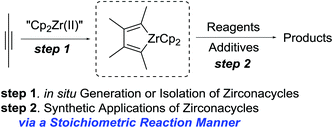 |
| Scheme 13
In situ generation and synthetic applications of zirconacyclopentadiene derivatives. | |
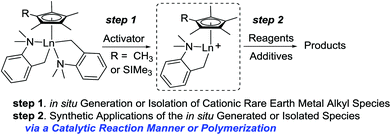 |
| Scheme 14 Synthetic applications of in situ generated or isolated cationic rare earth metal alkyl species. | |
Cationic half-sandwich rare earth metal alkyl complexes are of much current interest and regarded as a class of highly reactive intermediates. Well-defined cationic half-sandwich rare earth metal alkyl complexes remain scarce because of facile ligand scrambling and other decomposition reactions. As illustrated in Scheme 14, the reaction of the dialkyl complexes with activators yields the corresponding cationic monoalkyl species. These either in situ generated or isolated cationic monoalkyl species exhibit excellent catalytic activity for organic transformations, including polymerization (Scheme 14).33
6. Concluding remarks
In this Perspective, the above-introduced research strategy on organometallic intermediate-based organic synthesis is centered on isolation and structural characterization of reactive organometallic intermediates, which usually require more time and effort than the discovery of the reaction itself and are considerably more challenging experimentally. The isolation and study of reactive organometallic intermediates not only lead to a better understanding of their reactivity and reaction mechanisms, but can also guide the discovery of new reactions, culminating in the advancement of novel synthetic methods.
We hope this manuscript has demonstrated a useful bridge between organometallic chemistry and synthetic organic chemistry. Filling this gap should benefit both areas and advance the development of synthetic chemistry as a whole. Furthermore, we hope this manuscript will encourage more researchers to develop a deeper interest in the chemical nature of metal-mediated or catalyzed reactions, and will convince, to a certain extent, funding agencies to better support such fundamental and widely applied research areas.
Acknowledgements
This work was supported by the 973 Program (2011CB808700) and the Natural Science Foundation of China (NSFC).
References
-
(a)
Organotransition Metal Chemistry: From Bonding to Catalysis, ed. J. F. Hartwig, University Science Books, Mill Valley, California, 2010 Search PubMed;
(b)
Comprehensive Organometallic Chemistry III, ed. R. H. Crabtree and D. M. P. Mingos, Elsevier, Oxford, 2006 Search PubMed.
-
(a) I. Marek, Y. Minko, M. Pasco, T. Mejuch, N. Gilboa, H. Chechik and J. P. Das, J. Am. Chem. Soc., 2014, 136, 2682 CrossRef CAS PubMed;
(b) Z. Qiu and Z. Xie, Dalton Trans., 2014, 43, 4925 RSC;
(c) A. S. K. Hashmi, Acc. Chem. Res., 2014, 47, 864 CrossRef CAS PubMed;
(d) A. Quintard and J. Rodriguez, Angew. Chem., Int. Ed., 2014, 53, 4044 CrossRef CAS PubMed;
(e) K. M. Engle, T.-S. Mei, M. Wasa and J.-Q. Yu, Acc. Chem. Res., 2012, 45, 788 CrossRef CAS PubMed;
(f) W.-X. Zhang, S. Zhang and Z. Xi, Acc. Chem. Res., 2011, 44, 541 CrossRef CAS PubMed;
(g) J. M. Racowski and M. S. Sanford, Top. Organomet. Chem., 2011, 35, 61 CrossRef CAS;
(h) C. Coperet, Chem. Rev., 2010, 110, 656 CrossRef CAS PubMed;
(i) V. Capriati, S. Florio and R. Luisi, Chem. Rev., 2008, 108, 1918 CrossRef CAS PubMed;
(j) R. Chinchilla, C. Najera and M. Yus, Chem. Rev., 2004, 104, 2667 CrossRef CAS PubMed;
(k) J. A. Varela and C. Saá, Chem. Rev., 2003, 103, 3787 CrossRef CAS PubMed;
(l) W. D. Jones, Acc. Chem. Res., 2003, 36, 140 CrossRef CAS PubMed;
(m) J. R. Fulton, A. W. Holland, D. J. Fox and R. G. Bergman, Acc. Chem. Res., 2002, 35, 44 CrossRef CAS PubMed;
(n) A. P. Pape, K. P. Kaliappan and E. P. Kundig, Chem. Rev., 2000, 100, 2917 CrossRef CAS PubMed.
-
(a) J. Halpern, Pure Appl. Chem., 1986, 58, 575 CrossRef CAS;
(b) J. Halpern, J. F. Harrod and B. R. James, J. Am. Chem. Soc., 1966, 88, 5150 CrossRef CAS.
-
(a) S. M. Ahmed, A. Poater, M. I. Childers, P. C. B. Widger, A. M. Lapointe, E. B. Lobkovsky, G. W. Coates and L. Cavallo, J. Am. Chem. Soc., 2013, 135, 18901 CrossRef CAS PubMed;
(b) G. W. Coates, P. D. Hustad and S. Reinartz, Angew. Chem., Int. Ed., 2002, 41, 2236 CrossRef CAS.
-
(a) G. C. Vougioukalakis and R. H. Grubbs, Chem. Rev., 2010, 110, 1746 CrossRef CAS PubMed;
(b) Y. Chauvin, Angew. Chem., Int. Ed., 2006, 45, 3740 CrossRef PubMed;
(c) R. R. Schrock, Angew. Chem., Int. Ed., 2006, 45, 3748 CrossRef CAS PubMed;
(d) R. H. Grubbs, Angew. Chem., Int. Ed., 2006, 45, 3760 CrossRef CAS PubMed.
- J. F. Hartwig and L. M. Stanley, Acc. Chem. Res., 2010, 43, 1461 CrossRef CAS PubMed.
-
(a) C. Li, T. Yano, N. Ishida and M. Murakami, Angew. Chem., Int. Ed., 2013, 52, 9801 CrossRef CAS PubMed;
(b) K. Sasano, J. Takaya and N. Iwasawa, J. Am. Chem. Soc., 2013, 135, 10954 CrossRef CAS PubMed;
(c) A. Maleckis, J. W. Kampf and M. S. Sanford, J. Am. Chem. Soc., 2013, 135, 6618 CrossRef CAS PubMed;
(d) D. Katayev, Y.-X. Jia, A. K. Sharma, D. Banerjee, C. Besnard, R. B. Sunoj and E. P. Kündig, Chem. – Eur. J., 2013, 19, 11916 CrossRef CAS PubMed;
(e) Y. Li, X.-S. Zhang, H. Li, W.-H. Wang, K. Chen, B.-J. Li and Z.-J. Shi, Chem. Sci., 2012, 3, 1634 RSC;
(f) R. Giri, Y. Lan, P. Liu, K. N. Houk and J.-Q. Yu, J. Am. Chem. Soc., 2012, 134, 14118 CrossRef CAS PubMed;
(g) B. Xiao, T.-J. Gong, J. Xu, Z.-J. Liu and L. Liu, J. Am. Chem. Soc., 2011, 133, 1466 CrossRef CAS PubMed;
(h) M. E. Evans, T. Li and W. D. Jones, J. Am. Chem. Soc., 2010, 132, 16278 CrossRef CAS PubMed;
(i) D. C. Powers, M. A. L. Geibel, J. E. M. N. Klein and T. Ritter, J. Am. Chem. Soc., 2009, 131, 17050 CrossRef CAS PubMed;
(j) D. A. Culkin and J. F. Hartwig, J. Am. Chem. Soc., 2002, 124, 9330 CrossRef CAS PubMed.
-
(a) X. Wang, P. Guo, Z. Han, X. Wang, Z. Wang and K. Ding, J. Am. Chem. Soc., 2014, 136, 405 CrossRef CAS PubMed;
(b) W.-B. Liu, C. Zheng, C.-X. Zhuo, L.-X. Dai and S.-L. You, J. Am. Chem. Soc., 2012, 134, 4812 CrossRef CAS PubMed;
(c) S. Fan, F. Chen and X. Zhang, Angew. Chem., Int. Ed., 2011, 50, 5918 CrossRef CAS PubMed.
-
(a) Y. Tang, J. Li, Y. Zhu, Y. Li and B. Yu, J. Am. Chem. Soc., 2013, 135, 18396 CrossRef CAS PubMed;
(b) J. Cornella, E. Gómez-Bengoa and R. Martin, J. Am. Chem. Soc., 2013, 135, 1997 CrossRef CAS PubMed;
(c) J. F. Hooper, A. B. Chaplin, C. González-Rodríguez, A. L. Thompson, A. S. Weller and M. C. Willis, J. Am. Chem. Soc., 2012, 134, 2906 CrossRef CAS PubMed;
(d) T. Koreeda, T. Kochi and F. Kakiuchi, J. Am. Chem. Soc., 2009, 131, 7238 CrossRef CAS PubMed.
-
(a) A. D. Schwarz, C. S. Onn and P. Mountford, Angew. Chem., Int. Ed., 2012, 51, 12298 CrossRef CAS PubMed;
(b) D. C. Leitch, C. S. Turner and L. L. Schafer, Angew. Chem., Int. Ed., 2010, 49, 6382 CrossRef CAS PubMed.
-
(a) M. A. Düfert, K. L. Billingsley and S. L. Buchwald, J. Am. Chem. Soc., 2013, 135, 12877 CrossRef PubMed;
(b) M. M. Hussain, H. M. Li, N. Hussain, M. Urena, P. J. Carroll and P. J. Walsh, J. Am. Chem. Soc., 2009, 131, 6516 CrossRef CAS PubMed;
(c) J. L. Paih, F. Monnier, S. Derien, P. H. Dixneuf, E. Clot and O. Eisenstein, J. Am. Chem. Soc., 2003, 125, 11964 CrossRef PubMed;
(d) J. H. Kirchhoff, M. R. Netherton, I. D. Hills and G. C. Fu, J. Am. Chem. Soc., 2002, 124, 13662 CrossRef CAS PubMed.
- D. Seyferth, Organometallics, 2009, 28, 2 CrossRef CAS.
-
(a) A. J. Kos and P. v. R. Schleyer, J. Am. Chem. Soc., 1980, 102, 7928 CrossRef CAS;
(b) U. Schubert, W. Neugebauer and P. v. R. Schleyer, J. Chem. Soc., Chem. Commun., 1982, 1184 RSC;
(c) W. Bauer, M. Feigel, G. Müller and P. v. R. Schleyer, J. Am. Chem. Soc., 1988, 110, 6033 CrossRef CAS PubMed;
(d) F. Pauer and P. P. Power, J. Organomet. Chem., 1994, 474, 27 CrossRef CAS;
(e) A. J. Ashe III, J. W. Kampf and P. M. Savla, Organometallics, 1993, 12, 3350 CrossRef;
(f) M. Saito, M. Nakamura, T. Tajima and M. Yoshioka, Angew. Chem., Int. Ed., 2007, 46, 1504 CrossRef CAS PubMed.
-
(a) V. P. Ananikov, O. V. Hazipov and I. P. Beletskaya, Chem. – Asian J., 2011, 6, 306 CrossRef CAS PubMed;
(b) J. Dubac, A. Laporterie and G. Manuel, Chem. Rev., 1990, 90, 215 CrossRef CAS.
-
(a) F. Foubelo and M. Yus, Curr. Org. Chem., 2005, 9, 459 CrossRef CAS;
(b) P. Langer and W. Freiberg, Chem. Rev., 2004, 104, 4125 CrossRef CAS PubMed.
- Z. Xi, Acc. Chem. Res., 2010, 43, 1342 CrossRef CAS PubMed.
-
(a) Z. Xi and Q. Song, J. Org. Chem., 2000, 65, 9157 CrossRef CAS PubMed;
(b) Z. Xi, Q. Song, J. Chen, H. Guan and P. Li, Angew. Chem., Int. Ed., 2001, 40, 1913 CrossRef CAS;
(c) Q. Song, Z. Li, J. Chen, C. Y. Wang and Z. Xi, Org. Lett., 2002, 4, 4627 CrossRef CAS PubMed;
(d) J. Chen, Q. Song, C. Y. Wang and Z. Xi, J. Am. Chem. Soc., 2002, 124, 6238 CrossRef CAS PubMed;
(e) C. Wang, Q. Luo, H. Sun, X. Guo and Z. Xi, J. Am. Chem. Soc., 2007, 129, 3094 CrossRef CAS PubMed;
(f) N. Yu, C. Y. Wang, F. Zhao, W.-X. Zhang and Z. Xi, Chem. – Eur. J., 2008, 14, 5670 CrossRef CAS PubMed.
- Q. Song, J. Chen, X. Jin and Z. Xi, J. Am. Chem. Soc., 2001, 123, 10419 CrossRef CAS.
- D. Seyferth, Isr. J. Chem., 1984, 24, 167 CrossRef CAS.
-
(a) L. Liu, W.-X. Zhang, Q. Luo, H. Li and Z. Xi, Organometallics, 2010, 29, 278 CrossRef CAS;
(b) S. Zhang, M. Zhan, Q. Luo, W.-X. Zhang and Z. Xi, Organometallics, 2013, 32, 4020 CrossRef CAS.
- L. Liu, W.-X. Zhang, C. Wang, C. Y. Wang and Z. Xi, Angew. Chem., Int. Ed., 2009, 48, 8111 CrossRef CAS PubMed.
- H. Li, L. Liu, Z. Wang, F. Zhao, S. Zhang, W.-X. Zhang and Z. Xi, Chem. – Eur. J., 2011, 17, 7399 CrossRef CAS PubMed.
- H. Li, W.-X. Zhang and Z. Xi, Chem. – Eur. J., 2013, 19, 12859 CrossRef CAS PubMed.
-
(a) Y. Zhou, W.-X. Zhang and Z. Xi, Organometallics, 2012, 31, 5546 CrossRef CAS;
(b) H. Fang, G. Li, G. Mao and Z. Xi, Chem. – Eur. J., 2004, 10, 3444 CrossRef CAS PubMed.
-
(a) C. Wang, J. Yuan, G. Li, Z. Wang, S. Zhang and Z. Xi, J. Am. Chem. Soc., 2006, 128, 4564 CrossRef CAS PubMed;
(b) S. Zhang, M. Zhan, Q. Wang, C. Wang, W.-X. Zhang and Z. Xi, Org. Chem. Front., 2014, 1, 130 RSC.
- Q. Luo, C. Wang, W.-X. Zhang and Z. Xi, Chem. Commun., 2008, 1593 RSC.
- J. Wei, Z. Wang, W.-X. Zhang and Z. Xi, Org. Lett., 2013, 15, 1222 CrossRef CAS PubMed.
-
(a) G. van Koten, P. J. Pérez and L. Liebeskind, Organometallics, 2012, 31, 7631 CrossRef CAS;
(b)
Z. Pappoport and I. Marek, The Chemistry of Organocopper Compounds, Wiley-VCH, Weinheim, Germany, 2009 Search PubMed;
(c) B. H. Lipshutz and Y. Yamamoto, Chem. Rev., 2008, 108, 2793 CrossRef CAS PubMed.
- W. Geng, J. Wei, W.-X. Zhang and Z. Xi, J. Am. Chem. Soc., 2014, 136, 610 CrossRef CAS PubMed.
-
(a) J. Wei, L. Liu, M. Zhan, L. Xu, W.-X. Zhang and Z. Xi, Angew. Chem., Int. Ed., 2014, 53, 5634 CrossRef CAS PubMed. See also:
(b) J. Wei, Y. Zhang, W.-X. Zhang and Z. Xi, Org. Chem. Front., 2014, 1, 983 RSC.
-
(a) H. Li, B. Wei, L. Xu, W.-X. Zhang and Z. Xi, Angew. Chem., Int. Ed., 2013, 52, 10822 CrossRef CAS PubMed. See also:
(b) H. Li, X.-Y. Wang, B. Wei, L. Xu, W.-X. Zhang, J. Pei and Z. Xi, Nat. Commun., 2014, 5, 4508 Search PubMed.
-
(a)
T. Takahashi and Y. Li, in Titanium and Zirconium in Organic Synthesis, ed. I. Marek, Wiley-VCH, Weinhein, Germany, 2002, pp. 50–85 Search PubMed;
(b) G. Erker, G. Kehr and R. Fröhlich, J. Organomet. Chem., 2004, 689, 4305 CrossRef CAS PubMed;
(c) E. Negishi, Dalton Trans., 2005, 827 RSC;
(d) U. Rosenthal, V. V. Burlakov, M. A. Bach and T. Beweries, Chem. Soc. Rev., 2007, 36, 719 RSC;
(e) T. Beweries, M. Haehnel and U. Rosenthal, Catal. Sci. Technol., 2013, 3, 18 RSC.
-
(a) J. Oyamada and Z. Hou, Angew. Chem., Int. Ed., 2012, 51, 12828 CrossRef CAS PubMed;
(b) M. Nishiura and Z. Hou, Nat. Chem., 2010, 2, 257 CrossRef CAS PubMed;
(c) M. Nishiura and Z. Hou, Bull. Chem. Soc. Jpn., 2010, 83, 595 CrossRef CAS;
(d) M. Takimoto, S. Usami and Z. Hou, J. Am. Chem. Soc., 2009, 131, 18266 CrossRef CAS PubMed.
Footnote |
† Dedicated to Prof. Ei-ichi Negishi on the occasion of his 80th birthday. |
|
This journal is © the Partner Organisations 2014 |