DOI:
10.1039/C4QO00026A
(Tutorial Account)
Org. Chem. Front., 2014,
1, 587-595
The highly enantioselective catalytic aza-Morita–Baylis–Hillman reaction†
Received
23rd January 2014
, Accepted 7th March 2014
First published on 13th March 2014
Abstract
The highly enantioselective aza-Morita–Baylis–Hillman (aza-MBH) reaction is one of the most important reactions for the synthesis of optically active α-methylene-β-amino carbonyl compounds. The use of chiral phosphines or amines as organocatalysts can be envisaged for this catalytic asymmetric reaction. This mini review focuses on the important developments with regard to asymmetric aza-MBH reactions catalyzed by chiral phosphines or amines or even organometallic complexes in recent decades and also on the perspectives that these new developments offer.
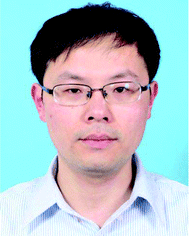 Fang-Le Hu | Fang-Le Hu was born in 1986 in Jiangsu (P. R. China). He received his BS degree in 2009 from the Shanghai University before joining a PhD program in the East China University of Science & Technology. He is currently a PhD candidate under the direction of Professor Min Shi. |
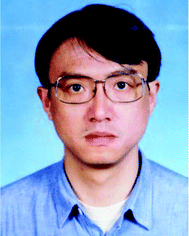 Min Shi | Dr Min Shi was born in 1963 in Shanghai, China. He received his BS in 1984 (Institute of Chemical Engineering of East China) and PhD in 1991 (Osaka University, Japan). He gained postdoctoral research experience with Prof. Kenneth M. Nicholas at University of Oklahoma (1995–6) and worked as an ERATO Researcher in Japan Science and Technology Corporation (JST) (1996–8). He is currently a group leader in the State Key Laboratory of Organometallic Chemistry, Shanghai Institute of Organic Chemistry (SIOC). |
1. Introduction
The carbon–carbon bond forming reaction is one of the most fundamental reactions in organic synthesis due to its pivotal role in building up various classes of carbon frameworks. Thus, it has been an important challenging and fascinating topic in organic chemistry.1 Among the diverse carbon–carbon bond forming reactions, the Morita–Baylis–Hillman (MBH) reaction has received remarkable and increasing interest since it is well equipped with the important concepts of atom economy and organocatalysis. The classical MBH reaction can be accomplished by addition of α,β-unsaturated carbonyl compounds to aldehydes in the presence of tertiary phosphine or amine, giving densely functionalized α-methylene-β-hydroxycarbonyl compounds (Scheme 1, X = O). Instead of aldehydes, imines are also suitable for this reaction if they can be appropriately activated, leading to α-methylene-β-amino carbonyl compounds smoothly, and the process of this case is commonly referred to as the aza-Morita–Baylis–Hillman (aza-MBH) reaction. The origin of the Morita–Baylis–Hillman reaction can be traced back to a pioneering report presented in 1968 by Morita (phosphine catalyzed reaction)2 and later Baylis and Hillman described a similar amine catalyzed reaction in 1972.3 However, it has been ignored by organic chemists for almost a decade after its discovery. Since the mid-1990s, more and more research groups have initiated their work on different facets of this reaction, involving the scope of the substrates, novel catalysts including chiral catalysts, understanding the mechanism and various synthetic applications of MBH adducts.4 In this mini review, we wish to discuss organocatalytic or organometallic complex-catalyzed asymmetric aza-MBH reactions briefly and we hope that this article can also direct the reader to several exhaustive reviews that have been published for more detailed information.
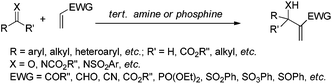 |
| Scheme 1 Tertiary amine or phosphine-catalyzed MBH or aza-MBH reaction. | |
2. Asymmetric aza-MBH reactions of aldimines
2.1 Amine-catalyzed asymmetric aza-MBH reactions
The chiral tertiary amine catalysts based on the quinidine framework such as β-ICD for asymmetric MBH/aza-MBH reactions have been intensively investigated. In 1999, Hatakeyama and co-workers employed a modified cinchona alkaloid β-ICD as the base-catalyst for the first highly enantioselective organocatalyzed MBH reaction of aliphatic aldehydes with the highly reactive Michael acceptor, 1,1,1,3,3,3-hexafluoroisopropyl acrylate.5a This important finding then sparked the catalytic asymmetric MBH reactions. In 2002, we reported the first example of highly enantioselective aza-MBH reactions of aromatic aldimines with MVK (methyl vinyl ketone)/methyl acrylate/acrylonitrile catalyzed by β-ICD, thus providing by far the highest ee values for the aza-MBH reaction (Scheme 2).5b Concerning aliphatic imines, however, complicated unidentified products rather than normal aza-MBH adducts were obtained under the standard conditions.
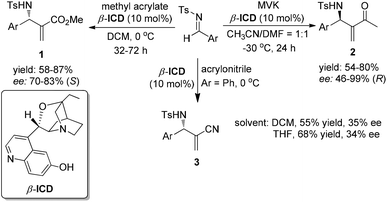 |
| Scheme 2 Asymmetric aza-MBH reaction of N-tosylimines with MVK/methyl acrylate/acrylonitrile catalyzed by β-ICD. | |
Hatakeyama and co-workers almost simultaneously reported the β-ICD-catalyzed aza-MBH reaction of various aryl diphenylphosphinoyl imines 4 with 1,1,1,3,3,3-hexafluoroisopropyl acrylate (HFIPA) 5 in DMF at low temperature, producing (S)-adducts 6 in up to 97% yields with high ee values, in contrast to the MBH reactions of aldehydes with 5, which afford (R)-selectivity.6a Moreover, to demonstrate the synthetic utility of the products, sequences of transformations were conducted for the synthesis of β-lactam 7 (Scheme 3).6b
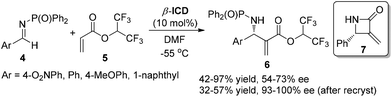 |
| Scheme 3 β-ICD-catalyzed aza-MBH reaction of 4 with 5. | |
Soon after Shi's report, Adolfsson and co-workers demonstrated the use of the chiral quinuclidine-derivative β-ICD as a catalyst in the one-pot, three-component aza-MBH reaction, leading to the desired products in moderate to good yields with high ee values (Scheme 4).7
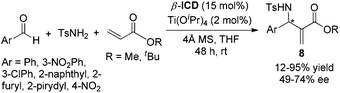 |
| Scheme 4 β-ICD-catalyzed three-component aza-MBH reaction. | |
Due to the fact that a different stereochemistry for the aza-MBH reaction involving different Michael acceptors was observed,5 we reinvestigated systematically the reaction of N-sulfonated imines with different activated olefins. It was found that the aza-MBH reaction of N-sulfonated imines with phenyl acrylate, α-naphthyl acrylate, acrolein or acrylonitrile catalyzed by β-ICD afforded (S)-enriched adducts 10, 11 and 12, respectively (Scheme 5).8 Acrylonitrile is less reactive than acrolein, phenyl acrylate and α-naphthyl acrylate, and a higher temperature (0 °C) is required for its reaction, giving the desired products 12 in lower yields (43–55%) and moderate ee values (35–40%).
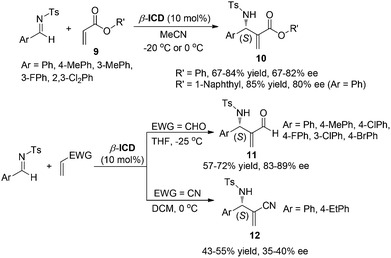 |
| Scheme 5 β-ICD-catalyzed aza-MBH reactions of N-sulfonated imines with acrylates, acrolein or acrylonitrile. | |
Notably, when methyl or ethyl vinyl ketone was subjected to this reaction in DMF–MeCN (1
:
1) mixtures at low temperature (−30 °C), (R)-adducts were observed, which is opposite to the aza-MBH reaction of N-sulfonated imines with phenyl acrylate, α-naphthyl acrylate, acrolein or acrylonitrile. N-Mesyl or N-SES-protected imines gave similar results (Scheme 6).8
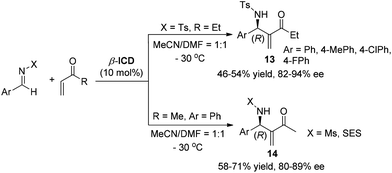 |
| Scheme 6 β-ICD-catalyzed aza-MBH reactions of imines with alkyl vinyl ketones. | |
In 2005, Sasai and co-workers designed and synthesized an efficient and novel bifunctional organocatalyst 15 for the enantioselective aza-MBH reaction for the first time. They found that the reaction outcomes were deeply influenced by the position of the Lewis base attached to BINOL and the acid–base-mediated functionalities for the activation of the substrate and the fixing of conformation of the organocatalyst are harmoniously performed to promote the reaction with high enantiocontrol (Scheme 7).9
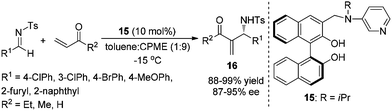 |
| Scheme 7 Bifunctional organocatalysts for the asymmetric aza-MBH reaction. | |
Although β-ICD was demonstrated to be an efficient catalyst in the aza-MBH reaction, the substrate scopes are limited to arylaldimines. To resolve this problem, Masson and Zhu et al. developed a novel bifunctional catalyst 17 derived from β-ICD, which in combination with β-naphthol served as a highly effective dual catalyst for the asymmetric aza-MBH reaction, leading to the corresponding adducts in high yields and enantioselectivities in most cases of aromatic imines.10 As for aliphatic N-sulfonated imines, the reactions could also proceed smoothly to give the desired products in moderate yields (38–57%) with high ee values (84–87%) for the first time. It was assumed that the pairing of cooperative H-bonds is important and nucleophilic addition of the (Z)-enolate onto the Re-face of the (E)-imine via the less crowded transition state TS-1 was proposed to account for the observed (S)-enantioselectivity in the adduct 19 (Scheme 8).10
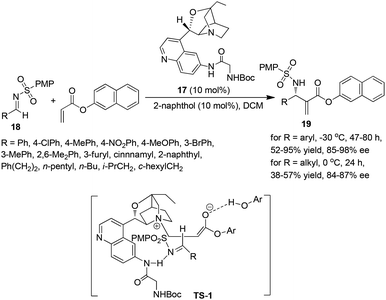 |
| Scheme 8 Asymmetric aza-MBH reactions of imines 18 with β-naphthyl acrylate. | |
On the basis of the above mechanistic assumption, the author assumed that this dual catalytic system should favor the (S)-aza-MBH product regardless of the nature of the Michael acceptor used. Therefore, they developed a new β-ICD-amide catalyst 20 to investigate the reaction between N-tosylimine and alkyl vinyl ketone and found that an achiral protic additive was capable of inversing the β-ICD and β-ICD-amide catalyzed enantioselective aza-MBH reaction between N-sulfonylimines and MVK/EVK, thereby providing another solution to the enantio-complementarity associated with this family of catalysts (Scheme 9).11
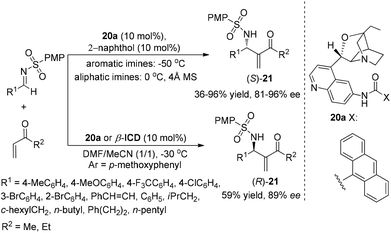 |
| Scheme 9 Asymmetric aza-MBH reactions of N-sulfonated imines with alkyl vinyl ketones. | |
Subsequently, Zhu's group reported another β-ICD-amide catalyzed and β-naphthol co-catalyzed aza-MBH reaction using readily available α-amidosulfones 22 as substrates to afford uniformly the (S)-adducts 23 in high yields and excellent enantioselectivities (Scheme 10).12 At almost the same time, we demonstrated a similar asymmetric aza-MBH reaction of N-protected imines 24 or N-protected α-amidoalkyl phenyl sulfones 22 with MVK or EVK catalyzed by β-ICD, affording highly enantioselective aza-MBH products 25 in good yields with high enantioselectivities (Scheme 11).13
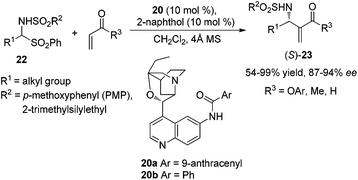 |
| Scheme 10 Asymmetric aza-MBH reaction of 22 with activated olefins. | |
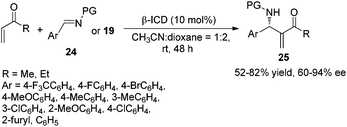 |
| Scheme 11 Asymmetric aza-MBH reactions of imines with MVK or EVK catalyzed by β-ICD. | |
Chiral thiourea is also an efficient catalyst for the aza-MBH reaction in the presence of an achiral nucleophilic additive. Nagasawa and coworkers first reported a highly efficient chiral thiourea catalyst for the enantioselective MBH reaction in 2004.14a Subsequently, Jacobsen and coworkers reported a chiral thiourea catalyst 26 combined with a stoichiometric amount of DABCO for a highly enantioselective aza-MBH reaction of nosylimines with methyl acrylate, affording the desired products in high ee values (Scheme 12).14b
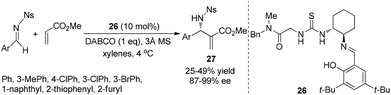 |
| Scheme 12 Highly enantioselective aza-MBH reaction catalyzed by chiral thiourea and DABCO. | |
2.2 Phosphine-catalyzed asymmetric aza-MBH reactions
Chiral phosphines have been intensively used as efficient organocatalysts in MBH/aza-MBH reactions.15 In 2003, we first demonstrated that chiral LBBA (Lewis base and Brønsted acid) bifunctional phosphine CP1 derived from 1,1′-bi-2,2′-naphthol (BINOL) could catalyze the aza-MBH reaction of N-tosylimines with activated olefins effectively, affording the corresponding adducts 28, 29, 30 and 31 in good yields with high ee values, respectively (Scheme 13).16 The addition of molecular sieves increased chemical yields because they could remove the ambient moisture that caused the decomposition of N-sulfonated imines. It was found that the presence of a phenolic hydroxyl group in the catalyst CP1 plays a crucial role in this reaction and the phosphine catalyst without a phenol moiety could not catalyze this reaction smoothly. We have proposed a detailed mechanism to rationalize the stereochemistry of the produced adducts. The reaction might be initiated by nucleophilic addition of the phosphorus centre in the catalyst CP1 to MVK, and the phenolic OH group acts as a Brønsted acid to stabilize the in situ formed key zwitterionic intermediate A and reaction intermediate B through hydrogen bonds. Subsequent hydrogen transfer and β-elimination produces the desired products (Scheme 14).16 Notably, the key enolate intermediate A, which was stabilized by intramolecular hydrogen bonding, has been observed by 31P and 1H NMR spectroscopy. During the investigations on the aza-MBH reaction, we found that the catalyst CP1 also demonstrated good asymmetric induction for the aza-MBH reaction of ethyl (arylimino)acetates with MVK or EVK under mild conditions to give the corresponding adducts in moderate to high yields as well as good to high enantioselectivities;17 however, catalyst CP1 could not give good enantiomeric excess in the reaction of N-(arylmethylene)diphenylphosphinamides with various activated olefins such as phenyl acrylate, acrylonitrile or MVK.18
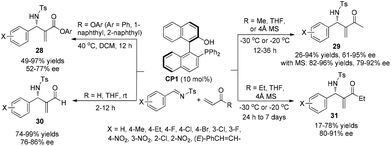 |
| Scheme 13
CP1-catalyzed asymmetric aza-MBH reactions. | |
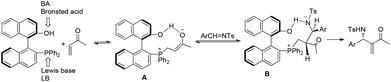 |
| Scheme 14 Detailed mechanism of aza-MBH reaction catalyzed by CP1. | |
Having identified CP1 as an effective catalyst for the aza-MBH reaction,16 we envisaged that replacing the phenol group in the catalyst CP1 with other groups such as a (thio-)urea might also give good reaction outcomes, because acidic NH protons are good hydrogen-bonding donors for hydrogen bond formation, which can also stabilize similar intermediates in the aza-MBH reaction.19 As hypothesized, the chiral phosphine-thiourea CP2 in combination with benzoic acid indeed proved to be a very successful catalytic system for the aza-MBH reaction of N-tosylimines with MVK, EVK, PVK or acrolein; 67–97% ee and 61–98% yields of the corresponding adducts 32 were obtained (Scheme 15).20 To the best of our knowledge, this was the first report on the synthesis and application of chiral phosphine-thiourea catalysts in asymmetric catalysis.
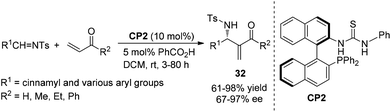 |
| Scheme 15
CP2-catalyzed asymmetric aza-MBH reactions. | |
Subsequently, to further improve the catalytic activity and enantioselectivity, we developed a series of bifunctional chiral phosphine-amide catalysts,21 and found that the catalyst CP3 with a moderate acidic amide proton displayed the best asymmetric induction for the aza-MBH reaction of N-sulfonated imines with MVK or EVK (Scheme 16).21 We also designed and synthesized sterically congested bifunctional chiral phosphine-amide catalysts22 and investigated their application in the asymmetric aza-MBH reactions of N-sulfonated imines with MVK or EVK under mild conditions. The corresponding aza-MBH adducts 33 can be obtained in good-to-excellent yields and moderate-to-good enantioselectivities.22
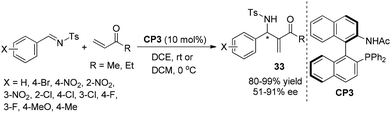 |
| Scheme 16
CP3-catalyzed asymmetric aza-MBH reactions. | |
Inspired by the observation that long perfluoroalkane chains, so called “pony” tails, in a variety of chiral ligands can improve the enantioselectivities under identical conditions,23 we also synthesized chiral phosphine Lewis bases CP4 and CP5 bearing long perfluoroalkane chains as “pony tails” and investigated their performance in the catalytic asymmetric aza-MBH reaction. Indeed, the catalyst CP5 was more effective in the aza-MBH reaction of N-sulfonated imines with MVK than the previously reported chiral phosphine CP1. The performance of the catalyst CP4 was not so impressive presumably due to the steric bulkiness (Scheme 17).24
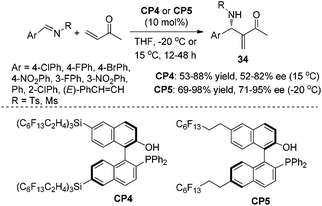 |
| Scheme 17 CP4 or CP5-catalyzed aza-MBH reactions. | |
In our previous report of chiral phosphine Lewis base CP1-catalyzed asymmetric aza-MBH reactions, we also disclosed that a phenolic hydroxy group played a key role in this bifunctional organocatalyst, with intramolecular hydrogen bonding affording the corresponding aza-MBH adduct in high ee.16 We envisioned that increasing the number of hydrogen bond donating groups can significantly stabilize the key phosphonium enolate and produce the corresponding adducts in good yields and high ee. Herein, we synthesized the chiral phosphine catalyst CP6 bearing multiple phenol groups, and it was found that in the aza-MBH reaction of N-sulfonated aldimines with MVK using CP6, the corresponding adducts 35 can be obtained in >90% ee and good to high yields at −20 °C or room temperature (25 °C) in THF for most of the substrates using MVK, EVK, or acrolein as a Michael acceptor (Scheme 18).25 On the basis of the same hypothesis, Sasai,26a Ito,26b and Liu26c,26d independently reported multifunctional catalysts derived from BINOL for the asymmetric aza-MBH reaction, affording the corresponding adducts in good yields and high ee values.
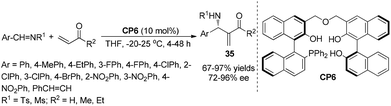 |
| Scheme 18 Asymmetric aza-MBH reaction catalyzed by CP6. | |
The catalyst CP1 was demonstrated to be an efficient catalyst in the aza-MBH reaction of N-tosylimines with MVK and phenyl acrylate. Recently, we also reported the asymmetric aza-MBH reaction of N-protected α-amidoalkyl phenyl sulfones 36 with MVK catalyzed by the catalyst CP1, affording the corresponding aza-MBH products 37 in good yields with high enantioselectivities (Scheme 19).27 The reaction was found to be general with respect to various α-amidoalkyl phenyl sulfones. Later, Sasai reported the first domino process based on the aza-MBH reaction catalyzed by bifunctional chiral phosphine (S)-CP1, affording 1,3-disubstituted isoindolines 40 in good yields with excellent diastereo- and enantioselectivities (up to 93% ee).28 The author proposed that this reaction might proceed via a tandem aza-MBH/intramolecular aza-Michael reaction sequence (Scheme 20).
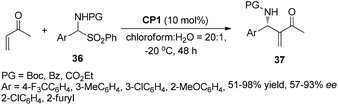 |
| Scheme 19 CP1-catalyzed asymmetric aza-MBH reactions. | |
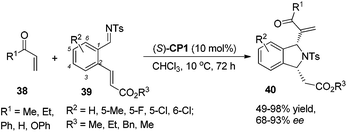 |
| Scheme 20 CP1-catalyzed asymmetric domino reaction. | |
Very recently, Lu's group designed and prepared a novel bifunctional phosphine-sulfonamide organic catalyst CP7 derived from L-threonine. CP7 was found to be an efficient catalyst for the asymmetric aza-MBH reaction of N-sulfonylimines with β-naphthyl acrylate. Notably, the ortho-substituted aromatic imines, which are well-known to be difficult substrates for the aza-MBH reaction, were found to be suitable substrates in this reaction, and the products 42 were obtained in nearly quantitative yields with up to 97% ee. These results represent by far the best enantioselectivities attainable for the ortho-substituted substrates in the aza-MBH reaction (Scheme 21).29a This catalyst can be also used for the catalytic asymmetric MBH reaction using aldehydes as electrophiles.29b Later, Sasai and co-workers have developed a novel spiro-type bifunctional organocatalyst CP8 having Lewis base and Brønsted acid moieties for the enantioselective aza-MBH reaction. This bifunctional spiro-phosphine catalyst CP8 was found to have high asymmetric induction to yield aza-MBH products (Scheme 22).30
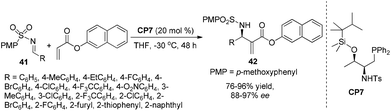 |
| Scheme 21 CP7-catalyzed asymmetric aza-MBH reaction. | |
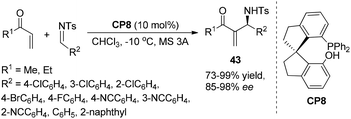 |
| Scheme 22 CP8-catalyzed asymmetric aza-MBH reaction. | |
2.3 Metal-catalyzed asymmetric aza-MBH reactions
In 2010, Matsunaga, Berkessel and Shibasaki found that the La(O-iPr)3/(S,S)-TMS-linked-BINOL 45 complex combined with a catalytic amount of DABCO could efficiently catalyze the aza-MBH reaction of N-diphenylphosphinoyl imines 44 with methyl acrylate.31 The La(O-iPr)3/(S,S)-TMS-linked-BINOL 45/DABCO system was applicable to a broad range of aryl, heteroaryl, alkenyl, and alkyl imines at ambient temperature, giving the desired products 46 in 67–99% yields and 81–98% ee (Scheme 23). Kinetic studies pointed out the importance of both the nucleophilicity of La-enolate and the Brønsted basicity of a La-catalyst for promoting the reaction.
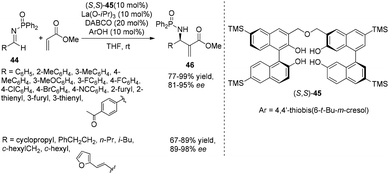 |
| Scheme 23 Catalytic asymmetric aza-MBH reaction of various N-Dpp imines with methyl acrylate. | |
On the basis of the same hypothesis, Shibata and coworkers have developed the first highly enantioselective aza-MBH reaction of acrylonitrile with sulfonated imines using chiral pincer type Pd complexes of 1,3-bis(imidazolin-2-yl)benzene bearing sterically bulky substituents with a catalytic amount of DABCO. A range of imines can be tolerated in this aza-MBH reaction to give the desired products in good yields with good ee values (Scheme 24).32
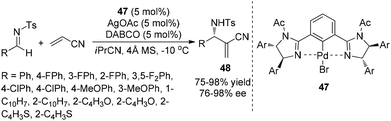 |
| Scheme 24 Enantioselective aza-MBH reaction catalyzed by palladium(II) pincer complexes. | |
2.4 Appendix
Although chiral organocatalyzed or organometallic complex-catalyzed aza-MBH reactions have been extensively investigated in recent decades, another methodology for the enantioselective aza-MBH reaction has been also emerging as an important alternative.33 In 2006, Leitner and co-workers reported the first example of an asymmetric aza-MBH reaction in which a chiral reaction medium could induce a high level of enantioselectivity. Using a specifically designed ionic liquid with a chiral anion as the only source of chirality, the desired aza-MBH reaction products were obtained in up to 84% ee (Scheme 25).34
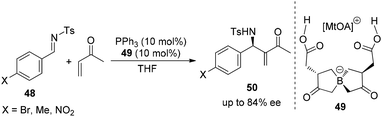 |
| Scheme 25 Highly enantioselective aza-MBH reaction in a chiral reaction medium. | |
3. Asymmetric aza-MBH reactions of ketimines
In 2010, our group and other groups independently reported the MBH reaction of N-protected isatins with electron-deficient alkenes to construct 3-substituted 3-hydroxyoxindoles in good yields and excellent enantioselectivities.35 Based on this work, we speculated that the chiral 3-aminooxindoles could be achieved via a similar strategy. Indeed, the asymmetric aza-MBH reaction of isatin-derived N-Boc ketimines 51 with MVK catalyzed by chiral amine and phosphine has been developed for the first time, providing a highly efficient and enantioselective synthesis of 3-amino-2-oxindoles bearing a quaternary stereogenic center (Scheme 26).36
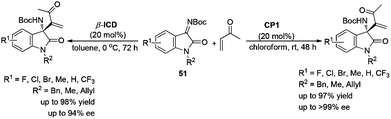 |
| Scheme 26 Chiral amine or phosphine-catalyzed asymmetric aza-MBH reaction. | |
At almost the same time, Chen's group developed a highly enantioselective aza-MBH reaction with N-Ts imines of β,γ-unsaturated α-ketoesters and acrolein for the first time, which relies on the employment of a combined catalytic system of β-ICD and bifunctional BINOL or tertiary amine-thiourea 56. A range of products 54 and 55 bearing a quaternary chiral center and densely functional groups have been efficiently produced in moderate to excellent enantioselectivity (up to 92% ee) (Scheme 27).37
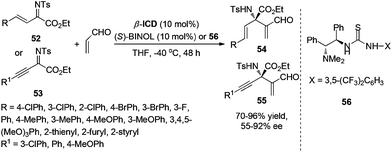 |
| Scheme 27 β-ICD-catalyzed aza-MBH reaction of ketimines with acrolein. | |
Subsequently, Sasai and co-workers developed the first highly enantioselective P-chirogenic organocatalyzed aza-MBH reaction to produce the desired products 58 with tetrasubstituted carbon stereogenic centers in moderate to excellent enantioselectivities (Scheme 28).38
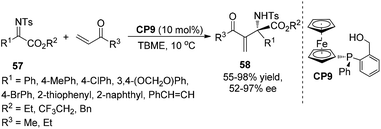 |
| Scheme 28 P-chirogenic organocatalyzed aza-MBH reaction of ketimines with MVK or EVK. | |
For the purpose of examination of the potential utility of the aza-MBH reaction, the reaction was carried out on a 1.0 g scale. As shown in Scheme 29, as for the substrate N-sulfonated imine 59, the reaction proceeded smoothly, affording the desired product 60 in a similar yield (83%) with the same enantiomeric excess (94% ee) as those reported before.13 However, when the aza-MBH reaction of ketimine 61 with MVK was carried out on a 1.0 g scale, the enantiomeric excess of the desired product 62 decreased remarkably from 97% to 84%.35 Adding 4 Å MS into the reaction system, the ee value of 61 declined from 97% to 91%, suggesting that the water or ambient moisture in the reaction system might affect the reaction outcomes. We speculated that on a large reaction scale, 4 Å MS could not completely get rid of the trace of water and ambient moisture in this particular reaction system and the water and ambient moisture might impair the ee value of the reaction product through the intramolecular hydrogen bonding.
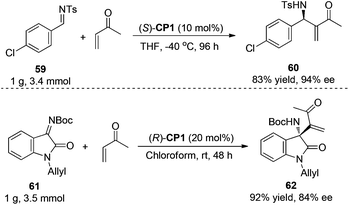 |
| Scheme 29 Enlarging the reaction scale of the asymmetric aza-MBH reaction. | |
Conclusion
In summary, asymmetric aza-MBH reactions have already become a powerful tool in organic chemistry, and have been studied intensively. In the past few decades, it has been demonstrated that great progress has been made in the asymmetric aza-MBH reactions of imines with α,β-unsaturated carbonyl compounds and a variety of chiral phosphine or amine organocatalysts has been found to be effective for this reaction. Although many important factors governing the reactions were identified, the present understanding of the basic factors and the control of reactivity and selectivity remains incomplete. There is no single catalyst which is suitable for all substrates so far; thus the development of effective catalysts and catalyst diversity for asymmetric aza-MBH reactions that are applicable to most of the common activated alkenes and electrophiles continues to be a challenging issue in this respect.
Acknowledgements
We thank the Shanghai Municipal Committee of Science and Technology (11JC1402600), the National Basic Research Program of China (973)-2010CB833302, the Fundamental Research Funds for the Central Universities, and the National Natural Science Foundation of China for financial support (20472096, 21372241, 21361140350, 20672127, 21102166, 21121062, 21302203 and 20732008).
Notes and references
-
(a)
M. B. Smith and J. March, March's Advanced Organic Chemistry: Reactions, Mechanisms, and Structure, Wiley, New York, 6th edn, 2007 Search PubMed;
(b)
F. A. Carey and R. J. Sundberg, Advanced Organic Chemistry, Springer, New York, 5th edn, 2007, Parts A and B Search PubMed.
-
(a) K. Morita, Z. Suzuki and H. Hirose, Bull. Chem. Soc. Jpn., 1968, 41, 2815 CrossRef CAS;
(b)
K. Morita, Japan Patent, 6803364, 1968 Search PubMed.
-
A. B. Baylis and M. E. D. Hillman, German Patent, 2155113, 1972 Search PubMed.
-
(a) S. E. Drewes and G. H. P. Roos, Tetrahedron, 1988, 44, 4653 CrossRef CAS;
(b) D. Basavaiah, P. D. Rao and R. S. Hyma, Tetrahedron, 1996, 52, 8001 CrossRef CAS;
(c) D. Basavaiah, T. Satyanarayana and A. J. Rao, Chem. Rev., 2003, 103, 811 CrossRef CAS PubMed;
(d) V. Declerck, J. Martinez and F. Lamaty, Chem. Rev., 2009, 109, 1 CrossRef CAS PubMed;
(e) D. Basavaiah, B. S. Reddy and S. S. Badsara, Chem. Rev., 2010, 110, 5447 CrossRef CAS PubMed;
(f) V. Singh and S. Batra, Tetrahedron, 2008, 64, 4511 CrossRef CAS;
(g)
E. Ciganek, in Organic Reactions, ed. L. A. Paquette, John Wiley & Sons, Inc., 1997, vol. 51, p. 201 Search PubMed;
(h) Y.-L. Shi and M. Shi, Eur. J. Org. Chem., 2007, 2905 CrossRef CAS;
(i) G. Masson, C. Housseman and J. Zhu, Angew. Chem., Int. Ed., 2007, 46, 4614 CrossRef CAS PubMed;
(j) Y. Wei and M. Shi, Acc. Chem. Res., 2010, 43, 1005 CrossRef CAS PubMed;
(k) Y. Lu, S.-X. Wang, X. Han, F. Zhong and Y. Wang, Synlett, 2011, 2766 CrossRef;
(l) J. Mansilla and J. M. Saa, Molecules, 2010, 15, 709 CrossRef CAS PubMed;
(m) V. Carrasco-Sanchez, M. J. Simirgiotis and L. S. Santos, Molecules, 2009, 14, 3989 CrossRef CAS PubMed;
(n) D. Basavaiah and G. Veeraraghavaiah, Chem. Soc. Rev., 2012, 41, 68 RSC;
(o) D. Basavaiah, K. V. Venkateswara Rao and R. J. Reddy, Chem. Soc. Rev., 2007, 36, 1581 RSC;
(p) Y. Wei and M. Shi, Chem. Rev., 2013, 113, 6659 CrossRef CAS PubMed.
-
(a) Y. Iwabuchi, M. Nakatani, N. Yokoyama and S. Hatakeyama, J. Am. Chem. Soc., 1999, 121, 10219 CrossRef CAS;
(b) M. Shi and Y.-M. Xu, Angew. Chem., Int. Ed., 2002, 41, 4507 CrossRef CAS.
-
(a) A. Nakano, S. Kawahara, S. Akamatsu, K. Morokuma, M. Nakatani, Y. Iwabuchi, K. Takahashi, J. Ishihara and S. Hatakeyama, Tetrahedron, 2006, 62, 381 CrossRef CAS;
(b) S. Kawahara, A. Nakano, T. Esumi, Y. Iwabuchi and S. Hatakeyama, Org. Lett., 2003, 5, 3103 CrossRef CAS PubMed.
- D. Balan and H. Adolfsson, Tetrahedron Lett., 2003, 44, 2521 CrossRef CAS.
- M. Shi, Y.-M. Xu and Y.-L. Shi, Chem. – Eur. J., 2005, 11, 1794 CrossRef CAS PubMed.
- K. Matsui, S. Takizawa and H. Sasai, J. Am. Chem. Soc., 2005, 127, 3680 CrossRef CAS PubMed.
- N. Abermil, G. Masson and J. Zhu, J.
Am. Chem. Soc., 2008, 130, 12596 CrossRef CAS PubMed.
- N. Abermil, G. Masson and J. Zhu, Org. Lett., 2009, 11, 4648 CrossRef CAS PubMed.
- N. Abermil, G. Masson and J. Zhu, Adv. Synth. Catal., 2010, 352, 656 CrossRef CAS.
- X.-Y. Guan, Y. Wei and M. Shi, Eur. J. Org. Chem., 2010, 4098 CrossRef CAS.
-
(a) Y. Sohtome, A. Tanatami, Y. Hashimoto and K. Nagasawa, Tetrahedron Lett., 2004, 45, 5589 CrossRef CAS;
(b) I. T. Raheem and E. N. Jacobsen, Adv. Synth. Catal., 2005, 347, 1701 CrossRef CAS.
- M. Pouliquen, J. Blanchet, M. D. Paolis, B. R. Devi, J. Rouden, M.-C. Lasne and J. Maddaluno, Tetrahedron: Asymmetry, 2010, 1511 CrossRef CAS.
- M. Shi, L.-H. Chen and C.-Q. Li, J. Am. Chem. Soc., 2005, 127, 3790 CrossRef CAS PubMed.
- M. Shi, G.-N. Ma and Y. Gao, J. Org. Chem., 2007, 72, 9779 CrossRef CAS PubMed.
- M. Shi and G.-L. Zhao, Adv. Synth. Catal., 2004, 346, 1205 CrossRef CAS.
- For (thio)urea derivative catalyzed reactions, see:
(a) M. S. Taylor and E. N. Jacobsen, Angew. Chem., Int. Ed., 2006, 45, 1520 CrossRef CAS PubMed;
(b) S. J. Connon, Chem. – Eur. J., 2006, 12, 5418 CrossRef PubMed;
(c) A. Berkessel, K. Roland and J. M. Neudörfl, Org. Lett., 2006, 8, 4195 CrossRef CAS PubMed.
- Y.-L. Shi and M. Shi, Adv. Synth. Catal., 2007, 349, 2129 CrossRef CAS.
- M. J. Qi, T. Ai, M. Shi and G. Li, Tetrahedron, 2008, 64, 1181 CrossRef CAS.
- X.-Y. Guan, Y.-Q. Jiang and M. Shi, Eur. J. Org. Chem., 2008, 2150 CrossRef CAS.
- J.-W. Han and T. Hayashi, Chem. Lett., 2001, 976 CrossRef CAS.
-
(a) M. Shi and L.-H. Chen, Pure Appl. Chem., 2005, 77, 2105 CrossRef CAS;
(b) M. Shi, L.-H. Chen and W.-D. Teng, Adv. Synth. Catal., 2005, 347, 1781 CrossRef CAS.
- Y.-H. Liu, L.-H. Chen and M. Shi, Adv. Synth. Catal., 2006, 348, 973 CrossRef CAS.
-
(a) K. Matsui, S. Takizawa and H. Sasai, Synlett, 2006, 761 CAS;
(b) K. Ito, K. Nishida and T. Gotauda, Tetrahedron Lett., 2007, 48, 6147 CrossRef CAS;
(c) J.-M. Garnier, C. Anstiss and F. Liu, Adv. Synth. Catal., 2009, 351, 331 CrossRef CAS;
(d) J.-M. Garnier and F. Liu, Org. Biomol. Chem., 2009, 7, 1272 RSC.
- X.-Y. Guan, Y. Wei and M. Shi, Eur. J. Org. Chem., 2010, 4098 CrossRef CAS.
- S. Takizawa, N. Inoue, S. Hirata and H. Sasai, Angew. Chem., Int. Ed., 2010, 49, 9725 CrossRef CAS PubMed.
-
(a) F. Zhong, Y. Wang, X. Han, K.-W. Huang and Y. Lu, Org. Lett., 2011, 13, 1310 CrossRef CAS PubMed;
(b) X. Han, Y. Wang, F. Zhong and Y. Lu, Org. Biomol. Chem., 2011, 9, 6734 RSC.
- S. Takizawa, K. Kiriyama, K. Ieki and H. Sasai, Chem. Commun., 2011, 47, 9227 RSC.
- T. Yukawa, B. Seelig, Y. Xu, H. Morimoto, S. Matsunaga, A. Berkessel and M. Shibasaki, J. Am. Chem. Soc., 2010, 132, 11988 CrossRef CAS PubMed.
- K. Hyodo, S. Nakamura and N. Shibata, Angew. Chem., Int. Ed., 2012, 51, 10337 CrossRef CAS PubMed.
- For the other types of asymmetric MBH reactions, see
(a) M. Shi, Y.-H. Liu and L.-H. Chen, Chirality, 2007, 19, 124 CrossRef CAS PubMed;
(b) M. Shi, M.-J. Qi and X.-G. Liu, Chem. Commun., 2008, 6025 RSC;
(c) Y.-H. Liu and M. Shi, Adv. Synth. Catal., 2008, 350, 122 CrossRef CAS;
(d) X. Wang, Y.-F. Chen, L.-F. Niu and P.-F. Xu, Org. Lett., 2009, 11, 3310 CrossRef CAS PubMed;
(e) C. Anstiss, J.-M. Garnier and F. Liu, Org. Biomol. Chem., 2010, 8, 4400 RSC;
(f) C. Anstiss and F. Liu, Tetrahedron, 2010, 66, 5486 CrossRef CAS;
(g) S. Takizawa, N. Inoue and H. Sasai, Tetrahedron Lett., 2011, 52, 377 CrossRef CAS;
(h) N. Lu, L. Meng, D. Chen and G. Zhang, RSC Adv., 2011, 1, 1113 RSC;
(i) Y.-L. Yang, Y. Wei and M. Shi, Org. Biomol. Chem., 2012, 10, 7429 RSC;
(j) S. Hirata, K. Tanaka, K. Matsui, F. A. Arteaga, Y. Yoshida, S. Takizawa and H. Sasai, Tetrahedron: Asymmetry, 2013, 24, 1189 CrossRef CAS;
(k) R. Lee, F. Zhong, B. Zheng, Y. Meng, Y. Lu and K.-W. Huang, Org. Biomol. Chem., 2013, 11, 4818 RSC;
(l) N. Lu, H. Wang and Y. Wang, Bull. Korean Chem. Soc., 2013, 34, 3591 CrossRef CAS;
(m) S. Kitagaki, Y. Ohta, R. Takahashi, M. Komizu and C. Mukai, Tetrahedron Lett., 2013, 54, 384 CrossRef CAS;
(n) P. Stepnicka, K. Skoch and I. Cisarova, Organometaliics, 2013, 32, 623 CrossRef CAS.
- R. Gausepohl, P. Buskens, J. Kleinen, A. Bruckmann, C. W. Lehmann, J. Klankermayer and W. Leitner, Angew. Chem., Int. Ed., 2006, 45, 3689 CrossRef CAS PubMed.
-
(a) X.-Y. Guan, Y. Wei and M. Shi, Chem. – Eur. J., 2010, 16, 13617 CrossRef CAS PubMed;
(b) Y.-L. Liu, B.-L. Wang, L. Chen, Y.-X. Zhang, C. Wang and J. Zhou, J. Am. Chem. Soc., 2010, 132, 15176 CrossRef CAS PubMed;
(c) F.-R. Zhong, G.-Y. Chen and Y.-X. Lu, Org. Lett., 2011, 13, 82 CrossRef CAS PubMed.
- F.-L. Hu, Y. Wei, M. Shi, S. Pindi and G. Li, Org. Biomol. Chem., 2013, 11, 1921 CAS.
- Y. Yao, J.-L. Li, Q.-Q. Zhou, L. Dong and Y.-C. Chen, Chem. – Eur. J., 2013, 19, 9447 CrossRef CAS PubMed.
- S. Takizawa, E. Rémond, F. A. Arteaga, Y. Yoshida, V. Sridharan, J. Bayardon, S. Jugé and H. Sasai, Chem. Commun., 2013, 49, 8392 RSC.
Footnote |
† Electronic supplementary information (ESI) available: Experimental procedures. See DOI: 10.1039/c4qo00026a |
|
This journal is © the Partner Organisations 2014 |