DOI:
10.1039/C4QO00152D
(Research Article)
Org. Chem. Front., 2014,
1, 1077-1082
1,2-Migration in the reactions of ruthenium vinyl carbene with propargyl alcohols†
Received
20th May 2014
, Accepted 25th August 2014
First published on 26th August 2014
Abstract
Reactions of the ruthenium vinyl carbene complex [Ru{CHC(PPh3)CH(2-Py)}Cl2PPh3]BF4 (1) with five propargyl alcohols HC
CC(OH)R1R2, (R1 = R2 = Ph; R1 = Ph, R2 = CH3; R1 = H, R2 = Ph; R1 = H, R2 = CH3; R1 = CH
CH2, R2 = CH3) have been investigated, which led to the formation of several ten-membered η2-olefin coordinated ruthenacycles [Ru{O
CR2CHR1-η2-CH
CHC(PPh3)
CH(2-Py)}Cl2PPh3]BF4 (R1 = R2 = Ph, 2; R1 = Ph, R2 = CH3, 3; R1 = H, R2 = Ph, 4; R1 = H, R2 = CH3, 5; R1 = CH
CH2, R2 = CH3, 6), respectively. In these reactions, insertion of alkynes and intramolecular 1,2-migration of propargyl alcohols were performed in tandem. The results show that the 1,2-migratory preference of the groups is in the order of H > Ph > CH3. Complexes 2, 3, 5, and 6 were characterized by X-ray diffraction analysis and NMR spectra.
Introduction
Ruthenium vinyl carbenes are widely used as carbene initiators in metathesis reactions.1,2 They are also extensively reported as crucial intermediates in enyne metathesis.3 The vinyl substitution has a significant influence on the ruthenium carbene reactivity because the electron-deficient carbene center could be partially stabilized by π–π conjugation.3d Investigation into vinyl carbene reactivity would be helpful in understanding the catalytic enyne metathesis. Nevertheless, in sharp contrast to their extensive use, fundamental reactivity studies on such compounds are less explored.1a,c,4 The first well-defined group 8 vinyl carbene was the neophylidene complex, which was developed by Grubbs’ group in 1992.1a It was stable towards water, alcohol and the diethyl ether solution of HCl.1a However, it could react with strained acyclic olefins and functionalized olefins,4a and catalyze the ROMP of norbornene derivatives.1a,e,f
Recently, we reported a convenient route to prepare a stable ruthenium vinyl carbene 1.5 It was a fused heterocycle different from the reported aromatic metallacycles6 because of the significant alternation of the bond lengths in the ring. The electron in it was quite localized, so that it was more suitable to be viewed as a 1,3-butadiene moiety.5 Its Cγ was easily attacked by nucleophilic reagents to form a series of ruthena-polycyclic complexes [Ru{CHC(PPh3)CHR(2-Py)}Cl(PPh3)2]BF4 [R = OH, OCH3, NHPh].4g It could also react with alkynones and alkynoates in the presence of an acid, leading to the formation of 3-alkenyl-2-phosphonium indolizine salts.5 For our continuous interest in the reactivity of ruthenium vinyl carbene, we have studied the reactions of 1 with five propargyl alcohols bearing different substituents. The results show that the alkyne groups of propargyl alcohols undergo facile insertion into the ruthenium–carbon double bond of 1, yielding a series of ten-membered η2-olefin coordinated ruthenacycles (Chart 1a). The reaction mechanism involves [2 + 2]-cycloaddition and [2 + 2]-cycloreversion reactions, which is related to the typical enyne metathesis steps (Chart 1b).3 It is also similar to our previous reported work of alkynes’ insertion into osmafuran.7 Interestingly, 1,2-migration of the substituents on propargyl alcohols has been observed in the reactions. The results show that the migratory preference of the substituents is in the order of H > Ph > CH3, which is in accordance with the common phenomenon observed in the singlet carbene and metal carbene species.8
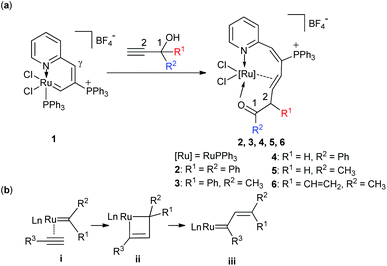 |
| Chart 1 (a) Reactions of 1 with propargyl alcohols; (b) the typical enyne metathesis process. | |
Results and discussion
Reaction of 1 with HC
CC(OH)(Ph)2
A mixture of ruthenium vinyl carbene 1 with HC
CC(OH)(Ph)2 in CHCl3 was stirred at room temperature for 1 h to give a red suspension. The precipitate was collected by filtration in 61% yield and was identified to be a ten-membered η2-olefin coordinated ruthenacycle 2 [Ru{O
CPhCHPh-η2-CH
CHC(PPh3)
CH(2-Py)}Cl2PPh3]BF4 (Scheme 1). Complex 2 had excellent thermal stability. The solid sample of 2 remains nearly unchanged at 120 °C in air for 5 h.
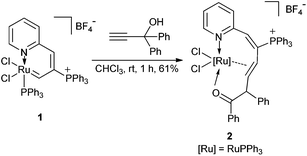 |
| Scheme 1 Reaction of 1 with HC CC(OH)(Ph)2. | |
As shown in Fig. 1, X-ray crystallographic analysis reveals that the atoms O1, C11, C10, C9, C1, C2, C3, C4, N1, together with Ru1, construct the ten-membered distorted metallacycle. The coordination geometry around the Ru atom can be rationalized as a octahedron with the P1 and N1 occupying trans positions (P(1)–Ru(1)–N(1) 174.60(12)°), Cl1 trans to the η2-olefin (C(1)–Ru(1)–Cl(1) 167.20(14)°, C(9)–Ru(1)–Cl(1) 154.70(14)°) and Cl2 trans to O1 (O(1)–Ru(1)–Cl(2) 166.86(10)°). The NMR spectral data are consistent with the X-ray structure. In particular, the 1H NMR spectrum shows the proton signals of η2-olefin at 4.53 and 5.46 ppm, respectively. The H10 signal is observed at 5.77 ppm. The signal at 6.44 ppm is attributed to H3. The 13C NMR spectrum shows the characteristic carbon signals of C(Ph)
O and η2-CH
CH at 218.97, 81.17 and 73.04 ppm, respectively. The signals of the other four carbons C10, C2, C3 and C4 appear at 60.32, 122.14, 147.80 and 151.85 ppm, respectively. The 31P NMR spectrum shows two signals at 23.33 and 37.06 ppm for CPPh3 and RuPPh3, respectively.
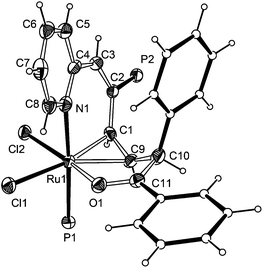 |
| Fig. 1 Molecular structure of complex 2 (ellipsoids at the 50% probability level). The phenyl rings in PPh3 groups and the counter anion are omitted for clarity. Selected bond distances [Å] and angles [°]: Ru(1)–O(1) 2.085(4), O(1)–C(11) 1.246(6), C(10)–C(11) 1.510(7), C(9)–C(10) 1.526(8), C(1)–C(9) 1.412(7), C(1)–C(2) 1.481(7), C(2)–C(3) 1.348(7), C(3)–C(4) 1.452(7), N(1)–C(4) 1.359(7), Ru(1)–C(1) 2.176(5), Ru(1)–C(9) 2.164(5), Ru(1)–N(1) 2.192(4), O(1)–Ru(1)–C(9) 74.64(17), O(1)–Ru(1)–C(1) 109.99(17), O(1)–C(11)–C(10) 117.7(5), C(9)–C(10)–C(11) 105.2(4), C(10)–C(9)–Ru(1) 110.2(3), C(9)–C(1)–C(2) 130.2(4), C(1)–C(2)–C(3) 129.0(5), C(2)–C(3)–C(4) 129.2(5), N(1)–C(4)–C(3) 122.3(4), O(1)–Ru(1)–N(1) 85.59(15), C(4)–N(1)–Ru(1) 126.6(3), C(9)–Ru(1)–C(1) 37.98(19), N(1)–Ru(1)–P(1) 174.60(12), C(1)–Ru(1)–Cl(1) 167.20(14), O(1)–Ru(1)–Cl(1) 82.35(11), C(9)–Ru(1)–Cl(1) 154.70(14), O(1)–Ru(1)–Cl(2) 166.86(10). | |
A proposed mechanism for the formation of 2 is shown in Scheme 2. Firstly, the alkyne coordinates to the unsaturated Ru center, leading to a π-alkyne intermediate A. The addition of HC
CC(OH)(Ph)2 into 1via a [2 + 2] cycloaddition process gives metallacyclobutene intermediate B, which is a highly regioselective process probably due to the steric hindrance of the nearby bulky phosphonium substituent. The following cycloreversion generates the carbene intermediate C. Then the hydroxyl is activated by the ruthenium center to form the hydride-ruthenacycle D, which is followed by 1,2-phenyl migration to generate intermediate E. The subsequent reductive elimination, coordination of the carbonyl group and the internal alkene gave the 18-e complex 2. The deuterium-labeling experiment showed that 77% of hydrogen on C10 was deuterated when HC
CC(OD)(Ph)2 was used, which suggests that the hydrogen on C10 comes from an active hydrogen.
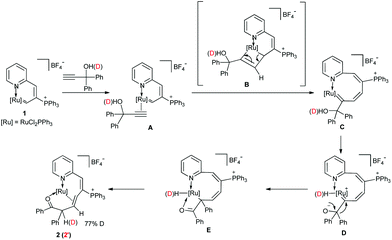 |
| Scheme 2 Proposed mechanisms for the formation of 2 (2′). | |
Investigation of 1,2-migratory preferences
It is worth noting that an intramolecular 1,2-phenyl migration is observed in the formation of 2 (from D to E). The migration is induced by the electrophilic carbon of the ruthenium carbene intermediate D. 1,2-Migration of free carbene or metal carbene has been widely utilized in organic synthesis, especially for catalytic reactions that incorporate rhodium,8c–h,9 gold,8i,10 and platinum carbenes.8i,10d–e,11 The inherent migratory preferences are in the order of H > Ar > alkyl, though the migratory preferences are also affected by nonmigrating factors.8a,9 However, the 1,2-migratory preferences of Ru carbene are rarely reported,12 thus propargyl alcohols with different substituents were subjected to the reaction of ruthenium vinyl carbene 1.
Reactions of 1 with propargyl alcohols HC
CC(OH)CH3(Ph) at room temperature readily afforded complex 3 in good isolated yields. Similarly, treatment of 1 with HC
CCH(OH)Ph and HC
CCH(OH)CH3 at room temperature could also generate complexes 4 and 5, and reacting them at 60 °C could slightly increase the yields (Scheme 3). Complexes 3, 4, and 5 were also determined as ten-membered η2-olefin coordinated ruthenacycles which were similar to complex 2. The structures of 3 and 5 were also confirmed by X-ray single-crystal structural analysis, in which the Ru atoms are surrounded by a tridentate ligand (N, η2-CH
CH, O sites) (ESI, Fig. S1-1 and S1-2†).
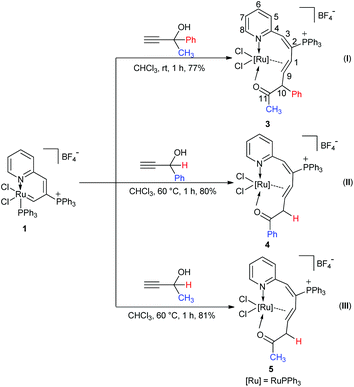 |
| Scheme 3 Reactions of 1 with propargyl alcohols bearing different migratory groups. | |
The solution NMR spectra of the three isolated ten-membered η2-olefin coordinated ruthenacycles show the characteristic 1H NMR signals of the η2-olefin at around 4.3–5.5 ppm, which is within the typical proton chemical shift range of η2-olefin.13 In complex 3, the 1H NMR spectrum shows the proton signal on C10 at 5.30 ppm, which is significantly lower compared with those of 4 (3.70, 2.92 ppm) and 5 (3.20, 2.71 ppm) respectively, probably due to the deshielding effect by the adjacent Ph group. In the 13C NMR spectra, the carbon signals of the η2-olefin for these three complexes are in the range of 75.24 to 81.06 ppm. The 31P NMR spectra show similar signals for the three complexes (see the Experimental section), which confirms they are analogous structures as well.
Both the solid and solution states analyses confirmed the well-defined structures of 3, 4, and 5. From the product structures in Scheme 3, migratory aptitudes for H, Ph and CH3 are evident. As observed in reaction (I), the phenyl group was in preference to migrate compared with the CH3 group. The hydrogen was more likely to migrate than the phenyl group (reaction (II)). This is consistent with the fact that 1,2-H migration is a highly favorable process. Accordingly, the migration of H was also easier than CH3 (reaction (III)). Thus, 1,2-migratory preference for the groups is in the order of H > Ph > CH3, which is consistent with the inherent migratory aptitude of singlet free carbenes and metal carbenes.8
In addition, complex 6 was prepared when HC
CC(OH)CH3 (CH
CH2) was subjected to this reaction (Scheme 4). The structure of 6 was also confirmed by X-ray diffraction, and its structure is depicted in Fig. 2. The X-ray diffraction study and solution NMR spectrum confirmed that complex 6 was similar to complexes 2, 3, 4, and 5 (see the Experimental section). It is noted that the vinyl group migrates preferentially as compared to the methyl group and the π-bond isomerization occurs during the migration process.
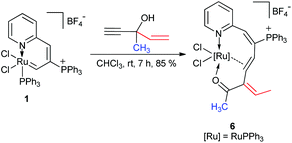 |
| Scheme 4 Reaction of 1 with HC CC(OH)CH3(CH CH2). | |
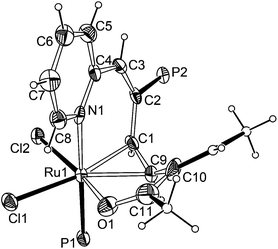 |
| Fig. 2 Molecular structure of complex 6 (ellipsoids at the 50% probability level). The phenyl rings in PPh3 groups and the counter anion are omitted for clarity. Selected bond distances [Å] and angles [°] of complex 6: Ru(1)–O(1) 2.092(4), O(1)–C(11) 1.223(8), C(10)–C(11) 1.446(11), C(9)–C(10) 1.506(10), C(1)–C(9) 1.391(9), C(1)–C(2) 1.479(8), C(2)–C(3) 1.337(8), C(3)–C(4) 1.457(8), N(1)–C(4) 1.361(7), Ru(1)–C(1) 2.164(6), Ru(1)–C(9) 2.135(6), Ru(1)–N(1) 2.135(6), O(1)–Ru(1)–C(9) 75.2(2), O(1)–Ru(1)–C(1) 110.9(2), O(1)–C(11)–C(10) 117.4(7), C(9)–C(10)–C(11) 110.8(6), C(10)–C(9)–Ru(1) 105.4(4), C(9)–C(1)–C(2) 128.2(6), C(1)–C(2)–C(3) 129.1(6), C(2)–C(3)–C(4) 129.2(6), N(1)–C(4)–C(3) 121.9(5), O(1)–Ru(1)–N(1) 81.15(17), C(4)–N(1)–Ru(1) 127.1(4), C(9)–Ru(1)–C(1) 37.8(2). | |
Conclusions
In conclusion, we have investigated the reactions of ruthenium vinyl carbene 1 with five propargyl alcohols, which led to the formation of a series of ten-membered η2-olefin coordinated ruthenacycles. The proposed mechanisms for the formation of these complexes involve a regioselective [2 + 2] cycloaddition and 1,2-migration process. By introduction of different substituents on propargyl alcohols, we conclude that the intramolecular 1,2-migratory preference of the groups is in the order of H > Ph > CH3, which is consistent with the inherent migratory aptitude of singlet free carbenes and metal carbenes. Further studies on the reactivities of ruthenium vinyl carbenes are in progress in our laboratory.
Experimental
All syntheses were carried out under an inert atmosphere (N2) by means of standard Schlenk techniques, unless otherwise stated. Solvents were distilled from sodium/benzophenone (hexane and diethyl ether) or calcium hydride (dichloromethane, chloroform) under N2 prior to use. Reagents were used as received from commercial sources without further purification. NMR spectroscopic experiments were performed on an AVIII-400 (1H, 400.13 MHz; 13C, 100.63 MHz; 31P, 161.96 MHz) spectrometer at room temperature. 1H and 13C NMR chemical shifts (δ) are relative to tetramethylsilane, and 31P NMR chemical shifts are relative to 85% H3PO4. The absolute values of the coupling constants are given in hertz (Hz). Multiplicities are abbreviated as singlet (s), doublet (d), triplet (t), multiplet (m) and broad (br). Elemental analyses were performed on a Vario EL III elemental analyzer.
Compound 2
A mixture of 1,1-diphenyl-2-propyn-1-ol (624 mg, 3.00 mmol) and compound 1 (899 mg, 1.00 mmol) in CHCl3 (30 mL) was stirred at room temperature for 1 h to give a red suspension, which was collected by filtration, washed with Et2O (3 × 10 mL) and dried under vacuum. Yield: 675 mg, 61%.
1H NMR (400.13 MHz, CD
2Cl
2):
δ = 9.73 (d,
J(HH) = 5.3 Hz, 1 H, H8), 6.44 (d,
J(PH) = 23.9 Hz, 1 H, H3), 6.22 (t,
J(HH) = 7.09 Hz, 1 H, an aromatic proton, confirmed by
1H–
13C HSQC), 5.77 (d,
J(HH) = 7.8 Hz, 1 H, H10), 5.52 (d,
J(HH) = 7.11 Hz, 1 H, an aromatic proton, confirmed by
1H–
13C HSQC), 5.46 (m, 1 H, H9), 4.64 (d,
J(HH) = 7.28 Hz, 1 H, an aromatic proton, confirmed by
1H–
13C HSQC), 4.53 (dd, apparent t,
J(PH) = 9.2 Hz,
J(HH) = 9.2 Hz, 1 H, H1), 7.90–6.73 ppm (40 H, other aromatic carbon atoms);
31P NMR (161.96 MHz, CD
2Cl
2): 23.33 (s, C
PPh
3), 37.06 ppm (s, Ru
PPh
3).
13C NMR (100.63 MHz, CD
2Cl
2):
δ = 218.97 (s, C11), 157.02 (s, C8), 151.85 (d,
J(PC) = 19.3 Hz, C4), 147.80 (d,
J(PC) = 19.8 Hz, C3), 122.14 (d,
J(PC) = 72.4 Hz, C2), 116.09 (d,
J(PC) = 87.23 Hz, carbons on phenyls of PPh
3), 81.11 (d,
J(PC) = 4.1 Hz, C9), 73.04 (d,
J(PC) = 12.4 Hz, C1), 60.32 (s, C10), 139.00–115.65 ppm (other aromatic carbon atoms); Anal. Calcd for C
59H
48Cl
2P
2BF
4NORu: C, 63.97; H, 4.37; N, 1.26. Found: C, 63.84; H, 4.28; N, 1.14.
Compound 3
2-Phenyl-3-butyn-2-ol (439 mg, 3.00 mmol) was added to a solution of compound 1 (899 mg, 1.00 mmol) in CHCl3 (30 mL) at room temperature. The mixture was stirred for 1 h to give a red solution, and then the same volume of Et2O was added to obtain a precipitate which was collected by filtration. The precipitate was washed with Et2O (3 × 10 mL) and dried under vacuum. Yield: 806 mg, 77%.
1H NMR (400.13 MHz, CD
2Cl
2):
δ = 9.64 (d,
J(HH) = 5.3 Hz, 1 H, H8), 6.42 (d,
J(PH) = 23.5 Hz, 1 H, H3), 6.42 (1 H, an aromatic proton overlapped with H3, confirmed by
1H–
13C HSQC), 5.46 (m, 1 H, H9), 5.46 (1 H, 1 H, an aromatic proton overlapped with H9, confirmed by
1H–
13C HSQC), 5.30 (d,
J(HH) = 7.8 Hz, 1 H, H10), 4.61 (d, 1 H,
J(HH) = 6.37 Hz, an aromatic proton, confirmed by
1H–
13C HSQC), 4.47 (dd, apparent t,
J(PH) = 9.8 Hz,
J(HH) = 9.8 Hz, 1 H, H1), 1.70 (s, 3 H, H12), 7.87–6.77 ppm (36 H, other aromatic carbon atoms).
31P NMR (161.96 MHz, CD
2Cl
2): 23.26 (s, C
PPh
3), 37.82 ppm (s, Ru
PPh
3).
13C NMR (100.63 MHz, CD
2Cl
2):
δ = 232.93 (s, C11), 157.14 (s, C8), 151.88 (d,
J(PC) = 19.7 Hz, C4), 147.78 (d,
J(PC) = 15.1 Hz, C3), 122.21 (d,
J(PC) = 72.1 Hz, C2), 116.00 (d,
J(PC) = 87.9 Hz, carbons on phenyls of PPh
3), 81.06 (d,
J(PC) = 4.0 Hz, C9), 73.04 (d,
J(PC) = 12.4 Hz, C1), 65.34 (s, C10), 27.7 (s, C12), 139.14–126.00 ppm (other aromatic carbon atoms). Anal. Calcd for C
54H
46Cl
2P
2BF
4NORu: C, 62.02; H, 4.43; N, 1.34. Found: C, 61.90; H, 4.33; N, 1.32.
Compound 4
1-Phenyl-2-propyn-1-ol (365 μL, 3.00 mmol) was added to a suspension of complex 1 (899 mg, 1.00 mmol) in CHCl3 (30 mL). The mixture was stirred at 60 °C for 1 h to give a red precipitate. The precipitate was collected by filtration, washed with Et2O (2 × 5 mL) and dried under vacuum to give 4 (824 mg, 80%) as a red solid.
Complex
4 was separated from the reaction mixture as a precipitate, and the solubility of pure complex
4 was so poor that it prevented NMR characterization. We just characterized complex
4 by
in situ NMR.
In situ1H NMR (400.13 MHz, CD
2Cl
2):
δ = 9.41 (d,
J(HH) = 3.1 Hz, 1 H, H8), 4.84 (br, 1 H, H9), 4.34 (dd, apparent t,
J(PH) = 9.1 Hz,
J(HH) = 9.1 Hz, 1 H, H1), 3.70 (br, 1 H, H10), 2.92 (br, 1 H, H10), 7.77–6.98 ppm (39 H, other aromatic carbon atoms and H3);
In situ31P NMR (161.96 MHz, CD
2Cl
2): 23.40 (s, C
PPh
3), 38.12 ppm (s, Ru
PPh
3).
In situ13C NMR (100.63 MHz, CD
2Cl
2):
δ = 218.79 (s, C11), 156.29 (s, C8), 150.6 (d,
J(PC) = 19.7 Hz, C4), 147.80 (d,
J(PC) = 15.5 Hz, C3), 122.88 (d,
J(PC) = 72.7 Hz, C2), 116.70 (d,
J(PC) = 86.7 Hz, carbons on phenyls of PPh
3), 80.80 (d,
J(PC) = 3.5 Hz, C9), 77.00 (d,
J(PC) = 12.3 Hz, C1), 45.34 (s, C10), 138.78–126.50 ppm (other aromatic carbon atoms); Anal. Calcd for C
53H
44BCl
2F
4NOP
2Ru: C, 61.70; H, 4.30; N, 1.36. Found: C, 61.79; H, 4.66; N, 1.64.
Compound 5
3-Butyn-2-ol (221 μL, 3.00 mmol) was added to a suspension of compound 1 (899 mg, 1.00 mmol) in CHCl3 (30 mL). The mixture was stirred at 60 °C for 1 h to give a brown solution. The solution was concentrated to ca. 2 mL, and then Et2O (20 mL) was added to the solution. The precipitate was collected by filtration, washed with Et2O (2 × 5 mL) and dried under vacuum to give 5 (780 mg, 81%) as a nacarat solid.
1H NMR (400.13 MHz, CD
2Cl
2–CDCl
3 = 3
![[thin space (1/6-em)]](https://www.rsc.org/images/entities/char_2009.gif)
:
![[thin space (1/6-em)]](https://www.rsc.org/images/entities/char_2009.gif)
5):
δ = 9.54 (d,
J(HH) = 4.3 Hz, 1 H, H8), 4.70 (br, 1 H, H9), 4.36 (dd, apparent t,
J(PH) = 9.1 Hz,
J(HH) = 9.1 Hz, 1 H, H1), 3.20 (br, 1 H, H10), 2.71 (br, 1 H, H10), 1.80 (s, 3 H, H12), 7.78–7.04 ppm (34 H, other aromatic carbon atoms and H3);
31P NMR (161.96 MHz, CD
2Cl
2–
![[thin space (1/6-em)]](https://www.rsc.org/images/entities/char_2009.gif)
CDCl
3 = 3
![[thin space (1/6-em)]](https://www.rsc.org/images/entities/char_2009.gif)
:
![[thin space (1/6-em)]](https://www.rsc.org/images/entities/char_2009.gif)
5): 23.45 (s, C
PPh
3), 38.75 ppm (s, Ru
PPh
3).
13C NMR (100.63 MHz, CD
2Cl
2–CDCl
3 = 3
![[thin space (1/6-em)]](https://www.rsc.org/images/entities/char_2009.gif)
:
![[thin space (1/6-em)]](https://www.rsc.org/images/entities/char_2009.gif)
5):
δ = 232.03 (s, C11), 155.29 (s, C8), 149.48 (d,
J(PC) = 19.6 Hz, C4), 147.21 (d,
J(PC) = 15.6 Hz, C3), 121.16 (d, J(PC) = 71.36 Hz, C2), 115.84 (d,
J(PC) = 87.2 Hz, carbons on phenyls of PPh
3), 79.45 (d,
J(PC) = 2.7 Hz, C9), 75.24 (d,
J(PC) = 12.4 Hz, C1), 48.99 (s, C10), 27.69 (s, C12), 137.83–124.93 ppm (other aromatic carbon atoms). Anal. Calcd for C
48H
42BCl
2F
4NOP
2Ru: C, 59.46; H, 4.37; N, 1.44. Found: C, 59.37; H, 4.18; N, 1.47.
Compound 6
3-Methyl-1-penten-4-yn-3-ol (162 μL, 1.50 mmol) was added to a suspension of complex 1 (449 mg, 0.50 mmol) in CHCl3 (30 mL). The mixture was stirred at room temperature for 8 h to give a red solution. The solution was concentrated to ca. 2 mL, and then Et2O (20 mL) was added to the solution. The precipitate was collected by filtration, washed with Et2O (2 × 5 mL) and dried under vacuum to give 6 (422 mg, 85%) as a nacarat solid.
1H NMR (400.13 MHz, CD
2Cl
2):
δ = 9.63 (d,
J(HH) = 5.7 Hz, 1 H, H8), 7.02 (d,
J(PH) = 23.1 Hz, 1 H, H3), 6.40 (q,
J(HH) = 7.1 Hz, 1 H, H13), 5.59 (dd, apparent t,
J(HH) = 8.9 Hz,
J(PH) = 8.9 Hz, 1 H, H9), 4.69 (dd, apparent t,
J(PH) = 8.9 Hz,
J(HH) = 8.9 Hz, 1 H, H1), 1.67 (s, 3 H, H12), 0.86 (d,
J(HH) = 7.1 Hz, 3 H, H14), 7.84–7.06 ppm (33 H, other aromatic carbon atoms);
31P NMR (161.96 MHz, CD
2Cl
2): 24.80 (s, C
PPh
3), 39.25 ppm (s, Ru
PPh
3).
13C NMR (100.63 MHz, CD
2Cl
2):
δ = 217.13 (s, C11), 157.40 (s, C8), 150.92 (d,
J(PC) = 18.9 Hz, C4), 149.52 (s, C13), 146.79 (d,
J(PC) = 15.1 Hz, C3), 142.73 (s, C10), 124.37 (d, J(PC) = 70.22 Hz, C2), 116.45 (d,
J(PC) = 87.2 Hz, carbons on phenyls of PPh
3), 73.90 (t,
J(PC) = 4.0 Hz, C9), 70.66 (d,
J(PC) = 11.1 Hz, C1), 24.15 (s, C12), 15.37 (s, C14), 138.78–125.68 (other aromatic carbon atoms). Anal. Calcd for C
50H
44BCl
2F
4NOP
2Ru: C, 60.32; H, 4.45; N, 1.41. Found: C, 60.19; H, 4.33; N, 1.68.
Acknowledgements
We thank the 973 Program (2012CB821600), the National Natural Science Foundation of China (21332002).
Notes and references
- For ruthenium vinyl carbenes as carbene initiators in olefin metathesis reactions, see:
(a) S. T. Nguyen, L. K. Johnson, R. H. Grubbs and J. W. Ziller, J. Am. Chem. Soc., 1992, 114, 3974–3975 CrossRef CAS;
(b) G. C. Fu, S. T. Nguyen and R. H. Grubbs, J. Am. Chem. Soc., 1993, 115, 9856–9857 CrossRef CAS;
(c) S. T. Nguyen, R. H. Grubbs and J. W. Ziller, J. Am. Chem. Soc., 1993, 115, 9858–9859 CrossRef CAS;
(d) Z. Wu, A. D. Benedicto and R. H. Grubbs, Macromolecules, 1993, 26, 4975–4977 CrossRef CAS;
(e) C. Fraser and R. H. Grubbs, Macromolecules, 1995, 28, 7248–7255 CrossRef CAS;
(f) S. Kanaoka and R. H. Grubbs, Macromolecules, 1995, 28, 4707–4713 CrossRef CAS;
(g) B. R. Maughon and R. H. Grubbs, Macromolecules, 1997, 30, 3459–3469 CrossRef CAS;
(h) C. W. Bielawski and R. H. Grubbs, Prog. Polym. Sci., 2007, 32, 1–29 CrossRef CAS PubMed.
- For ruthenium vinyl carbenes as carbene initiators in enyne metathesis reactions, see:
(a) S.-H. Kim, N. Bowden and R. H. Grubbs, J. Am. Chem. Soc., 1994, 116, 10801–10802 CrossRef CAS;
(b) A. Kinoshita and M. Mori, Synlett, 1994, 1020–1022 CrossRef CAS.
- Reviews for enyne metathesis, see:
(a) C. S. Poulsen and R. Madsen, Synthesis, 2003, 1–18 CAS;
(b) S. T. Diver and A. J. Giessert, Chem. Rev., 2004, 104, 1317–1382 CrossRef CAS PubMed;
(c) E. C. Hansen and D. Lee, Acc. Chem. Res., 2006, 39, 509–519 CrossRef CAS PubMed;
(d) S. T. Diver, Coord. Chem. Rev., 2007, 251, 671–701 CrossRef CAS PubMed;
(e) M. Mori, Adv. Synth. Catal., 2007, 349, 121–135 CrossRef CAS PubMed;
(f) H. Villar, M. Frings and C. Bolm, Chem. Soc. Rev., 2007, 36, 55–66 RSC;
(g) S. Kotha, M. Meshram and A. Tiwari, Chem. Soc. Rev., 2009, 38, 2065–2092 RSC;
(h) M. Mori, Materials, 2010, 3, 2087–2140 CrossRef CAS PubMed;
(i) J. Li and D. Lee, Eur. J. Org. Chem., 2011, 4269–4287 CrossRef CAS PubMed.
- For reactions with olefins, see:
(a) Z. Wu, S. T. Nguyen, R. H. Grubbs and J. W. Ziller, J. Am. Chem. Soc., 1995, 117, 5503–5511 CrossRef CAS;
(b) S. M. Hansen, F. Rominger, M. Metz and P. Hofmann, Chem. – Eur. J., 1999, 5, 557–566 CrossRef CAS. For ligand substitution reactions, see:
(c) M. A. Esteruelas, F. J. Lahoz, E. Oñate, L. A. Oro and B. Zeier, Organometallics, 1994, 13, 4258–4265 CrossRef CAS;
(d) D. Amoroso, G. P. A. Yap and D. E. Fogg, Organometallics, 2002, 21, 3335–3343 CrossRef CAS;
(e) M. A. O. Volland, S. M. Hansen, F. Rominger and P. Hofmann, Organometallics, 2004, 23, 800–816 CrossRef CAS. For reactions with nucleophilic reagents, see:
(f) K. J. Harlow, A. F. Hill, T. Welton, A. J. P. White and D. J. Williams, Organometallics, 1998, 17, 1916–1918 CrossRef CAS;
(g) C. Zhang, H. Zhang, A. Wei, X. He and H. Xia, Acta Chim. Sin., 2013, 71, 1373–1378 CrossRef CAS.
- C. Zhang, H. Zhang, L. Zhang, T. B. Wen, X. He and H. Xia, Organometallics, 2013, 32, 3738–3743 CrossRef CAS.
- Reviews for aromatic metallacycles, see:
(a) C. W. Landorf and M. M. Haley, Angew. Chem., Int. Ed., 2006, 45, 3914–3936 CrossRef CAS PubMed;
(b) L. J. Wright, Dalton Trans., 2006, 1821–1827 RSC;
(c) J. R. Bleeke, Acc. Chem. Res., 2007, 40, 1035–1047 CrossRef CAS PubMed;
(d) I. Fernández and G. Frenking, Chem. – Eur. J., 2007, 13, 5873–5884 CrossRef PubMed;
(e) J. Chen and G. Jia, Coord. Chem. Rev., 2013, 257, 2491–2521 CrossRef CAS PubMed;
(f) X.-Y. Cao, Q. Zhao, Z. Lin and H. Xia, Acc. Chem. Res., 2014, 47, 341–354 CrossRef CAS PubMed.
- Y. Lin, L. Gong, H. Xu, X. He, T. B. Wen and H. Xia, Organometallics, 2009, 28, 1524–1533 CrossRef CAS.
- For 1,2-migratory preference of free carbene, see:
(a) A. Nickon, Acc. Chem. Res., 1993, 26, 84–89 CrossRef CAS;
(b)
Carbene Chemistry, ed. G. Bertrand and S. A. FontisMedia, Marcel Dekker, Inc., Lausanne, Switzerland, 2005 Search PubMed. For 1,2-migratory preference of Rh carbene, see:
(c) R. Pellicciari, R. Fringuelli, P. Ceccherelli and E. Sisani, J. Chem. Soc., Chem. Commun., 1979, 959–960 RSC;
(d) K. Nagao, M. Chiba and S.-W. Kim, Synthesis, 1983, 197–199 CrossRef CAS;
(e) F. Xiao and J. Wang, J. Org. Chem., 2006, 71, 5789–5791 CrossRef CAS PubMed;
(f) T. Miura, Y. Funakoshi, M. Morimoto, T. Biyajima and M. Murakami, J. Am. Chem. Soc., 2012, 134, 17440–17443 CrossRef CAS PubMed;
(g) N. Selander, B. T. Worrell and V. V. Fokin, Angew. Chem., Int. Ed., 2012, 51, 13054–13057 CrossRef CAS PubMed;
(h) A. V. Gulevich and V. Gevorgyan, Angew. Chem., Int. Ed., 2013, 52, 1371–1373 CrossRef CAS PubMed. For 1,2-migratory preference of some other metal carbene, see a review:
(i) B. Crone and S. F. Kirsch, Chem. – Eur. J., 2008, 14, 3514–3522 CrossRef CAS PubMed.
-
(a) M. Vitale, T. Lecourt, C. G. Sheldon and V. K. Aggarwal, J. Am. Chem. Soc., 2006, 128, 2524–2525 CrossRef CAS PubMed;
(b) Z. Zhang and J. Wang, Tetrahedron, 2008, 64, 6577–6605 CrossRef CAS PubMed, and references therein;
(c) Z. Zhang, W. Shi, J. Zhang, B. Zhang, B. Liu, Y. Liu, B. Fan, F. Xiao, F. Xu and J. Wang, Chem. – Asian J., 2010, 5, 1112–1119 CrossRef CAS PubMed.
-
(a) S. Ghorpade, M. D. Su and R. S. Liu, Angew. Chem., Int. Ed., 2013, 52, 4229–4234 CrossRef CAS PubMed;
(b) Z. Zhang, X. Tang, Q. Xu and M. Shi, Chem. – Eur. J., 2013, 19, 10625–10631 CrossRef CAS PubMed;
(c) Y. Chen, L. Wang, N. Sun, X. Xie, X. Zhou, H. Chen, Y. Li and Y. Liu, Chem. – Eur. J. DOI:10.1002/chem.201403113;
(d) Y. Qiu, C. Fu, X. Zhang and S. Ma, Chem. – Eur. J., 2014, 20, 10314–10322 CrossRef CAS PubMed;
(e) Y. Qiu, D. Ma, W. Kong, C. Fu and S. Ma, Org. Chem. Front., 2014, 1, 62–67 RSC.
-
(a) H. Kusama, H. Sogo, K. Saito, T. Suga and N. Iwasawa, Synlett, 2013, 1364–1370 CAS;
(b) J. Li, C. Sun, S. Demerzhan and D. Lee, J. Am. Chem. Soc., 2011, 133, 12964–12967 CrossRef CAS PubMed;
(c) H. Kusama, E. Watanabe, K. Ishida and N. Iwasawa, Chem. – Asian J., 2011, 6, 2273–2277 CrossRef CAS PubMed;
(d) X.-Z. Shu, S.-C. Zhao, K.-G. Ji, Z.-J. Zheng, X.-Y. Liu and Y.-M. Liang, Eur. J. Org. Chem., 2009, 117–122 CAS;
(e) K.-G. Ji, X.-Z. Shu, J. Chen, S.-C. Zhao, Z.-J. Zheng, L. Lu, X.-Y. Liu and Y.-M. Liang, Org. Lett., 2008, 10, 3919–3922 CrossRef CAS PubMed.
- L. Peng, X. Zhang, J. Ma and J. Wang, Org. Chem. Front., 2014, 1, 235–239 RSC.
-
(a) P. Álvarez, E. Lastra, J. Gimeno, P. Braña, J. A. Sordo, J. Gomez, L. R. Falvello and M. Bassetti, Organometallics, 2004, 23, 2956–2966 CrossRef;
(b) E. Mothes, S. Sentets, M. A. Luquin, R. Mathieu, N. Lugan and G. Lavigne, Organometallics, 2008, 27, 1193–1206 CrossRef CAS;
(c) M. Hirano, Y. Arai, Y. Hamamura, N. Komine and S. Komiya, Organometallics, 2012, 31, 4006–4019 CrossRef CAS.
Footnote |
† Electronic supplementary information (ESI) available: Materials including copies of 1H and 13C NMR spectra of all new products and crystallographic data for 2, 3, 5, 6. CCDC 993228, 993229, 993231, 993230. For ESI and crystallographic data in CIF or other electronic format see DOI: 10.1039/c4qo00152d |
|
This journal is © the Partner Organisations 2014 |
Click here to see how this site uses Cookies. View our privacy policy here.