DOI:
10.1039/C4QO00209A
(Research Article)
Org. Chem. Front., 2014,
1, 1045-1049
Generation of 1-amino-isoquinoline-N-oxides via a tandem reaction of 2-alkynylbenzaldoxime with secondary amines in the presence of silver(I) and copper(I)†
Received
26th July 2014
, Accepted 19th August 2014
First published on 20th August 2014
Abstract
1-Amino-isoquinoline-N-oxides are generated under mild conditions through a tandem reaction of 2-alkynylbenzaldoxime with secondary amines in the presence of silver(I) and copper(I). The reaction proceeds smoothly at room temperature under air, leading to the corresponding products in good yields. During the reaction process, a silver-catalyzed 6-endo cyclization and a copper(I)-catalyzed C–H bond activation are involved.
1. Introduction
In the past decade, the achievement of the diversity-oriented synthesis of natural product-like compounds with privileged scaffolds has been witnessed.1 A range of N-heterocycles have been applied broadly in the drug discovery process. We have also been involved in the construction of libraries of small molecules with privileged scaffolds.2 Some hits have been identified during the subsequent biological assays. For instance, 2-alkynylbenzaldoxime as a versatile building block has been applied widely for the construction of N-heterocycles.3 Since it can be easily transferred to isoquinoline N-oxide via intramolecular 6-endo cyclization in the presence of metal catalysts or electrophiles,4 the subsequent [3 + 2] cycloaddition/nucleophilic addition and rearrangement could be expected. On the other hand, a Beckmann rearrangement could happen first since an oxime moiety is present in the molecule, which would then undergo an intramolecular cyclization to furnish nitrogen-containing heterocycles.5 Therefore, 2-alkynylbenzaldoxime is recognized as a good candidate for the generation of libraries of small molecules. In most cases, the chemistry is related to isoquinoline N-oxide, which can be isolated and obtained from 2-alkynylbenzaldoxime via 6-endo cyclization. A large collection of small molecules would be produced if diverse isoquinoline N-oxides could be easily accessible.
Recently, the transition metal catalyzed direct C–H oxidative amination of arenes has attracted much attention.6 For instance, Wu and Cui reported a copper(I)-catalyzed reaction of quinoline-N-oxide with amines.6a During the process, the C–H bond at the ortho-position of the N-oxide was activated, thus undergoing subsequent amination. Encouraged by this result, we envisioned that the C–H bond at the ortho-position of isoquinoline N-oxide could be activated as well, which would react with amines to provide diverse 1-amino-isoquinoline-N-oxides. The proposed synthetic route is present in Scheme 1. We conceived that in the presence of silver(I) and copper(I) salts as co-catalysts, the reaction of 2-alkynylbenzaldoxime 1 with amines 2 would produce the corresponding 1-amino-isoquinoline-N-oxides. During the transformation, a silver(I)-catalyzed 6-endo cyclization would occur first, to afford isoquinoline N-oxide A. Subsequently, a C–H bond activation would take place in the presence of copper(I) salt to form intermediate B. If the reaction was performed under air atmosphere, the oxygen would act as an oxidant to involve amines in the transformation, leading to intermediate C with the release of a molecule of water. The following reductive elimination will deliver 1-amino-isoquinoline-N-oxides 3. With this consideration in mind, we thus initiated a program for the generation of 1-amino-isoquinoline-N-oxides.
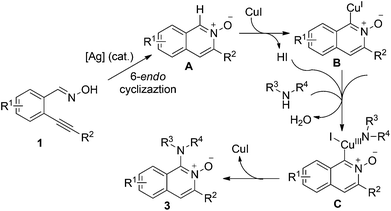 |
| Scheme 1 A proposed synthetic route to 1-amino-isoquinoline-N-oxides. | |
2. Results and discussion
At the outset, the studies were initially performed for a model reaction of 2-alkynylbenzaldoxime 1a with piperidine 2a at 50 °C in toluene under air atmosphere (Table 1). A control experiment catalysed by 10 mol% of silver triflate without the addition of copper(I) salt indicated that no desired product 3a was formed (Table 1, entry 1). Only isoquinoline-N-oxide A1 was isolated. This result demonstrated the important role of the copper(I) catalyst in the reaction process for the activation of the C–H bond at the ortho-position of isoquinoline N-oxide. As expected, 1-amino-isoquinoline-N-oxide 3a was obtained in 87% yield when Cu2O (5 mol%) was added to the reaction system (Table 1, entry 2). The structure of 3a was confirmed by X-ray crystallographic analysis (Fig. 1). A similar result was observed when the copper salt was changed to CuCl or CuBr (Table 1, entries 3 and 4). The reaction co-catalyzed by silver triflate and palladium acetate gave rise to the expected product 3a in 17% yield (data not shown in Table 1). Gratifyingly, the corresponding product 3a was furnished in 95% yield when copper(I) iodide was used as the catalyst (Table 1, entry 5). No better yields were isolated when other solvents were utilized (Table 1, entries 6–10). The efficiency was not affected when the reaction occurred at room temperature (Table 1, entry 11). The same result was observed when the reaction was performed at 80 °C (Table 1, entry 12).
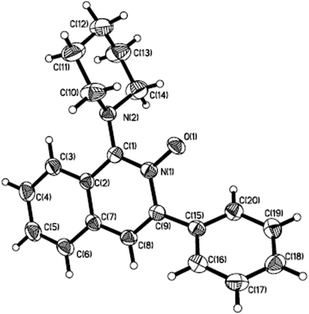 |
| Fig. 1 X-ray ORTEP illustration of compound 3a. | |
Table 1 Initial studies for the reaction of 2-alkynylbenzaldoxime 1a with piperidine 2a
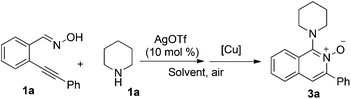
|
Entry |
[Cu] |
Temp (°C) |
Solvent |
Yielda (%) |
Isolated yield based on 2-alkynylbenzaldoxime 1a.
In the absence of AgOTf.
|
1 |
— |
50 |
Toluene |
nr |
2 |
Cu2O |
50 |
Toluene |
87 |
3 |
CuCl |
50 |
Toluene |
85 |
4 |
CuBr |
50 |
Toluene |
88 |
5 |
CuI |
50 |
Toluene |
95 |
6 |
CuI |
50 |
DCE |
82 |
7 |
CuI |
50 |
MeCN |
79 |
8 |
CuI |
50 |
1,4-Dioxane |
83 |
9 |
CuI |
50 |
THF |
80 |
10 |
CuI |
50 |
DMA |
76 |
11 |
CuI |
25 |
Toluene |
96 |
12 |
CuI |
80 |
Toluene |
95 |
13b |
CuI |
25 |
Toluene |
21 |
The above mild optimized conditions prompted us to explore the scope of this silver(I) and copper(I) co-catalyzed reaction of 2-alkynylbenzaldoximes 1 with amines 2. The results are summarized in Tables 2 and 3. At the beginning, reactions of 2-alkynylbenzaldoximes 1 with piperidine 2a were examined (Table 2). It was found that in most cases, the reactions worked well to afford the expected products 3 in good to excellent yields. 2-Alkynylbenzaldoximes 1 with different groups attached on the aromatic ring were all good reactants under the standard conditions. Reactions of 2-alkynylbenzaldoximes 1 with aryl groups attached on the triple bond (R2) proceeded smoothly, leading to the corresponding products in good yields. Inferior results were obtained for the reaction of 2-alkynylbenzaldoximes 1 with alkyl groups attached on the triple bond (compounds 3e and 3f).
Table 2 Synthesis of 1-amino-isoquinoline-N-oxides 3 through a silver(I) and copper(I) co-catalyzed reaction of 2-alkynylbenzaldoxime 1 with piperidine 2aa
Isolated yield based on 2-alkynylbenzaldoxime 1.
|
|
Table 3 Synthesis of 1-amino-isoquinoline-N-oxides 3 through a silver(I) and copper(I) co-catalyzed reaction of 2-alkynylbenzaldoxime 1a with secondary amines 2a
Isolated yield based on 2-alkynylbenzaldoxime 1a.
|
|
We next investigated the silver(I) and copper(I) co-catalyzed reaction of 2-alkynylbenzaldoxime 1a with various amines (Table 3). Not only pyrrolidine but also azepane was a good partner in this transformation. The reaction of 2-alkynylbenzaldoxime 1a with morpholine gave rise to the expected product 3n in 93% yield. However, reaction of 2-alkynylbenzaldoxime 1a with 2,2,6,6-tetramethylpiperidine did not afford the desired product 3o. From these results, it was shown that the outcome was affected by the steric hindrance of the substrates. Other acyclic amines such as diethyl amine and dihexyl amine were employed in the reaction, which afforded a trace amount of the desired products. Moreover, primary amines are also not effective under these conditions (data not shown in Table 3), and no expected products 3 were detected when primary amines were used as reaction partners under these conditions.
3. Conclusion
In conclusion, we have reported the synthesis of 1-amino-isoquinoline-N-oxides through a silver(I) and copper(I) co-catalyzed reaction of 2-alkynylbenzaldoxime with secondary amines under mild conditions. The transformation proceeds smoothly at room temperature under air, leading to the corresponding products in good yields. During the reaction process, a silver-catalyzed 6-endo cyclization and a copper(I)-catalyzed C–H bond activation are involved. The reaction scope has been demonstrated and a range of 1-amino-isoquinoline-N-oxides is produced efficiently. Currently, application of the 1-amino-isoquinoline-N-oxides for the construction of diverse N-heterocycles is ongoing in our laboratory.
4. Experimental section
General experimental procedure for the synthesis of 1-amino-isoquinoline-N-oxides 3 through a silver(I) and copper(I) co-catalyzed reaction of 2-alkynylbenzaldoximes 1 with amines 2: a mixture of silver triflate (0.02 mmol, 5.1 mg) and 2-alkynylbenzaldoxime 1 (0.2 mmol) in toluene (1.0 mL) was stirred at 70 °C for 1 hour. Then secondary amine 2 (1.6 mmol), CuI (0.01 mmol, 1.9 mg), and toluene (1.0 mL) were added subsequently. The reaction was stirred at 25 °C until completion of the reaction as indicated by TLC. The solvent was evaporated and the residue was purified by column chromatography on silica gel to provide the product 3.
3-Phenyl-1-(trifluoromethyl)isoquinoline 3a
1H NMR (400 MHz, CDCl3) δ 8.19 (d, J = 8.2 Hz, 1H), 7.75–7.70 (m, 3H), 7.61–7.49 (m, 3H), 7.49–7.38 (m, 3H), 3.79 (s, 2H), 3.17 (s, 2H), 1.83–1.75 (m, 6H); 13C NMR (100 MHz, CDCl3) δ 151.1, 147.5, 133.8, 130.2, 129.9, 128.9, 128.2, 128.0, 127.5, 127.3, 126.9, 124.2, 120.8, 49.7, 26.6, 24.4; HRMS (ESI) calcd for C20H20N2O: 305.1648 (M + H+), found: 305.166.
5-Methoxy-3-phenyl-1-(piperidin-1-yl)isoquinoline 2-oxide 3b
1H NMR (400 MHz, CDCl3) δ 7.94 (s, 1H), 7.77–7.74 (m, 3H), 7.48–7.38 (m, 4H), 6.86 (d, J = 7.7 Hz, 1H), 3.97 (s, 3H), 3.72 (s, 2H), 3.16 (s, 2H), 1.79–1.75 (m, 6H); 13C NMR (100 MHz, CDCl3) δ 155.2, 150.9, 146.6, 134.2, 130.9, 129.9, 128.7, 128.6, 127.9, 122.3, 116.2, 115.6, 106.4, 55.7, 49.6, 26.5, 24.4; HRMS (ESI) calcd for C21H22N2O2: 335.1754 (M + H+), found: 335.1758.
7-Chloro-3-phenyl-1-(piperidin-1-yl)isoquinoline 2-oxide 3c
1H NMR (400 MHz, CDCl3) δ 8.15 (s, 1H), 7.72 (dd, J = 8.0, 1.6 Hz, 2H), 7.65 (d, J = 8.4 Hz, 1H), 7.51 (s, 1H), 7.48–7.40 (m, 4H), 3.80 (s, 2H), 3.09 (s, 2H), 1.85–1.75 (m, 6H); 13C NMR (100 MHz, CDCl3) δ 150.5, 147.9, 134.3, 133.4, 130.9, 129.7, 129.1, 128.9, 128.5, 128.2, 128.1, 123.0, 120.6, 49.3, 26.5, 24.3; HRMS (ESI) calcd for C20H19ClN2O: 339.1259 (M + H+), found: 339.1256.
7-Fluoro-3-phenyl-1-(piperidin-1-yl)isoquinoline 2-oxide 3d
1H NMR (400 MHz, CDCl3) δ 7.81 (dd, J = 10.3, 2.0 Hz, 1H), 7.73–7.70 (m, 3H), 7.54 (s, 1H), 7.48–7.40 (m, 3H), 7.31–7.26 (m, 1H), 3.78 (s, 2H), 3.08 (s, 2H), 1.84–1.75 (m, 6H); 13C NMR (100 MHz, CDCl3) δ 162.2 (d, 1JCF = 247.4 Hz), 150.7, 147.1, 133.5, 130.9, 129.8, 129.0, 128.8, 128.1, 127.0, 120.7, 118.2 (d, 2JCF = 25.4 Hz), 108.1 (d, 2JCF = 24.0 Hz), 49.2, 26.5, 24.3; HRMS (ESI) calcd for C20H19FN2O: 323.1554 (M + H+), found: 323.1561.
3-Butyl-1-(piperidin-1-yl)isoquinoline 2-oxide 3e
1H NMR (400 MHz, CDCl3) δ 8.14–8.12 (m, 1H), 7.66–7.64 (m, 1H), 7.50–7.48 (m, 2H), 7.33 (s, 1H), 3.75 (s, 2H), 3.10 (s, 1H), 3.03–2.95 (m, 2H), 2.17 (s, 1H), 1.78–1.72 (m, 8H), 1.54–1.45 (m, 2H), 0.99 (t, J = 7.3 Hz, 3H); 13C NMR (100 MHz, CDCl3) δ 150.8, 149.8, 130.2, 128.0, 127.3, 126.2, 124.1, 117.9, 49.6, 30.4, 29.2, 26.6, 24.3, 22.7, 13.9; HRMS (ESI) calcd for C18H24N2O: 285.1961 (M + H+), found: 285.1952.
3-(tert-Butyl)-1-(piperidin-1-yl)isoquinoline 2-oxide 3f
1H NMR (400 MHz, CDCl3) δ 8.11 (d, J = 8.1 Hz, 1H), 7.66 (d, J = 7.7 Hz, 1H), 7.51–7.43 (m, 3H), 3.63 (s, 2H), 3.11 (s, 2H), 1.84–1.75 (m, 6H), 1.59 (s, 9H); 13C NMR (100 MHz, CDCl3) δ 155.5, 151.9, 129.6, 127.5, 126.7, 123.5, 117.2, 49.1, 36.6, 28.3, 26.5, 24.3; HRMS (ESI) calcd for C18H24N2O: 285.1961 (M + H+), found: 285.1941.
7-Methyl-3-phenyl-1-(piperidin-1-yl)isoquinoline 2-oxide 3g
1H NMR (400 MHz, CDCl3) δ 7.95 (s, 1H), 7.74 (d, J = 6.7 Hz, 2H), 7.62 (d, J = 7.9 Hz, 1H), 7.48–7.40 (m, 4H), 7.36 (d, J = 8.1 Hz, 1H), 3.74 (s, 2H), 3.14 (s, 2H), 2.56 (s, 3H), 1.81 (s, 6H); 13C NMR (100 MHz, CDCl3) δ 150.8, 146.6, 138.3, 133.9, 130.9, 130.4, 129.8, 128.7, 127.9, 127.4, 126.8, 123.1, 120.7, 49.4, 26.5, 24.3, 22.2; HRMS (ESI) calcd for C21H22N2O: 319.1805 (M + H+), found: 319.1802.
6-Fluoro-3-phenyl-1-(piperidin-1-yl)isoquinoline 2-oxide 3h
1H NMR (400 MHz, CDCl3) δ 8.19 (m, 1H), 7.73 (d, J = 6.6 Hz, 2H), 7.48–7.41 (m, 4H), 7.33–7.29 (m, 2H), 3.72 (s, 2H), 3.13 (s, 2H), 1.71 (s, 6H); 13C NMR (100 MHz, CDCl3) δ 162.1 (d, 1JCF = 249.6 Hz), 151.2, 148.6, 133.5, 131.3, 129.7, 129.1, 128.0, 127.3 (d, 3JCF = 8.9 Hz), 124.4, 120.1 (d, 4JCF = 5.0 Hz), 118.3 (d, 2JCF = 24.9 Hz), 110.9 (d, 2JCF = 21.7 Hz), 49.5, 26.5, 24.3; HRMS (ESI) calcd for C20H19FN2O: 323.1554 (M + H+), found: 323.1557.
3-(4-Chlorophenyl)-1-(piperidin-1-yl)isoquinoline 2-oxide 3i
1H NMR (400 MHz, CDCl3) δ 8.17 (d, J = 8.3 Hz, 1H), 7.72–7.69 (m, 3H), 7.60–7.50 (m, 3H), 7.42 (d, J = 8.5 Hz, 2H), 3.73 (s, 2H), 3.19 (s, 2H), 1.85–1.75 (m, 6H); 13C NMR (100 MHz, CDCl3) δ 151.2, 146.2, 134.9, 132.2, 131.2, 130.2, 128.4, 128.3, 127.3, 126.9, 124.2, 120.7, 49.6, 26.5, 24.3; HRMS (ESI) calcd for C20H19ClN2O: 339.1259 (M + H+), found: 339.1258.
3-(4-Fluorophenyl)-1-(piperidin-1-yl)isoquinoline 2-oxide 3j
1H NMR (400 MHz, CDCl3) δ 8.18 (d, J = 8.1 Hz, 1H), 7.76–7.70 (m, 3H), 7.59–7.50 (m, 3H), 7.13 (t, J = 8.4 Hz, 2H), 3.73 (s, 2H), 3.19 (s, 2H), 1.80 (s, 6H); 13C NMR (100 MHz, CDCl3) δ 163.1 (d, 1JCF = 247.3 Hz), 151.2, 146.4, 131.9 (d, 3JCF = 8.2 Hz), 130.2, 129.8, 128.3 (d, 3JCF = 7.7 Hz), 127.2, 126.9, 124.2, 120.7, 115.0 (d, 2JCF = 21.6 Hz), 49.6, 26.5, 24.3; HRMS (ESI) calcd for C20H19FN2O: 323.1554 (M + H+), found: 323.1543.
3-(4-Methoxyphenyl)-1-(piperidin-1-yl)isoquinoline 2-oxide 3k
1H NMR (400 MHz, CDCl3) δ 8.17 (d, J = 8.1 Hz, 1H), 7.73–7.68 (m, 3H), 7.59–7.48 (m, 3H), 6.98 (d, J = 8.8 Hz, 2H), 3.85 (s, 3H), 3.73 (s, 2H), 3.17 (s, 2H), 1.84–1.75 (m, 6H); 13C NMR (100 MHz, CDCl3) δ 160.1, 151.1, 147.2, 131.3, 130.3, 128.1, 127.9, 127.0, 126.7, 126.1, 124.1, 120.4, 113.4, 55.4, 49.5, 26.6, 24.3; HRMS (ESI) calcd for C21H22N2O2: 335.1754 (M + H+), found: 335.1753.
3-Phenyl-1-(pyrrolidin-1-yl)isoquinoline 2-oxide 3l
1H NMR (400 MHz, CDCl3) δ 8.18 (d, J = 8.2 Hz, 1H), 7.75–7.71 (m, 3H), 7.57–7.52 (m, 3H), 7.50–7.41 (m, 3H), 3.64–3.52 (m, 4H), 2.15–2.05 (m, 4H); 13C NMR (100 MHz, CDCl3) δ 149.7, 147.6, 133.9, 130.9, 129.9, 129.8, 128.9, 128.6, 128.2, 128.1, 126.7, 124.7, 120.9, 48.8, 26.5; HRMS (ESI) calcd for C19H18N2O: 291.1492 (M + H+), found: 291.1492.
1-(Azepan-1-yl)-3-phenylisoquinoline 2-oxide 3m
1H NMR (400 MHz, CDCl3) δ 8.27 (d, J = 8.3 Hz, 1H), 7.76–7.72 (m, 3H), 7.60–7.57 (m, 2H), 7.54–7.50 (m, 1H), 7.48–7.40 (m, 3H), 3.43 (s, 4H), 1.83 (s, 8H); 13C NMR (100 MHz, CDCl3) δ 152.5, 147.6, 133.7, 130.9, 130.1, 129.8, 128.9, 128.4, 128.2, 128.0, 126.8, 124.4, 121.4, 51.1, 30.5, 28.2; HRMS (ESI) calcd for C21H22N2O: 319.1805 (M + H+), found: 319.1803.
1-Morpholino-3-phenylisoquinoline 2-oxide 3n
1H NMR (400 MHz, CDCl3) δ 8.19 (d, J = 8.3 Hz, 1H), 7.75–7.72 (m, 3H), 7.61–7.54 (m, 3H), 7.50–7.43 (m, 3H), 3.96 (s, 8H); 13C NMR (100 MHz, CDCl3) δ 149.5, 147.5, 133.5, 130.9, 130.2, 129.7, 129.0, 128.5, 128.4, 128.1, 127.1, 123.8, 121.3, 67.9, 48.5; HRMS (ESI) calcd for C19H18N2O2: 307.1441 (M + H+), found: 307.1447.
Acknowledgements
This work was supported by the National Natural Science Foundation of China (21172038, 21372046, 21262015), the Science Funds of the Natural Science Foundation of Jiangxi Province (20122BAB203005), and the Project of the Science Funds of Jiangxi Education Office (KJLD13069).
Notes and references
-
(a) D. P. Walsh and Y.-T. Chang, Chem. Rev., 2006, 106, 2476 CrossRef CAS PubMed
;
(b) P. Arya, D. T. H. Chou and M.-G. Baek, Angew. Chem., Int. Ed., 2001, 40, 339 CrossRef CAS
;
(c) S. L. Schreiber, Science, 2000, 287, 1964 CrossRef CAS
;
(d) M. D. Burke and S. L. Schreiber, Angew. Chem., Int. Ed., 2004, 43, 46 CrossRef PubMed
;
(e) S. L. Schreiber, Nature, 2009, 457, 153 CrossRef CAS PubMed
;
(f) D. S. Tan, Nat. Chem. Biol., 2005, 1, 74 CrossRef CAS PubMed
;
(g) C. Cordier, D. Morton, S. Murrison, A. Nelson and C. O'Leary-Steele, Nat. Prod. Rep., 2008, 25, 719 RSC
;
(h) W. R. J. D. Galloway, A. I. Llobet and D. R. Spring, Nat. Commun., 2010, 1, 80 Search PubMed
;
(i) S. Oh and S. B. Park, Chem. Commun., 2011, 47, 12754 RSC
.
- For reviews, see: G. Qiu, Q. Ding and J. Wu, Chem. Soc. Rev., 2013, 42, 5257 RSC
.
- For recent selected examples, see:
(a) J. Sheng, C. Fan, Y. Ding, X. Fan and J. Wu, Chem. Commun., 2014, 50, 4188 RSC
;
(b) G. Liu, H. Liu, S. Pu and J. Wu, RSC Adv., 2013, 3, 10666 RSC
;
(c) Q. Xiao, J. Sheng, Q. Ding and J. Wu, Eur. J. Org. Chem., 2014, 217 CrossRef CAS PubMed
;
(d) Q. Xiao, S. Ye and J. Wu, Org. Lett., 2012, 14, 3430 CrossRef CAS PubMed
.
-
(a) Z. Huo, H. Tomeba and Y. Yamamoto, Tetrahedron Lett., 2008, 49, 5531 CrossRef CAS PubMed
;
(b) H.-S. Yeom, J.-E. Lee and S. Shin, Angew. Chem., Int. Ed., 2008, 47, 7040 CrossRef CAS PubMed
;
(c) H.-S. Yeom, S. Kim and S. Shin, Synlett, 2008, 924 CAS
;
(d) Q. Ding and J. Wu, Adv. Synth. Catal., 2008, 350, 1850 CrossRef CAS PubMed
.
-
(a) G. Qiu, Y. Hu, Q. Ding, Y. Peng and J. Wu, Chem. Commun., 2011, 47, 9708 RSC
;
(b) G. Qiu, Q. Ding, H. Ren, Y. Peng and J. Wu, Org. Lett., 2010, 12, 3975 CrossRef CAS PubMed
.
- For selected examples, see:
(a) C. Zhu, M. Yi, D. Wei, X. Chen, Y. Wu and X. Cui, Org. Lett., 2014, 16, 1840 CrossRef CAS PubMed
;
(b) M. Shang, S.-Z. Sun, H.-X. Dai and J.-Q. Yu, J. Am. Chem. Soc., 2014, 136, 3354 CrossRef CAS PubMed
;
(c) Q. Li, S.-Y. Zhang, G. He, Z. Ai, V. A. Nack and G. Chen, Org. Lett., 2014, 16, 1764 CrossRef CAS PubMed
;
(d) L. D. Trans, J. Roane and O. Daugulis, Angew. Chem., Int. Ed., 2013, 52, 6043 CrossRef PubMed
;
(e) H. Zhao, Y. Shang and W. Su, Org. Lett., 2013, 15, 5106 CrossRef CAS PubMed
;
(f) A. John and K. M. Nicholas, J. Org. Chem., 2011, 76, 4158 CrossRef CAS PubMed
;
(g) H.-Y. Thu, W.-Y. Yu and C.-M. Che, J. Am. Chem. Soc., 2006, 128, 9048 CrossRef CAS PubMed
;
(h) X. Chen, X.-S. Hao, C. E. Goodhue and J.-Q. Yu, J. Am. Chem. Soc., 2006, 128, 6790 CrossRef CAS PubMed
;
(i) D. Monguchi, T. Fujiwara, H. Furukawa and A. Mori, Org. Lett., 2009, 11, 1607 CrossRef CAS PubMed
;
(j) Q. Wang and S. L. Schreiber, Org. Lett., 2009, 11, 5178 CrossRef CAS PubMed
;
(k) J. Y. Kim, S. H. Cho, J. Joseph and S. Chang, Angew. Chem., Int. Ed., 2010, 49, 9899 CrossRef CAS PubMed
;
(l) S. H. Cho, J. Y. Kim, S. Y. Lee and S. Chang, Angew. Chem., Int. Ed., 2009, 48, 9127 CrossRef CAS PubMed
;
(m) Y. Li, Y. Xie, R. Zhang, K. Jin, X. Wang and C. Duan, J. Org. Chem., 2011, 76, 5444 CrossRef CAS PubMed
;
(n) S. Guo, B. Qian, Y. Xie, C. Xia and H. Huang, Org. Lett., 2011, 13, 522 CrossRef CAS PubMed
;
(o) H. Zhao, M. Wang, W. Su and M. Hong, Adv. Synth. Catal., 2010, 352, 1301 CrossRef CAS PubMed
.
Footnotes |
† Electronic supplementary information (ESI) available: Experimental procedure, characterization data, 1H and 13C NMR spectra of compounds 3, the CIF file of compound 3a. CCDC 1016318. For ESI and crystallographic data in CIF or other electronic format see DOI: 10.1039/c4qo00209a |
‡ These authors contributed equally. |
|
This journal is © the Partner Organisations 2014 |