DOI:
10.1039/C4QO00126E
(Research Article)
Org. Chem. Front., 2014,
1, 742-747
Highly stereoselective construction of adjacent tetrasubstituted carbon stereogenic centres via an organocatalytic Mukaiyama-aldol reaction of monofluorinated silyl enol ethers to isatins†
Received
28th April 2014
, Accepted 29th May 2014
First published on 30th May 2014
Abstract
We report the first organocatalytic activation of monofluorinated silyl enol ethers 1, by nucleophilic tertiary amine catalysis, to develop asymmetric reactions for the construction of fully substituted chiral carbons featuring a C–F bond. Accordingly, a highly stereoselective Mukaiyama-aldol reaction of isatins to furnish hydroxyoxindoles bearing two adjacent tetrasubstituted carbon stereocenters is developed.
Introduction
Tetrasubstituted stereogenic carbon centres featuring a fluorine atom occur in a number of natural products, drugs and bioactive compounds.1 For example, the first isolated naturally occurring organofluorine compound, nucleocidin, contains a fluorinated O-containing fully substituted carbon, which is a broad antibacterial and trypanocidal agent produced by Streptomyces calvus.1a The fluticasone propionate is a popularly used drug to treat asthma and eosinophilic esophagitis.1b BMS-204352,1c developed by Bristol-Myers Squibb, is a promising agent for the treatment of stroke (Fig. 1). Obviously, facile access to these compounds and their analogues is important for the corresponding activity–structure relationship study. On the other hand, tetrasubstituted stereogenic carbon centres widely exist in bioactive compounds,2 and their analogues featuring a fluorinated tetrasubstituted carbon are interesting for medicinal research, as selective introduction of a fluorine into chiral centres has proven to be a fruitful strategy to modulate the chemical & physical properties, metabolic stability and biological activities of chiral compounds.3 Therefore, efficient methods allowing the catalytic asymmetric creation of tetrasubstituted carbon stereogenic centres bearing a C–F bond are highly desirable.4
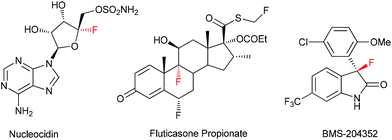 |
| Fig. 1 Representative bioactive compounds. | |
So far, both electrophilic fluorination5 and nucleophilic fluorination6 have been utilized for the catalytic enantioselective construction of fluorinated chiral centres. However, protocols allowing the efficient synthesis of fluorinated tetrasubstituted carbon stereogenic centres were still limited.7 Noticeably, the asymmetric nucleophilic fluorination is much less explored in terms of reaction types.6 While several organofluoro reagents had been developed and tried in asymmetric reactions,6 only prochiral α-fluoro ketones8 or 1,3-dicarbonyl compounds,9 fluorinated silyl enol ethers,10 1-fluoro(phenylsulfonyl)methane derivatives11 and 3-fluorooxindole12 were capable of syntheses of fluorinated fully substituted chiral carbons. In addition, their potential has not been fully explored. For example, monofluorinated silyl enol ethers 1 represent a type of versatile synthon for the synthesis of tertiary α-fluorinated ketones that had attracted much attention,13 but its application in asymmetric catalysis was rare. So far, only one example has been reported: in 2007, Paquin and co-workers achieved highly enantioselective syntheses of α-allyl α-fluoroketones from 1via a Pd-catalyzed asymmetric allylic substitution reaction (eqn (1)).10
| 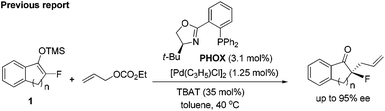 | (1) |
| 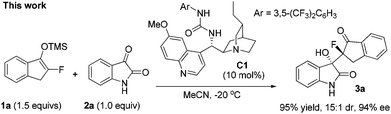 | (2) |
We have been engaged in catalytic asymmetric syntheses of privileged scaffolds featuring tetrasubstituted carbon stereogenic centres, such as 3,3-disubstituted oxindoles14 and fluorinated compounds.15 Considering the great potential of silyl enol ethers 1 in the synthesis of diverse fluorinated tetrasubstituted carbons, we tried to identify an organocatalytic method to activate it to develop asymmetric reactions, as organocatalysis alleviates the contamination of products by heavy metals, very attractive for medicinal research. Here, we wish to report that tertiary amines could efficiently activate prochiral monofluorinated enol ethers 1, contributing to a highly stereoselective Mukaiyama-aldol reaction of isatins to efficiently furnish two adjacent tetrasubstituted carbon stereocenters (eqn (2)).
Results and discussion
Previously, we discovered that tertiary amines could effectively activate difluoroenoxysilane 4, which contributed to a highly enantioselective Mukaiyama-aldol reaction using isatins15b or β,γ-unsaturated α-ketoesters15c to prepare difluoromethylated chiral tertiary alcohols. In the following studies, dramatic fluorine effects were observed in the bifunctional urea C1 catalyzed Mukaiyama-aldol reaction of N-methylisatin: while the reaction of difluoroenoxysilane 4 efficiently afforded the desired product 5 in 89% yield and 94% ee, the non-fluorinated silyl enol ether 6 proved to be much less reactive under the same conditions, giving the product 7 in only 10% yield with 27% ee. This observation was counterintuitive, as the electron-withdrawing effect of the fluorine atom should decrease the nucleophilicity of difluoroenoxysilane 4, as compared with the non-fluorinated one. Although why difluoro substitution enhanced the reactivity of silyl enol ether 4 was not clear till now, it was of interest to us whether monofluorinated silyl enol ethers 1 could be effectively harnessed by nucleophilic tertiary amine catalysis16 to develop catalytic asymmetric reactions, allowing efficient construction of tetrasubstituted stereogenic carbon centers featuring a C–F bond.
Therefore, we tried the reaction of
1a and isatin
2a, catalyzed by
C1, under the previously established conditions. However, the reaction proceeded slowly in THF at −20 °C, giving the desired product
3a in only 8% yield even after 3 days, with moderate stereoselectivities (entry 1,
Table 1). This result was discouraging, but further screenings revealed that the reaction outcome was greatly affected by the reaction solvent, and some typical results are summarized in
Table 1. For example, varying the solvent from THF to ethyl acetate (EtOAc) resulted in a great improvement in stereoselectivities, albeit the yield of
3a was still low (entry 2). When the reaction was run in acetone, the product
3a was obtained in 93% ee and 17
![[thin space (1/6-em)]](https://www.rsc.org/images/entities/char_2009.gif)
:
![[thin space (1/6-em)]](https://www.rsc.org/images/entities/char_2009.gif)
1 dr, with an obviously improved 63% yield (entry 3). The use of MeOH as the solvent further raised the yield to 95%, but the ee value was decreased to 11% (entry 4). Fortunately, both the yield and stereoselectivities of the product
3a were excellent when using MeCN as the solvent (entry 5). In the following, we screened other cinchona alkaloid derivatives
C2–C8, but no improved result was obtained (entries 6–13), and
C1 was still the best in terms of the yield and stereoselectivities. Interestingly, a newly synthesized catalyst
C3, with a
N-tert-butyl group, also achieved 87% ee in this reaction (entry 7). When using
C1 as the catalyst and MeCN as the solvent, improving the usage of silyl enol ether
1a from 1.2 equiv. to 1.5 equiv., the dr and ee values could be slightly raised to 15
![[thin space (1/6-em)]](https://www.rsc.org/images/entities/char_2009.gif)
:
![[thin space (1/6-em)]](https://www.rsc.org/images/entities/char_2009.gif)
1 and 94%.
Table 1 Condition optimizationa
Based on the above screenings, the optimal reaction conditions were determined to be to run the reaction in MeCN at −20 °C, using 10 mol% of the catalyst C1. Under this condition, we then examined the substrate scope with respect to different substituted prochiral fluorinated silyl enol ethers and isatins (Table 2). It was found that under the catalysis of 10 mol% of bifunctional urea C1, isatins 2a–f, without electron-withdrawing substituents, readily reacted with 1a to give the products 3a–f in high to excellent stereoselectivities, up to 15
:
1 dr and 94% ee (entries 1–6).
Table 2 Substrate scope
However, the electron-withdrawing halogen substituents on the framework of isatin greatly diminished the stereoselectivities. Therefore, we further optimized the conditions for the reaction of 5-halogenated isatins 2g–i, and found that the newly prepared urea-tertiary amine catalyst C3 afforded obviously better results for the corresponding reactions,18 and the desired products 3g–i were obtained in up to 86% ee and 5
:
1 dr value (entries 7–9). Noticeably, cinchona alkaloid derived bifunctional (thio)urea catalysts usually bear a N-aryl substituent at the (thio)urea part,17 and to the best of our knowledge, there is no precedent that this type of catalysts bearing a simple N-alkyl group of the urea moiety could achieve a better result than those with a N-aryl group.17h This result suggested that it was worthwhile to synthesize N-alkyl substituted cinchona alkaloid derived bifunctional (thio)urea catalysts.
Likely, the electron-releasing methyl group on the phenyl ring of ether 1 benefited the selectivities, while the electron-withdrawing chloro group destroyed the stereoselectivities (entries 10–11 vs. 12). It seems that the free N–H is essential for good results, as the N-methyl protected isatin 2j gave the product 3n in only 56% yield, 8
:
1 dr and 70% ee. However, α-fluorotetralone derived silyl enol ether 1d proved to be much less reactive than 1a, affording the desired product 3m in only 37% yield, albeit with high ee. The relative and absolute configurations of the product 3h were determined to be (3S, 8S) configuration by X-ray analysis, and those of other products were tentatively assigned by analogy.
Although the use of acyclic monofluorinated silyl enol ether
1e was unable to furnish the tetrasubstituted carbon stereocentre with a fluorine atom, we also examined whether a bifunctional tertiary amine-thiourea catalyst was able to activate it for reaction development. It was found that under the same conditions, the silyl enol ether
1e, obtained as a mixture of
Z/
E isomers with a ratio of 2
![[thin space (1/6-em)]](https://www.rsc.org/images/entities/char_2009.gif)
:
![[thin space (1/6-em)]](https://www.rsc.org/images/entities/char_2009.gif)
1, afforded the desired product
3o in 46% yield, 2
![[thin space (1/6-em)]](https://www.rsc.org/images/entities/char_2009.gif)
:
![[thin space (1/6-em)]](https://www.rsc.org/images/entities/char_2009.gif)
1 dr and 81% ee. The development of a suitable method to prepare such acyclic monofluorinated silyl enol ethers in an excellent
Z/
E ratio, which might influence the dr value of the corresponding products, is now ongoing in our lab.
Interestingly, obvious influences of fluorine substitution were also observed in this reaction: under the same reaction conditions, the monofluorinated silyl enol ether
1a reacted with isatin
2a at a faster rate than the non-fluorinated enol ether
8 (95%
vs. 48% yield), though the product
9 was also obtained in excellent 7
![[thin space (1/6-em)]](https://www.rsc.org/images/entities/char_2009.gif)
:
![[thin space (1/6-em)]](https://www.rsc.org/images/entities/char_2009.gif)
1 dr and 94% ee. On the other hand, α-fluoroindanone
10 turned out to be a much less reactive nucleophile than indanone in this reaction, either at −20 °C or 10 °C, with diminished stereoselectivities. This result justified the use of silyl enol ether
1a for the reaction development.
It should be noted that both prochiral monofluorinated enol silyl ether 1a and difluoroenoxysilane 4 showed obviously higher reactivity than the corresponding non-fluorinated analogues 8 and 6 in the Mukaiyama-aldol reaction of isatins, respectively.19 This result was also in sharp contrast to a recent report by Reissig and Mayr that in the nucleophilic substitution reaction of enol silyl ethers with benzhydrylium ions, the replacement of the phenyl ring of 6 by C6F5 slowed down the reaction by 4.5 orders of magnitude.20 On the other hand, the direct substitution of an α-proton of indanone by fluorine resulted in the greatly diminished reactivity, and we had reported a similar phenomenon that the α-proton of 2,2-difluoro-1-phenylethanone was difficult to be abstracted.15b It is now under investigation in our laboratory why the direct substitution of fluorine at the reactive center could improve the reactivity of silyl enol ethers but reduce the reactivity of ketones.
The thus obtained optically active 3-hydroxyoxindole
3a could be readily reduced to
syn-diol
12 by NaBH
4, featuring three consecutive stereogenic centers, in 95% yield and 95% ee value, and the relative configuration was confirmed by X-ray analysis. It should be noted that only relative configuration could be assigned by using the single crystal of
syn-
12, due to the lack of heavy atoms. Interestingly, the synthesis of its diastereoisomer
anti-diol
12 was achieved just by varying the solvent from THF–AcOH to THF–MeOH for the reduction by NaBH
4. This represents an expedient and useful method for the preparation of oxindole based chiral fluorinated 1,3-diol compounds as the selectivity could be controlled by the choice of the solvent.
Conclusions
In summary, we have developed an unprecedented highly stereoselective Mukaiyama-aldol reaction of prochiral fluorinated silyl enol ethers and isatins to furnish 3-hydroxyoxindoles with two continuous tetrasubstituted stereogenic carbon centres. The thus obtained optically active hydroxyoxindoles,21 featuring a tertiary α-fluoroketone moiety, are interesting for medicinal studies. Importantly, the identification of nucleophilic tertiary amine catalysis to activate monofluorinated silyl enol ethers offers the promise to develop new asymmetric nucleophilic fluorination reactions. In addition, our results also suggested that cinchona alkaloid derived bifunctional (thio)urea catalysts with a simple N-alkyl substituent in the urea moiety are worthwhile to explore, which is now in progress in our lab.
Financial support from NSFC (21172075, 21222204), the Ministry of Education (NCET-11-0147 and PCSIRT) and the Program of SSCS (13XD1401600) and Innovation Program of SMEC (12ZZ046) is appreciated.
Notes and references
- For selected examples:
(a) S. O. Thomas, V. L. R. Singleton, J. A. Lowery, R. W. Sharpe, L. M. Pruess, J. N. Porter, J. H. Mowat and N. Bohonos, Antibiot. Annu., 1956–1957, 716 Search PubMed;
(b) M. O. F. Khan and H. J. Lee, Chem. Rev., 2008, 108, 5131 CrossRef CAS PubMed;
(c) P. Hewawasam, V. K. Gribkoff, Y. Pendri, S. I. Dworetzky, N. A. Meanwell, E. Martinez, C. G. Boissard, D. J. Post-Munson, J. T. Trojnacki, K. Yeleswaram, L. M. Pajor, J. Knipe, Q. Gao, R. Perrone and J. E. Starrett Jr., Bioorg. Med. Chem. Lett., 2002, 12, 1023 CrossRef CAS;
(d) E. David, G. Milanole, P. Ivashkin, S. Couve-Bonnaire, P. Jubault and X. Pannecoucke, Chem. – Eur. J., 2012, 18, 14904 CrossRef CAS PubMed;
(e)
I. Ojima, Fluorine in Medicinal Chemistry and Chemical Biology, Wiley-Blackwell, New York, 2009 Search PubMed.
-
(a) E. J. Corey and A. Guzman-Perez, Angew. Chem., Int. Ed., 1998, 37, 388 CrossRef;
(b) J. Christoffers and A. Mann, Angew. Chem., Int. Ed., 2001, 40, 4591 CrossRef CAS;
(c) I. Denissova and L. Barriault, Tetrahedron, 2003, 59, 10105 CrossRef CAS PubMed;
(d) V. Farina, J. T. Reeves, C. H. Senanayake and J. J. Song, Chem. Rev., 2006, 106, 2734 CrossRef CAS PubMed;
(e) P. G. Cozzi, R. Hilgraf and N. Zimmermann, Eur. J. Org. Chem., 2007, 5969 CrossRef CAS;
(f) M. Shibasaki and M. Kanai, Chem. Rev., 2008, 108, 2853 CrossRef CAS PubMed;
(g) O. Riant and J. Hannedouche, Org. Biomol. Chem., 2007, 5, 873 RSC;
(h) Z.-L. Song, C.-A. Fan and Y.-Q. Tu, Chem. Rev., 2011, 111, 7523 CrossRef CAS PubMed;
(i) J. Zhou, L. Chen, X.-P. Yin and C.-H. Wang, Org. Biomol. Chem., 2014 10.1039/C4OB00718B.
-
(a) M. Schlosser, Angew. Chem., Int. Ed., 1998, 37, 1496 CrossRef CAS;
(b) B. E. Smart, J. Fluorine Chem., 2001, 109, 3 CrossRef CAS;
(c) K. Mikami, Y. Itoh and M. Yamanaka, Chem. Rev., 2004, 104, 1 CrossRef CAS PubMed;
(d) C. Pesenti and F. Viani, ChemBioChem, 2004, 5, 590 CrossRef CAS PubMed;
(e) N. Shibata, S. Mizuta and H. Kawai, Tetrahedron: Asymmetry, 2008, 19, 2633 CrossRef CAS PubMed;
(f) D. O'Hagan, Chem. Soc. Rev., 2008, 37, 308 RSC;
(g) J. Hu, W. Zhang and F. Wang, Chem. Commun., 2009, 7465 RSC;
(h) L. Hunter, Beilstein J. Org. Chem., 2010, 6 DOI:10.3762/bjoc.6.38;
(i) J. Nie, H.-C. Guo, D. Cahard and J.-A. Ma, Chem. Rev., 2011, 111, 455 CrossRef CAS PubMed;
(j) G. Valero, X. Companyó and R. Rios, Chem. – Eur. J., 2011, 17, 2018 CrossRef CAS PubMed;
(k) Y.-L. Liu, J.-S. Yu and J. Zhou, Asian J. Org. Chem., 2013, 2, 194 CrossRef CAS;
(l) F. Pan and Z. Shi, Acta Chim. Sin., 2012, 70, 1679 CAS;
(m) J.-A. Ma and S. Li, Org. Chem. Front., 2014, 1 10.1039/C4QO00078A.
- For a general review: D. Cahard, X. Xu, S. Couve-Bonnaire and X. Pannecoucke, Chem. Soc. Rev., 2010, 39, 558 RSC.
- For reviews:
(a) S. Lectard, Y. Hamashima and M. Sodeoka, Adv. Synth. Catal., 2010, 352, 2708 CrossRef CAS;
(b) V. A. Brunet and D. O'Hagan, Angew. Chem., Int. Ed., 2008, 47, 1179 CrossRef CAS PubMed;
(c) S. D. Taylor, C. C. Kotoris and G. Hum, Tetrahedron, 1999, 55, 12431 CrossRef CAS;
(d) R. P. Singh and J. M. Shreeve, Acc. Chem. Res., 2004, 37, 31 CrossRef CAS PubMed;
(e) V. D. Romanenko and V. P. Kukhar, Chem. Rev., 2006, 106, 3868 CrossRef CAS PubMed;
(f) M. Tredwell and V. Gouverneur, Org. Biomol. Chem., 2006, 4, 26 RSC;
(g) J.-B. Xia, Y. Ma and C. Chen, Org. Chem. Front., 2014, 1, 468 RSC.
- For reviews:
(a) Y. Zhao, Y. Pan, S.-B. D. Sim and C.-H. Tan, Org. Biomol. Chem., 2012, 10, 479 RSC;
(b) W. B. Farnham, Chem. Rev., 1996, 96, 1633 CrossRef CAS PubMed; for the syntheses of fluorinated tertiary chiral carbons by fluoroacetone:
(c) G. Zhong, J. Fan and C. F. Barbas III, Tetrahedron Lett., 2004, 45, 5681 CrossRef CAS PubMed;
(d) X.-Y. Xu, Y.-Z. Wang and L.-Z. Gong, Org. Lett., 2007, 9, 4247 CrossRef CAS PubMed; by α-fluoromalonate:
(e) X. Companyó, M. Hejnová, M. Kamlar, J. Vesely, A. Moyano and R. Rios, Tetrahedron Lett., 2009, 50, 5021 CrossRef PubMed; by α-fluorinated β-keto gem-diols:
(f) C. Wu, G. Li, W. Sun, M. Zhang, L. Hong and R. Wang, Org. Lett., 2014, 16, 1960 CrossRef CAS PubMed;
(g) I. Saidalimu, X. Fang, X.-P. He, J. Liang, X. Yang and F. Wu, Angew. Chem., Int. Ed., 2013, 52, 5566 CrossRef CAS PubMed; by 1-fluorobis(phenylsulfonyl)methane:
(h) S. Mizuta, N. Shibata, Y. Goto, T. Furukawa, S. Nakamura and T. Toru, J. Am. Chem. Soc., 2007, 129, 6394 CrossRef CAS PubMed;
(i) T. Furukawa, N. Shibata, S. Mizuta, S. Nakamura, T. Toru and M. Shiro, Angew. Chem., Int. Ed., 2008, 47, 8051 CrossRef CAS PubMed.
- For leading examples of electrophilic fluorination to tetrasubstituted stereogenic carbon centers:
(a) L. Hintermann and A. Togni, Angew. Chem., Int. Ed., 2000, 39, 4359 CrossRef CAS;
(b) N. Shibata, J. Kohno, K. Takai, T. Ishimaru, S. Nakamura, T. Toru and S. Kanemasa, Angew. Chem., Int. Ed., 2005, 44, 4204 CrossRef CAS PubMed;
(c) Y. Hamashima, T. Suzuki, H. Takano, Y. Shimura and M. Sodeoka, J. Am. Chem. Soc., 2005, 127, 10164 CrossRef CAS PubMed;
(d) K. Shibatomi and H. Yamamoto, Angew. Chem., Int. Ed., 2008, 47, 5796 CrossRef CAS PubMed;
(e) M. Nakamura, A. Hajra, K. Endo and E. Nakamura, Angew. Chem., Int. Ed., 2005, 44, 7248 CrossRef CAS PubMed;
(f) J. Nie, H.-W. Zhu, H.-F. Cui, M.-Q. Hua and J.-A. Ma, Org. Lett., 2007, 9, 3053 CrossRef CAS PubMed;
(g) P. Y. Toullec, C. Bonaccorsi, A. Mezzetti and A. Togni, Proc. Natl. Acad. Sci. U. S. A., 2004, 101, 5810 CrossRef CAS PubMed;
(h) S. M. Kim, H. R. Kim and D. Y. Kim, Org. Lett., 2005, 7, 2309 CrossRef CAS PubMed;
(i) X. Wang, Q. Lan, S. Shirakawa and K. Maruoka, Chem. Commun., 2010, 46, 321 RSC;
(j) S. Brandes, B. Niess, M. Bella, A. Prieto, J. Overgaard and K. A. Jørgensen, Chem. – Eur. J., 2006, 12, 6039 CrossRef CAS PubMed;
(k) H.-F. Wang, H.-F. Cui, Z. Chai, P. Li, C.-W. Zheng, Y.-Q. Yang and G. Zhao, Chem. – Eur. J., 2009, 15, 13299 CrossRef CAS PubMed;
(l) J. T. Mohr, D. C. Behenna, A. M. Harned and B. M. Stoltz, Angew. Chem., Int. Ed., 2005, 44, 6924 CrossRef CAS PubMed;
(m) A. Tarui, H. Nishimura, T. Ikebata, A. Tahira, K. Sato, M. Omote, H. Minami, Y. Miwa and A. Ando, Org. Lett., 2014, 16, 2080 CrossRef CAS PubMed.
-
(a) S. Arai, M. Oku, T. Ishida and T. Shioiri, Tetrahedron Lett., 1999, 40, 6785 CrossRef CAS;
(b) B. Thierry, T. Perrard, C. Audouard, J.-C. Plaquevent and D. Cahard, Synthesis, 2001, 1742 CrossRef CAS;
(c) Y. Zhao, Y. Pan, H. Liu, Y. Yang, Z. Jiang and C.-H. Tan, Chem. – Eur. J., 2011, 17, 3571 CrossRef CAS PubMed.
-
(a) R. Frantz, L. Hintermann, M. Perseghini, D. Broggini and A. Togni, Org. Lett., 2003, 5, 1709 CrossRef CAS PubMed;
(b) R. He, X. Wang, T. Hashimoto and K. Maruoka, Angew. Chem., Int. Ed., 2008, 47, 9466 CrossRef CAS PubMed;
(c) X. Han, J. Kwiatkowski, F. Xue, K.-W. Huang and Y. Lu, Angew. Chem., Int. Ed., 2009, 48, 7604 CrossRef CAS PubMed;
(d) H. Li, S. Zhang, C. Yu, X. Song and W. Wang, Chem. Commun., 2009, 2136 RSC;
(e) Z. Jiang, Y. Pan, Y. Zhao, T. Ma, R. Lee, Y. Yang, K.-W. Huang, M. W. Wong and C.-H. Tan, Angew. Chem., Int. Ed., 2009, 48, 3627 CrossRef CAS PubMed;
(f) H.-F. Cui, Y.-Q. Yang, Z. Chai, P. Li, C.-W. Zheng, S.-Z. Zhu and G. Zhao, J. Org. Chem., 2010, 75, 117 CrossRef CAS PubMed.
- É. Bélanger, K. Cantin, O. Messe, M. Tremblay and J.-F. Paquin, J. Am. Chem. Soc., 2007, 129, 1034 CrossRef PubMed.
-
(a) Y. Pan, Y. Zhao, T. Ma, Y. Yang, H. Liu, Z. Jiang and C.-H. Tan, Chem. – Eur. J., 2010, 16, 779 CrossRef CAS PubMed;
(b) G. K. S. Prakash, F. Wang, T. Stewart, T. Mathew and G. A. Olah, Proc. Natl. Acad. Sci. U. S. A., 2009, 106, 4090 CrossRef CAS PubMed.
- X. Dou and Y. Lu, Org. Biomol. Chem., 2013, 11, 5217 CAS.
-
(a) G. K. S. Prakash and P. Beier, Angew. Chem., Int. Ed., 2006, 45, 2172 CrossRef CAS PubMed;
(b) P. M. Pihko, Angew. Chem., Int. Ed., 2006, 45, 544 CrossRef CAS PubMed.
-
(a) Z.-Y. Cao, X. Wang, C. Tan, X.-L. Zhao, J. Zhou and K. Ding, J. Am. Chem. Soc., 2013, 135, 8197 CrossRef CAS PubMed;
(b) F. Zhou, C. Tan, J. Tang, Y.-Y. Zhang, W.-M. Gao, H.-H. Wu, Y.-H. Yu and J. Zhou, J. Am. Chem. Soc., 2013, 135, 10994 CrossRef CAS PubMed;
(c) Y.-L. Liu, B.-L. Wang, J.-J. Cao, L. Chen, Y.-X. Zhang, C. Wang and J. Zhou, J. Am. Chem. Soc., 2010, 132, 15176 CrossRef CAS PubMed;
(d) M. Ding, F. Zhou, Y.-L. Liu, C.-H. Wang, X.-L. Zhao and J. Zhou, Chem. Sci., 2011, 2, 2035 RSC;
(e) Z.-Y. Cao, F. Zhou, Y.-H. Yu and J. Zhou, Org. Lett., 2013, 15, 42 CrossRef CAS PubMed;
(f) Z.-Y. Cao, Y. Zhang, C.-B. Ji and J. Zhou, Org. Lett., 2011, 13, 6398 CrossRef CAS PubMed;
(g) Y.-L. Liu and J. Zhou, Chem. Commun., 2013, 49, 4421 RSC;
(h) F. Zhou, X.-P. Zeng, C. Wang, X.-L. Zhao and J. Zhou, Chem. Commun., 2013, 49, 2022 RSC;
(i) Y.-H. Wang, Z.-Y. Cao, Y.-F. Niu, X.-L. Zhao and J. Zhou, Acta Chim. Sin., 2014, 72 DOI:10.6023/A14040335.
-
(a) Y.-L. Liu, X. Wang, Y.-L. Zhao, F. Zhu, X.-P. Zeng, L. Chen, C.-H. Wang, X.-L. Zhao and J. Zhou, Angew. Chem., Int. Ed., 2013, 52, 13735 CrossRef CAS PubMed;
(b) Y.-L. Liu and J. Zhou, Chem. Commun., 2012, 48, 1919 RSC;
(c) Y.-L. Liu and J. Zhou, Acta Chim. Sin., 2012, 70, 1451 CAS;
(d) Y.-L. Liu, T.-D. Shi, F. Zhou, X.-L. Zhao, X. Wang and J. Zhou, Org. Lett., 2011, 13, 3826 CrossRef CAS PubMed;
(e) Y.-L. Liu, X.-P. Zeng and J. Zhou, Chem. – Asian. J., 2012, 7, 1759 CrossRef CAS PubMed;
(f) L. Chen, T.-D. Shi and J. Zhou, Chem. – Asian. J., 2013, 8, 556 CrossRef CAS PubMed.
- For nucleophilic tertiary amine catalysis:
(a) S. E. Denmark and G. L. Beutner, Angew. Chem., Int. Ed., 2008, 47, 1560 CrossRef CAS PubMed;
(b) T.-Y. Liu, M. Xie and Y.-C. Chen, Chem. Soc. Rev., 2012, 41, 4101 RSC.
- For reviews on cinchona alkaloid derivatives, see:
(a) E. M. O. Yeboah, S. O. Yeboah and G. S. Singh, Tetrahedron, 2011, 67, 1725 CrossRef CAS PubMed;
(b) T. Marcelli and H. Hiemstra, Synthesis, 2010, 1229 CrossRef CAS PubMed;
(c) S. J. Connon, Chem. Commun., 2008, 2499 RSC. For pioneering work on the cinchona alkaloid thiourea catalyst, see:
(d) B.-J. Li, L. Jiang, M. Liu, Y.-C. Chen, L.-S. Ding and Y. Wu, Synlett, 2005, 603 CAS;
(e) B. Vakulya, S. Varga, A. Csámpai and T. Soós, Org. Lett., 2005, 7, 1967 CrossRef CAS PubMed;
(f) S. H. McCooey and S. J. Connon, Angew. Chem., Int. Ed., 2005, 44, 6367 CrossRef CAS PubMed;
(g) J. Ye, D. J. Dixon and P. S. Hynes, Chem. Commun., 2005, 4481 RSC. As far as
we knew, only a N-cyclohexyl substituted cinchona alkaloid bifunctional thiourea catalyst had been tried:
(h) B. Vakulya, S. Varga and T. Soós, J. Org. Chem., 2008, 73, 3475 CrossRef CAS PubMed.
- In the reaction of silyl enol ether 1a and 5-bromoisatin 2i, the use of the catalyst C1 afforded the desired product 3i in lower yield (78%) and ee value (81%), albeit with a slightly better dr value (6.6
:
1).
- As far as the reactivity comparison of difluoroenoxysilane 4 and its non-fluorinated analogue 6 is concerned, it seemed to be dependent on reaction conditions: 6 was two times more reactive than 4 in a Pd-catalyzed arylation reaction:
(a) Y. Guo and J. M. Shreeve, Chem. Commun., 2007, 3583 RSC; but was less reactive in an acid catalyzed Mannich reaction:
(b) W. Kashikura, K. Mori and T. Akiyama, Org. Lett., 2011, 13, 1860 CrossRef CAS PubMed.
- H. A. Laub, D. Gladow, H.-U. Reissig and H. Mayr, Org. Lett., 2012, 14, 3990 CrossRef CAS PubMed.
- For reviews:
(a) F. Zhou, Y.-L. Liu and J. Zhou, Adv. Synth. Catal., 2010, 352, 1381 CrossRef CAS;
(b) K. Shen, X. Liu, L. Lin and X. Feng, Chem. Sci., 2012, 3, 327 RSC;
(c) R. Dalpozzo, G. Bartoli and G. Bencivenni, Chem. Soc. Rev., 2012, 41, 7247 RSC;
(d) A. Kumar and S. S. Chimni, RSC Adv., 2012, 2, 9748 RSC;
(e) Y.-L. Liu, F. Zhu, C.-H. Wang and J. Zhou, Chin. J. Org. Chem., 2013, 33, 1595 CrossRef CAS;
(f) S. Mohammadi, R. Heiran, R. P. Herrera and E. Marqués-López, ChemCatChem, 2013, 5, 2131 CrossRef CAS;
(g) Z.-Y. Cao, Y.-H. Wang, X.-P. Zeng and J. Zhou, Tetrahedron Lett., 2014, 55, 2571 CrossRef CAS PubMed. For selected examples, see:
(h) K. Zheng, C. Yin, X. Liu, L. Lin and X. Feng, Angew. Chem., Int. Ed., 2011, 50, 2573 CrossRef CAS PubMed;
(i) P. Chauhan and S. S. Chimni, Chem. – Eur. J., 2010, 16, 7709 CrossRef CAS PubMed;
(j) X.-N. Wang, Y.-Y. Zhang and S. Ye, Adv. Synth. Catal., 2010, 352, 1892 CrossRef CAS;
(k) Q. Guo, M. Bhanushali and C.-G. Zhao, Angew. Chem., Int. Ed., 2010, 49, 9460 CrossRef CAS PubMed;
(l) X.-Y. Guan, Y. Wei and M. Shi, Chem. – Eur. J., 2010, 16, 13617 CrossRef CAS PubMed;
(m) T. Bui, N. R. Candeias and C. F. Barbas III, J. Am. Chem. Soc., 2010, 132, 5574 CrossRef CAS PubMed;
(n) Z. Zhang, W. Zheng and J. C. Antilla, Angew. Chem., Int. Ed., 2011, 50, 1135 CrossRef CAS PubMed;
(o) L. Liu, S. Zhang, F. Xue, G. Lou, H. Zhang, S. Ma, W. Duan and W. Wang, Chem. – Eur. J., 2011, 17, 7791 CrossRef CAS PubMed;
(p) N. V. Hanhan, Y. C. Tang, N. T. Tran and A. K. Franz, Org. Lett., 2012, 14, 2218 CrossRef CAS PubMed;
(q) G.-G. Liu, H. Zhao, Y.-B. Lan, B. Wu, X.-F. Huang, J. Chen, J.-C. Tao and X.-W. Wang, Tetrahedron, 2012, 68, 3843 CrossRef CAS PubMed;
(r) H. Liu, H. Wu, Z. Luo, J. Shen, G. Kang, B. Liu, Z. Wan and J. Jiang, Chem. – Eur. J., 2012, 18, 11899 CrossRef CAS PubMed.
Footnote |
† Electronic supplementary information (ESI) available. CCDC 999612 and 999613. For ESI and crystallographic data in CIF or other electronic format see DOI: 10.1039/c4qo00126e |
|
This journal is © the Partner Organisations 2014 |