DOI:
10.1039/C4QO00012A
(Research Article)
Org. Chem. Front., 2014,
1, 373-381
Cp2TiCl-catalyzed highly stereoselective intramolecular epoxide allylation using allyl carbonates†
Received
14th January 2014
, Accepted 17th February 2014
First published on 18th March 2014
Abstract
A useful method for the diastereoselective synthesis of vinyl substituted carbo- and heterocycles is described. Highly functionalized structures difficult to achieve by other methodologies are obtained in a single step by this procedure.
Epoxides are highly versatile functional groups in organic synthesis owing to the fact that their manipulation yields many attractive final products. Thus, for example, diverse carbon nucleophiles, such as Grignard and organolithium reagents or organocuprates, have been used in ring-opening reactions to install a new C–C bond.1,2 The intramolecular version of this reaction would allow the preparation of different carbo- and heterocycles with different sizes and functionalities. Nevertheless, the synthesis of suitable polyfunctionalized starting materials is not simple taking into account the chemical incompatibilities between the required reactive partners. In this context, neutral pronucleophiles like olefins or allylsilanes (I, LG = SiR3, Scheme 1) are more convenient since they allow better control of the reaction and functional group compatibility.3 A valuable advantage of the reactions of epoxides with allylsilanes, compared with alkenes, is their ability to stabilize β-carbocations (III, Scheme 1), thereby controlling which carbon of the alkene is the nucleophilic carbon.4 Moreover the allylsilane can control the direction of the final elimination acting as a good leaving group that stabilizes the generated positive charge (IV, Scheme 1). On the other hand, its main drawback is related to the electrophilic character of the reaction, which implies the use of Lewis acids, such as TiCl4, and strict control over the temperature. Another disadvantage of allylsilanes relative to simple alkenes is that extra synthetic steps are necessary because they are generally prepared from oxygenated-allyl groups. Therefore, the direct employment of allylic oxygenated functionalities in epoxide ring-opening reactions retaining the favourable characteristics of allyl silane analogues using very mild reaction conditions would represent an important advance in organic synthesis.
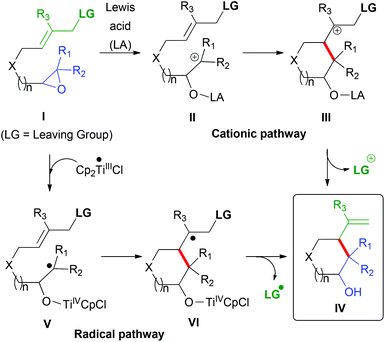 |
| Scheme 1 Working hypothesis. | |
The limitation of this approach is that the corresponding β-carbocations (Scheme 1, III, LG = OCOR) would not be stabilized and the control of the direction of elimination would remain a challenge due to the lack of a carbocation stabilizing group and also a good leaving group. As a result, a cationic pathway can be discarded in this case, and an alternative reaction pathway based on carbon-centered radicals was considered. Cp2TiCl5-mediated homolytic epoxide opening is a well-known reaction,6–19 which has allowed many remarkable transformations, including a highly successful bioinspired approach to different natural products.20–26 Epoxyallylcarboxylates I (LG = OCOR, Scheme 1) are expected to react with Cp2TiCl, yielding radical intermediate type-V. After the homolytic opening of the epoxide, the β-titanoxy radical generated V would undergo further radical cyclization generating a carbon-centered radical VI. At this point, we expected that an oxygenated function in the β-position would act as a good leaving group, thus directing the final elimination towards IV assisted by Cp2TiCl as the Lewis acid.27,28 In fact, we had previously observed the Cp2TiCl-mediated radical fragmentation of β-acetoxy alkyl radicals toward the corresponding alkenes.21,29
In this alternative radical pathway, Cp2TiCl would play a crucial dual role in the intramolecular epoxide allylation with oxygenated-allyl groups: (i) starting the reaction by homolytic opening of the oxirane ring and (ii) controlling the final product obtained by radical fragmentation.
Here we wish to communicate that epoxides can formally be allylated intramolecularly in a highly diastereoselective manner under smooth reaction conditions using easily prepared and handled allylic carbonates as allylation reagents. This approach also allows the preparation of different carbo- and heterocycles with different functionalities.
Due to the known oxophilic character of Ti(III), our initial studies began testing different allyl pronucleophiles 1a–d and 3, including different oxygenated functional groups such as carbonate, acetate, benzoate, methoxyl or hydroxyl groups. Moreover, an epoxyallylsilane 6 was also tested in order to compare the observed results with oxygenated functions. Remarkably, the new developments in titanocene(III)-regenerating agents now allow the use of substoichiometric amounts of Cp2TiCl2 as a precatalyst. In this context, the combination of 2,4,6-collidine and trimethylsilyl chloride developed in our lab30 has been extensively used, and it was the choice in this case.
Treatment of compounds 1a–d with Cp2TiCl led to the expected cyclic compound 2 with variable yields from 50 to 85% (Scheme 2). It is noteworthy that compound 2 was obtained as a single diastereomer in all cases. NOE-diff. experiments (see the Experimental section) showed a cis relationship between the hydroxyl group at C-3 and the vinyl group at C-5.
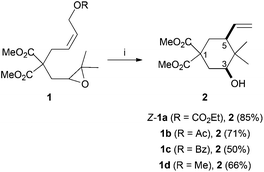 |
| Scheme 2 Ti(III)-mediated cyclization of model compounds 1a–d. Reaction conditions: (i) 1a–d (1.0 mmol) Cp2TiCl2 (0.2 mmol), Mn (8.0 mmol), Me3SiCl (4.0 mmol), 2,4,6-collidine (6.0 mmol), THF, RT, 16 h. Isolated yields after column chromatography. | |
When epoxyallylic alcohol 3 was treated with Cp2TiCl, cyclic compounds 4 and 5 were isolated in a 2/1 ratio (Scheme 3). In this case, the lack of a better leaving group resulted in a different final process. After homolytic oxirane-opening and subsequent cyclization, Ti(III)-mediated hydrogen abstraction in the radical intermediate yields aldehyde 4 (Scheme 3, process a).31 Besides, the radical intermediate can abstract a hydrogen-atom from the solvent (THF) leading to reduced product 5 (Scheme 3, process b).32
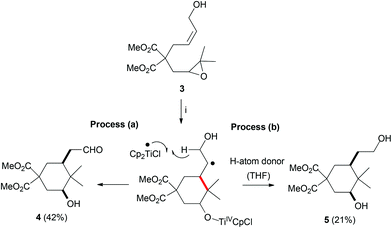 |
| Scheme 3 Ti(III)-mediated cyclization of compound 3. Reaction conditions: (i) 3 (1.0 mmol) Cp2TiCl2 (0.2 mmol), Mn (8.0 mmol), Me3SiCl (4.0 mmol), 2,4,6-collidine (6.0 mmol), THF, RT, 16 h. Isolated yields after column chromatography. | |
Silyl derivative 6 was assayed under the same reaction conditions, leading to a mixture of trimethylsilyl containing compounds 7 and 8 in a 1/0.6 ratio (Scheme 4).23 These two compounds were obtained by similar hydrogen-atom abstractions mentioned above. Ethyl carbonate derivative 1a (85% yield, Scheme 2) resulted in the best yield and therefore ethyl carbonate was the leaving group of choice for the following reactions.
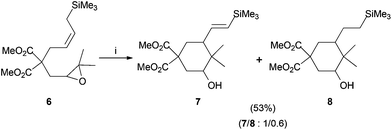 |
| Scheme 4 Ti(III)-mediated cyclization of compound 6. Reaction conditions: (i) 6 (1.0 mmol) Cp2TiCl2 (0.2 mmol), Mn (8.0 mmol), Me3SiCl (4.0 mmol), 2,4,6-collidine (6.0 mmol), THF, RT, 16 h. Isolated yield after column chromatography. Compounds 7 and 8 were not separated. | |
With the optimized conditions in hand, we explored substrates with different linkers, functionalities and substitution patterns. The results are summarized in Table 1.
Table 1 Substrate scope of [Ti]-catalyzed intramolecular epoxide allylations
Entry |
Substrate |
Product |
Yielda (%) |
Isolated yield after column chromatography.
7/3 mixture of cis/trans diastereoisomers.
Compounds 14 and 15 were obtained in a 1/1 ratio. Both compounds were obtained as diastereomeric mixture in 2/1 dr.
Additional 12% of cyclic compound S43 in which the carbonate group is eliminated was also obtained (see ESI for further details).
1/1 mixture of cis/trans diastereoisomers.
Additional 15% of cyclic compound S44 in which the carbonate moiety is eliminated was also obtained (see ESI for further details).
|
1 |
|
2
|
65% |
2 |
|
|
53% |
3 |
|
|
60%b |
4 |
|
|
55%c |
5 |
|
|
50%d |
6 |
|
|
54% |
7 |
|
|
58% |
8 |
|
|
76% |
9 |
|
|
74% |
10 |
|
|
71%e |
11 |
|
|
62%f |
12 |
|
|
73% |
The reaction successfully gave different five- and six-membered carbo- and heterocycles with excellent diastereoselectivities in almost all the tested substrates. Titanium-induced cyclization of compound E-1a (Table 1, entry 1) led to compound 2 as Z-1a (Scheme 2), revealing the stereoconvergent nature of the process. Additionally, the reaction proved to be compatible with different functional groups, including esters (Table 1, entries 1–7 and 11–12), sulfones (Table 1, entry 10), sulphonamides (Table 1, entries 8 and 9) or free hydroxyl groups (Table 1, entry 11), and permitted different substitution patterns in the oxirane ring (Table 1, entries 1–4) as well as in the involved alkene (Table 1, entries 5 and 11).
The regiochemistry of the radical epoxide opening mainly depends upon the substitution pattern33 and controls the size of the obtained final cycle (Table 1, entries 1 vs. 2 and entries 8 vs. 9). As shown in entry 4, treatment of compound 13 with Cp2TiCl led to the formation of a 1/1 mixture of five- and six-membered rings, as expected from a 1,2-disubstituted oxirane ring.8,9 However in compound 18 electronic effects control the homolytic epoxide opening, thus only affording the six-membered ring 19. Stereoconvergency was further demonstrated, as diastereomeric mixture 28 (entry 11) gave rise to a single cyclic diastereomer 29. It is also noteworthy that the stereoselectivity of this cyclization allows the setting up of two stereocenters in six-membered and notably five-membered rings (entries 2 and 9). When the stereocenters are located in 1,3-relative positions a cis stereochemistry between the hydroxyl group at C-3 and the vinyl group at C-5 is observed as in the case of compounds 2, 17 and 23 (entries 1, 5 and 8). On the other hand, compounds presenting contiguous stereocenters showed a trans (entries 2, 7, 9 and 12) or cis relationship (entry 3) between the vinyl and hydroxymethyl groups depending on the substitution pattern of the intermediate radical. Interestingly, in more functionalized substrates even three stereocenters can be allocated stereoselectively (entries 6 and 11). In the case of 1,3-relative positions, cis stereochemistry between the hydroxyl group at C-3 and the vinyl group at C-5 is preserved. The additional stereocenter at C-4 presents a trans stereochemistry with respect to the other two stereocenters.
All these stereochemical findings can be rationalized invoking the Beckwith–Houk rules.34,35cis Substituted five-membered rings are expected for 5-exo-trig cyclizations (entry 3). Trisubstituted radicals proceed disposing the bulkier substituent (R2 in Scheme 5a) in a pseudoequatorial position (Scheme 5) thus yielding the observed cyclopentanes 10 and 25 (entries 2 and 9).
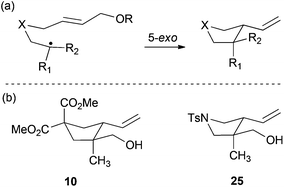 |
| Scheme 5 (a) Ring-closure of 5-hexenyl radicals. (b) Selected examples from Table 1. | |
Although cyclizations of 6-heptenyl radicals are less studied, a similar reasoning explains the experimental results. In the chair-like transition states all the bulkier substituents (R1 and R3 in Scheme 6) are disposed in the equatorial positions. Additional template effects cannot be ruled out in cyclization of compound 28 (entry 11).20–23
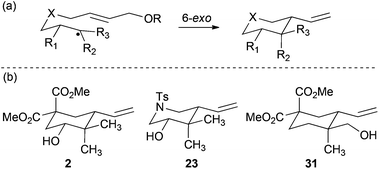 |
| Scheme 6 (a) Schematic ring-closure of 6-heptenyl radicals. (b) Selected examples from Table 1. | |
In the case of entries 4 and 10, the structures of compounds 13 and 26 do not follow these stereochemical trends and mixtures of diastereoisomers are obtained. The intrinsic reactivity of a 1,2-disubstituted epoxide in compound 13 (entry 4) precludes a clear analysis of its stereoselectivity. In compound 26 (entry 10), the transition state may be affected by bulky phenyl sulphonyl groups avoiding a clear chair-like transition state and leading to the formation of both isomers.
Conclusions
A useful method for the diastereoselective synthesis of vinyl substituted carbo- and heterocycles is presented. The protocol is based on the radical opening of an epoxide and subsequent intramolecular addition to an allyl carbonate. Formally, the reaction yields similar products as the allylation of epoxides by the appropriate nucleophile but with several significant advantages. Firstly, the polyfunctionalized substrates required are very easily obtained and handled. Secondly, the cyclization reaction occurs at room temperature and under very smooth conditions highly compatible with diverse functional groups. And lastly, the diastereoselectivity observed is quite remarkable giving rise in most of the cases to a single diastereomer even when three stereogenic centres are generated in the final product. Highly functionalized structures difficult to achieve by other methodologies are obtained in a single step by this procedure. Thus, this method is an interesting tool in the context of organic synthesis.
Experimental section
General remarks
Unless otherwise stated, all reagents and solvents (CH2Cl2, Et2O, MeCN, EtOAc, hexane, DMF, and MeOH) were purchased from commercial sources and used without further purification. Dry THF was freshly distilled over Na/benzophenone. Flash column chromatography was carried out using Silica Gel 60 (230–400 mesh, Scharlab, Spain) as the stationary phase. Analytical TLC was performed on aluminium sheets coated with silica gel with the fluorescent indicator UV254 (Alugram SIL G/UV254, Mackerey-Nagel, Germany) and observed under UV light (254 nm) and/or staining with Ce/Mo reagent or phosphomolybdic acid solution and subsequent heating. All 1H and 13C NMR spectra were recorded on Varian 300, 400 or 500 MHz spectrometers at a constant temperature of 298 K. Chemical shifts are reported in ppm and referenced to residual solvent. Coupling constants (J) are reported in hertz (Hz). Standard abbreviations indicating multiplicity were used as follows: m = multiplet, quint. = quintet, q = quartet, t = triplet, d = doublet, s = singlet, b = broad. Assignment of the 13C NMR multiplicities was accomplished by DEPT techniques.
Characterization data of substrates Z-1a, 1b–d, E-1a, 3, 6, 9, 11, 13, 16, 18, 20, 22, 24, 26, 28 and 30
Compound Z-1a.
1H-NMR (300 MHz, CDCl3) δ(ppm): 5.75–5.59 (m, 1H), 5.59–5.43 (m, 1H), 4.64 (d, J = 6.7 Hz, 2H), 4.15 (q, J = 7.0 Hz, 3H), 3.71 (s, 3H), 3.70 (s, 3H), 2.80 (d, J = 7.5 Hz, 2H), 2.70 (dd, J = 7.3, 4.2 Hz, 1H), 2.21 (dd, J = 14.8, 4.2 Hz, 1H), 1.95 (dd, J = 14.8, 7.3 Hz, 1H), 1.27 (t, J = 7.0 Hz, 3H), 1.24 (s, 3H), 1.20 (s, 3H). 13C-NMR (75 MHz, CDCl3) δ(ppm): 171.1 (C), 171.0 (C), 155.1 (C), 128.3 (CH), 127.2 (CH), 64.1 (CH2), 63.2 (CH2), 59.8 (C), 57.9 (C), 56.4 (C), 52.8 (CH3), 52.7 (CH3), 32.5 (CH2), 31.6 (CH2), 24.7 (CH3), 18.8 (CH3), 14.3 (CH3). HRMS (TOF MS ES+) m/z calcd for C17H26O8 [M + Na]+: 381.1519, found: 381.1525.
Compound 1b.
1H-NMR (300 MHz, CDCl3) δ(ppm): 5.70–5.55 (m, 1H), 5.55–5.40 (m, 1H), 4.57 (d, J = 6.6 Hz, 1H), 3.70 (s, 3H), 3.68 (s, 3H), 2.78 (d, J = 7.5 Hz, 2H), 2.68 (dd, J = 7.3, 4.3 Hz, 1H), 2.19 (dd, J = 14.9, 4.3 Hz, 1H), 1.99 (s, 3H), 2.00–1.89 (m, 1H), 1.23 (s, 3H), 1.19 (s, 3H). 13C-NMR (75 MHz, CDCl3) δ(ppm): 171.1 (C), 170.8 (C), 127.7 (CH), 60.1 (CH2), 59.8 (CH), 57.9 (C), 56.3 (C), 52.8 (CH3), 52.6 (CH3), 32.3 (CH2), 31.4 (CH2), 24.6 (CH3), 20.9 (CH3), 18.7 (CH3). HRMS (TOF MS ES+) m/z calcd for C16H24O7Na [M + Na]+: 351.1414, found: 351.1415.
Compound 1c.
1H-NMR (300 MHz, CDCl3) δ(ppm): 8.00 (d, J = 7.4 Hz, 2H), 7.52 (t, J = 7.4 Hz, 1H), 7.40 (t, J = 7.4 Hz, 2H), 5.87–5.71 (m, 1H), 5.64–5.50 (m, 1H), 4.86 (d, J = 6.7 Hz, 2H), 3.73 (s, 3H), 3.71 (s, 3H), 2.89 (d, J = 7.5 Hz, 2H), 2.74 (dd, J = 7.4, 4.1 Hz, 1H), 2.25 (dd, J = 14.8, 4.1 Hz, 1H), 2.08–1.94 (m, 1H), 1.24 (s, 3H), 1.21 (s, 3H). 13C-NMR (75 MHz, CDCl3) δ(ppm): 171.2 (C), 171.1 (C), 166.4 (C), 133.0 (CH), 130.2 (C), 129.6 (CH), 128.4 (CH), 128.0 (CH), 127.8 (CH), 60.6 (CH2), 59.9 (CH), 58.0 (C), 56.4 (C), 52.8 (CH3), 52.7 (CH3), 32.4 (CH2), 31.6 (CH2), 24.7 (CH3), 18.8 (CH3). HRMS (TOF MS ES+) m/z calcd for C21H26O7Na [M + Na]+: 413.1570, found: 413.1569.
Compound 1d.
1H-NMR (300 MHz, C6D6) δ(ppm): 5.85–5.68 (m, 1H), 5.60–5.43 (m, 1H), 3.86 (d, J = 6.3 Hz, 2H), 3.39 (s, 3H), 3.36 (s, 3H), 3.06 (s, 3H), 3.09–3.02 (m, 2H), 2.87 (dd, J = 8.0, 3.8 Hz, 1H), 2.45 (dd, J = 14.8, 3.8 Hz, 1H), 2.20 (dd, J = 14.8, 8.0 Hz, 1H), 1.05 (s, 3H), 1.03 (s, 3H). 13C-NMR (75 MHz, CDCl3) δ(ppm): 171.2 (C), 171.1 (C), 130.4 (CH), 125.9 (CH), 67.9 (CH2), 59.8 (CH), 58.0 (CH3), 57.9 (C), 56.3 (C), 52.7 (CH3), 52.6 (CH3), 32.2 (CH2), 31.5 (CH2), 24.6 (CH3), 18.7 (CH3). HRMS (TOF MS ES+) m/z calcd for C15H24O6Na [M + Na]+: 323.1465, found: 323.1474.
Compound 3.
1H-NMR (300 MHz, C6D6) δ(ppm): 5.84–5.68 (m, 1H), 5.51–5.34 (m, 1H), 4.21–4.06 (m, 1H), 4.06–3.91 (m, 1H), 3.36 (s, 3H), 3.34 (s, 3H), 3.12 (dd, J = 14.8, 8.5 Hz, 1H), 2.98 (dd, J = 14.8, 6.8 Hz, 1H), 2.80 (dd, J = 8.3, 3.2 Hz, 1H), 2.48 (dd, J = 14.9, 3.2 Hz, 1H), 2.12 (dd, J = 14.9, 8.3 Hz, 1H), 1.00 (s, 3H), 0.99 (s, 3H). 13C-NMR (75 MHz, C6D6) δ(ppm): 171.5 (C), 171.3 (C), 134.0 (CH), 125.0 (CH), 60.1 (CH), 58.1 (CH2), 57.7 (C), 57.1 (C), 52.4 (CH3), 52.3 (CH3), 32.8 (CH2), 31.6 (CH2), 24.5 (CH3), 18.6 (CH3). HRMS (TOF MS ES+) m/z calcd for C14H22O6Na [M + Na]+: 309.1308, found: 309.1307.
Compound 6.
1H-NMR (300 MHz, CDCl3) δ(ppm): 5.62–5.45 (m, 1H), 5.18–5.01 (m, 1H), 3.74 (s, 3H), 3.73 (s, 3H), 2.80–2.72 (m, 1H), 2.71 (d, J = 7.0 Hz, 2H), 2.20 (dd, J = 14.8, 4.6 Hz, 1H), 2.00 (dd, J = 14.8, 7.3 Hz, 1H), 1.42 (d, J = 8.0 Hz, 2H), 1.28 (s, 3H), 1.24 (s, 3H), −0.02 (s, 9H). 13C-NMR (75 MHz, CDCl3) δ(ppm): 171.6 (C), 132.0 (CH), 121.3 (CH), 60.1 (CH), 58.0 (C), 56.8 (C), 52.6 (CH3), 52.5 (CH3), 36.9 (CH2), 32.0 (CH2), 24.8 (CH3), 23.1 (CH3), 18.8 (CH3), −1.9 (CH3). HRMS (TOF MS ES+) m/z calcd for C17H31O5Si [M + H]+: 343.1941, found: 343.1951.
Compound E-1a.
1H-NMR (300 MHz, acetone) δ(ppm): 5.75–5.65 (m, 2H), 4.69 (d, J = 6.4 Hz, 2H, Z-isomer), 4.54 (d, J = 6.4 Hz, 2H, E-isomer), 4.19 (q, J = 7.1 Hz, 2H), 3.75 (s, 3H), 3.73 (s, 3H), 2.84 (d, J = 7.2 Hz, 2H, Z-isomer), 2.81–2.69 (m, 3H), 2.22 (dd, J = 14.9, 4.2 Hz, 1H), 1.99 (dd, J = 14.9, 7.6 Hz, 1H), 1.30 (t, J = 7.1 Hz, 3H), 1.28 (s, 3H), 1.25 (s, 3H). 13C-NMR (75 MHz, CDCl3) δ(ppm): 171.0 (C), 170.9 (C), 154.8 (C), 129.7 (CH), 128.4 (CH), 67.5 (CH2), 63.9 (CH2), 59.7 (CH), 57.8 (C), 56.5 (CH), 52.6 (CH3), 52.5 (CH3), 36.2 (CH2), 32.2 (CH2), 24.6 (CH3), 18.7 (CH3), 14.2 (CH3). HRMS (TOF MS ES+) m/z calcd for C17H26O8Na [M + Na]+: 381.1519, found: 381.1516.
Compound 9.
1H-NMR (300 MHz, C6D6) δ(ppm): 5.74–5.46 (m, 2H), 4.63 (d, J = 5.7 Hz, 2H), 3.91 (q, J = 7.1 Hz, 2H), 3.35 (s, 6H), 3.00–2.89 (m, 2H), 2.31 (d, J = 3.9 Hz, 2H), 2.26 (d, J = 5.1 Hz, 1H), 2.11 (d, J = 5.1 Hz, 1H), 1.10 (s, 3H), 0.93 (t, J = 7.1 Hz, 3H). 13C-NMR (75 MHz, C6D6) δ(ppm): 171.2 (C), 171.0 (C), 155.5 (C), 128.9 (CH), 127.4 (CH), 63.7 (CH2), 63.1 (CH2), 56.7 (C), 54.0 (CH2), 53.9 (C), 52.2 (CH3), 52.2 (CH3), 39.9 (CH2), 32.1 (CH2), 21.6 (CH3), 14.2 (CH3). HRMS (TOF MS ES+) m/z calcd for C16H24O8Na [M + Na]+: 367.1363, found: 367.1372.
Compound 11.
1H-NMR (300 MHz, C6D6) δ(ppm): 5.69–5.54 (m, 1H), 5.56–5.38 (m, 1H), 4.61 (d, J = 6.7 Hz, 2H), 3.90 (q, J = 7.1 Hz, 2H), 3.35 (s, 3H), 3.33 (s, 3H), 2.93 (d, J = 7.8 Hz, 2H), 2.87–2.76 (m, 1H), 2.33–2.20 (m, 2H), 1.98 (dd, J = 5.2, 2.4 Hz, 1H), 1.88 (dd, J = 14.7, 7.8 Hz, 1H), 0.90 (t, J = 7.1 Hz, 3H). 13C-NMR (75 MHz, C6D6) δ(ppm): 171.0 (C), 170.9 (C), 155.5 (C), 128.5 (CH), 127.8 (CH), 63.8 (CH2), 63.1 (CH2), 56.7 (CH2), 52.3 (CH3), 48.2 (CH), 46.0 (CH2), 36.8 (CH2), 31.9 (CH2), 14.2 (CH3). HRMS (TOF MS ES+) m/z calcd for C15H22O8Na [M + Na]+: 353.1206, found: 353.1197.
Compound 13.
5.69–5.56 (m, 1H), 5.56–5.41 (m, 1H), 4.61 (d, J = 6.9 Hz, 2H), 3.89 (q, J = 7.1 Hz, 2H), 3.36 (s, 3H), 3.34 (s, 3H), 2.94 (d, J = 7.8 Hz, 2H), 2.76–2.68 (m, 1H), 2.44–2.34 (m, 1H), 2.28 (dd, J = 14.6, 4.2 Hz, 1H), 2.02 (dd, J = 14.6, 7.4 Hz, 1H), 0.96 (d, J = 5.2 Hz, 3H), 0.90 (t, J = 7.1 Hz, 3H). 13C-NMR (75 MHz, C6D6) δ(ppm): 170.9 (C), 155.0 (C), 128.2 (CH), 127.2 (CH), 64.0 (CH2), 63.1 (CH2), 56.2 (C), 55.4 (CH), 54.3 (CH), 52.8 (CH3), 52.7 (CH3), 35.9 (CH2), 31.6 (CH2), 17.3 (CH3), 14.3 (CH3). HRMS (TOF MS ES+) m/z calcd for C16H24O8 [M + Na]+: 367.1363, found: 367.1377.
Compound 16.
1H-NMR (300 MHz, CDCl3) δ(ppm): 5.56 (t, J = 7.0 Hz, 1H), 4.40 (s, 2H), 3.93 (q, J = 7.1 Hz, 2H), 3.38 (s, 3H), 3.36 (s, 3H), 3.00 (t, J = 7.0 Hz, 2H), 2.86 (dd, J = 7.9, 4.1 Hz, 1H), 2.43 (dd, J = 14.8, 4.1 Hz, 1H), 2.20 (dd, J = 14.8, 7.9 Hz, 1H), 1.51 (s, 3H), 1.05 (s, 3H), 1.04 (s, 3H), 0.93 (t, J = 7.1 Hz, 3H). 13C-NMR (75 MHz, CDCl3) δ(ppm): 171.4 (C), 171.2 (C), 155.4 (C), 134.4 (C), 122.9 (CH), 72.6 (CH2), 63.7 (CH2), 59.7 (CH), 57.3 (C), 56.8 (C), 52.3 (CH3), 52.2 (CH3), 32.9 (CH2), 32.1 (CH2), 24.6 (CH3), 18.7 (CH3), 14.2 (CH3), 13.9 (CH3). HRMS (TOF MS ES+) m/z calcd for C18H28O8Na [M + Na]+: 395.1676, found: 395.1667.
Compound 18.
1H-NMR (300 MHz, CDCl3) δ(ppm): 7.36–7.25 (m, 3H), 7.26–7.16 (m, 2H), 5.78–5.61 (m, 2H), 4.52 (d, J = 4.5 Hz, 2H), 4.16 (q, J = 7.1 Hz, 2H), 3.73 (s, 3H), 3.69 (s, 3H), 3.56 (d, J = 1.8 Hz, 1H), 2.96 (ddd, J = 6.7, 5.0, 1.8 Hz, 1H), 2.77 (d, J = 5.7 Hz, 2H), 2.27 (dd, J = 14.7, 5.0 Hz, 1H), 2.14 (dd, J = 14.7, 6.7 Hz, 1H), 1.27 (t, J = 7.1 Hz, 3H). 13C-NMR (75 MHz, CDCl3) δ(ppm): 170.9 (C), 154.9 (C), 136.9 (C), 129.6 (CH), 128.6 (CH), 128.5 (CH), 128.3 (CH), 125.6 (CH), 67.5 (CH2), 64.0 (CH2), 58.8 (CH), 58.5 (CH), 56.4 (C), 52.8 (CH3), 36.7 (CH2), 36.2 (CH2), 14.3 (CH3). HRMS (TOF MS ES+) m/z calcd for C21H26O8Na [M + Na]+: 429.1519, found: 429.1529.
Compound 20.
1H-NMR (300 MHz, CDCl3) δ(ppm): 5.76–5.59 (m, 2H), 4.54 (d, J = 4.5 Hz, 2H), 4.19 (q, J = 7.1 Hz, 2H), 3.72 (s, 6H), 2.95–2.85 (m, 1H), 2.79–2.70 (m, 1H), 2.65 (d, J = 5.5 Hz, 2H), 2.51–2.42 (m, 1H), 2.11–1.91 (m, 2H), 1.54–1.38 (m, 2H), 1.30 (t, J = 7.1 Hz, 3H). 13C-NMR (75 MHz, CDCl3) δ(ppm): 171.1 (C), 154.9 (C), 129.7 (CH), 128.1 (CH), 67.5 (CH2), 63.9 (CH2), 57.1 (C), 52.5 (CH3), 51.7 (CH), 46.9 (CH2), 35.9 (CH2), 28.9 (CH2), 27.3 (CH2), 14.2 (CH3). HRMS (TOF MS ES+) m/z calcd for C16H24O8Na [M + Na]+: 367.1363, found: 367.1362.
Compound 22.
1H-NMR (300 MHz, CDCl3) δ(ppm): 7.71 (d, J = 8.3 Hz, 2H), 7.31 (d, J = 8.3 Hz, 2H), 5.79–5.64 (m, 1H), 5.64–5.51 (m, 1H), 4.68 (d, J = 6.7 Hz, 2H), 4.18 (q, J = 7.1 Hz, 2H), 4.09–3.90 (m, 2H), 3.65 (dd, J = 14.8, 3.7 Hz, 1H), 2.95 (dd, J = 14.8, 5.7 Hz, 1H), 2.87 (dd, J = 5.7, 3.7 Hz, 1H), 2.43 (s, 3H), 1.29 (t, J = 7.1 Hz, 3H), 1.28 (s, 3H), 1.24 (s, 3H). 13C-NMR (75 MHz, CDCl3) δ(ppm): 154.9 (C), 143.6 (C), 136.6 (C), 129.8 (CH), 129.6 (CH), 127.2 (CH), 127.1 (CH), 64.1 (CH2), 62.7 (CH2), 61.9 (CH), 57.8 (C), 47.1 (CH2), 45.3 (CH2), 24.4 (CH3), 21.5 (CH3), 18.8 (CH3), 14.2 (CH3). HRMS (TOF MS ES+) m/z calcd for C19H28NO6S [M + H]+: 398.1637, found: 398.1627.
Compound 24.
1H-NMR (300 MHz, C6D6) δ(ppm): 7.65 (d, J = 8.2 Hz, 2H), 6.78 (d, J = 8.2 Hz, 2H), 5.55–5.34 (m, 2H), 4.63 (d, J = 6.2 Hz, 2H), 3.91 (d, J = 6.1 Hz, 2H), 3.88 (q, J = 7.1 Hz, 2H), 3.35 (d, J = 14.5 Hz, 1H), 2.79 (d, J = 14.5 Hz, 1H), 2.25 (d, J = 4.8 Hz, 1H), 2.10 (d, J = 4.8 Hz, 1H), 1.90 (s, 3H), 1.20 (s, 3H), 0.90 (t, J = 7.1 Hz, 3H). 13C-NMR (75 MHz, acetone) δ(ppm): 155.7 (C), 144.4 (C), 138.2 (C), 130.7 (CH), 130.1 (CH), 128.1 (CH), 127.9 (CH), 64.4 (CH2), 63.5 (CH2), 56.2 (CH2), 53.6 (CH), 51.9 (CH2), 46.3 (CH2), 21.5 (CH3), 19.2 (CH3), 14.6 (CH3). HRMS (TOF MS ES+) m/z calcd for C18H26NO6S [M + H]+: 384.1481, found: 384.1476.
Compound 26.
1H-NMR (300 MHz, C6D6) δ(ppm): 8.22–8.11 (m, 4H), 7.09–6.93 (m, 6H), 6.08 (dt, J = 11.0, 6.5 Hz, 1H), 5.55 (dt, J = 11.0, 6.6 Hz, 1H), 4.43 (d, J = 6.8 Hz, 2H), 3.88 (q, J = 7.1 Hz, 2H), 3.49 (t, J = 4.9 Hz, 1H), 3.36 (d, J = 6.5 Hz, 2H), 2.81 (dd, J = 16.1, 4.9 Hz, 1H), 2.50 (dd, J = 16.1, 4.9 Hz, 1H), 1.16 (s, 3H), 1.02 (s, 3H), 0.91 (t, J = 7.1 Hz, 3H). 13C-NMR (75 MHz, C6D6) δ(ppm): 155.4 (C), 137.4 (C), 137.2 (C), 134.6 (CH), 134.5 (CH), 132.0 (CH), 131.9 (CH), 128.8 (CH), 128.7 (CH), 127.6 (CH), 126.8 (CH), 89.8 (C), 63.9 (CH2), 62.8 (CH2), 58.7 (CH), 58.5 (C), 30.6 (CH2), 28.9 (CH2), 24.5 (CH3), 18.8 (CH3), 14.2 (CH3). HRMS (TOF MS ES+) m/z calcd for C25H30O8S2Na [M + Na]+: 545.1274, found: 545.1260.
Compound 28.
1H-NMR (300 MHz, CDCl3) δ(ppm): 5.31 (t, J = 7.3 Hz, 1H), 4.42 (s, 2H), 4.13 (q, J = 7.1 Hz, 2H), 3.69 (s, 3H), 3.68 (s, 3H), 3.57 (s, 2H), 2.80 (dd, J = 6.9, 5.2 Hz, 1H), 2.71 (d, J = 7.3 Hz, 2H), 2.19 (dd, J = 14.9, 5.2 Hz, 1H), 2.03 (dd, J = 14.9, 6.9 Hz, 1H), 1.63 (s, 3H), 1.30 (s, 3H), 1.24 (t, J = 7.1 Hz, 3H). 13C-NMR (75 MHz, CDCl3) δ(ppm): 171.3 (C), 171.2 (C), 155.0 (C), 134.1 (C), 122.6 (CH), 72.7 (CH2), 64.0 (CH2), 63.5 (CH2), 60.6 (CH), 60.4 (C), 56.3 (C), 52.8 (CH3), 52.7 (CH3), 32.0 (CH2), 31.6 (CH2), 19.9 (CH3), 14.2 (CH3), 14.0 (CH3). HRMS (TOF MS ES+) m/z calcd for C18H28O9Na [M + Na]+: 411.1625, found: 411.1624.
Compound 30.
1H-NMR (300 MHz, CDCl3) δ(ppm): 5.72–5.59 (m, 1H), 5.53–5.40 (m, 1H), 4.61 (d, J = 6.8 Hz, 2H), 4.14 (q, J = 7.1 Hz, 2H), 3.67 (s, 6H), 2.65 (d, J = 7.6 Hz, 2H), 2.53 (dd, J = 11.9, 4.7 Hz, 2H), 1.92 (t, J = 8.3 Hz, 2H), 1.51–1.31 (m, 2H), 1.26 (t, J = 7.1 Hz, 3H), 1.25 (s, 3H). 13C-NMR (75 MHz, CDCl3) δ(ppm): 171.2 (C), 155.0 (C), 128.3 (CH), 127.0 (CH), 64.0 (CH2), 63.0 (CH2), 57.0 (C), 56.4 (C), 53.5 (CH2), 52.6 (CH3), 31.3 (CH2), 30.8 (CH2), 28.2 (CH2), 20.8 (CH3), 14.3 (CH3). HRMS (TOF MS ES+) m/z calcd for C17H27O8 [M + H]+: 359.1706, found: 359.1719.
General procedure for the intramolecular epoxide allylation
Rigorously deoxygenated dry THF (10 mL) was added to a previously deoxygenated mixture of Cp2TiCl2 (0.2 mmol) and Mn (8.0 mmol) under an Ar atmosphere, and the suspension was stirred at room temperature until it turned green (about 10 min). A solution of the previously synthesized polyfunctionalized substrate (1.0 mmol) in THF (2 mL), Me3SiCl (4.0 mmol) and 2,4,6-collidine (6.0 mmol) was then added. The reaction mixture was stirred at room temperature for 16 h and then diluted with EtOAc, washed with HCl (10%), dried over anhydrous Na2SO4 and the solvent removed. The residue was subjected to flash column chromatography (SiO2, EtOAc–hexane mixtures) to give the corresponding cyclic products.
Characterization data of cyclic products 2, 4, 5, 7, 8, 10, 12, 14, 15, 17, 19, 21, 23, 25, 27, 29, and 31
(See ESI† for numbering and copies of 1H-NMR and 13C-NMR spectra.)
Compound 2.
Colorless oil; 65–85% yield. 1H-NMR (500 MHz, CDCl3) δ(ppm): 5.69 (ddd, J = 17.2, 10.4, 8.1 Hz, 1H), 5.06 (dd, J = 10.4, 1.8 Hz, 1H), 5.03 (d, J = 17.2 Hz, 1H), 3.74 (s, 3H), 3.69 (s, 3H), 3.40 (dd, J = 12.1, 4.2 Hz, 1H), 2.37 (ddd, J = 12.1, 4.2, 2.4 Hz, 1H), 2.10 (dt, J = 13.6, 2.7 Hz, 1H), 1.90 (ddd, J = 12.7, 8.1, 2.7 Hz, 1H), 1.82 (t, J = 12.7 Hz, 1H), 1.80 (t, J = 13.2 Hz, 1H), 0.95 (s, 3H), 0.77 (s, 3H); NOE-diff. experiment: proton irradiated, (NOEs observed): H-7, (H2-8, H-5), H-3, (H-2b H-5). 13C-NMR (75 MHz, CDCl3) δ(ppm): 171.9 (C), 171.5 (C), 137.7 (CH), 116.6 (CH2), 74.4 (CH), 54.9 (C), 52.9 (CH3), 52.8 (CH3), 47.0 (CH), 38.0 (C), 34.7 (CH2), 31.9 (CH2), 25.6 (CH3), 12.3 (CH3). HRMS (TOF MS ES+) m/z calcd for C14H22O5Na [M + Na]+: 293.1359, found: 293.1351.
Compound 4.
Colorless oil; 40% yield. 1H-NMR (500 MHz, CDCl3) δ(ppm): 9.74 (s, 1H), 3.77 (s, 3H), 3.69 (s, 3H), 3.46 (dd, J = 11.9, 3.8 Hz, 1H), 2.57 (dd, J = 16.8, 2.4 Hz, 1H), 2.39 (ddd, J = 13.2, 4.0, 2.3 Hz, 1H), 2.17 (dd, J = 10.0, 2.9 Hz, 1H), 2.12 (dt, J = 13.2, 3.5 Hz, 1H), 1.97–1.86 (m, 1H), 1.80 (t, J = 12.6 Hz, 1H), 1.61 (t, J = 13.4 Hz, 1H), 0.99 (s, 3H), 0.77 (s, 3H); NOE-diff. experiment: proton irradiated (NOEs observed): H-7a (H-7b, H-5), H-3 (H-2a, H-5), H-2a (H-2b, H-3). 13C-NMR (125 MHz, CDCl3) δ(ppm): 201.8 (C), 171.5 (C), 171.2 (C), 73.9 (CH), 54.7 (C), 53.0 (CH2), 52.9 (CH2), 44.6 (CH2), 38.0 (C), 36.5 (CH), 34.7 (CH2), 32.5 (CH2), 25.1 (CH3), 12.4 (CH3). HRMS (TOF MS ES+) m/z calcd for C14H22O6Na [M + Na]+: 309.1308, found: 309.1308.
Compound 5.
Colorless oil; 23% yield. 1H-NMR (400 MHz, CDCl3) δ(ppm): 3.75 (s, 3H), 3.71 (s, 3H), 3.71–3.57 (m, 2H), 3.34 (dd, J = 11.8, 4.0 Hz, 1H), 2.39 (ddd, J = 13.2, 3.9, 2.3 Hz, 1H), 2.25 (dt, J = 13.8, 2.7 Hz, 1H), 1.86 (t, J = 12.0 Hz, 1H), 1.85–1.77 (m, 1H), 1.63–1.57 (bs, 2H), 1.51 (t, J = 13.0 Hz, 1H), 1.38–1.17 (m, 2H), 1.00 (s, 3H), 0.77 (s, 3H). 13C-NMR (100 MHz, CDCl3) δ(ppm): 171.9 (C), 171.5 (C), 74.6 (CH), 61.5 (CH2), 54.9 (C), 53.0 (CH2), 52.9 (CH2), 38.6 (CH), 38.3 (C), 34.7 (CH2), 32.2 (CH2), 31.7 (CH2), 24.9 (CH3), 12.2 (CH3). HRMS (TOF MS ES+) m/z calcd for C14H24O6Na [M + Na]+: 311.1465, found: 311.1468.
Compounds 7 and 8.
Compounds 7 and 8 were isolated as a mixture in a 7/8 ratio of 1.6/1. Colorless oil; 53% yield. (Compounds 7 (33% yield) and 8 (20% yield) were not separated.) 1H-NMR (400 MHz, CDCl3) δ(ppm): 5.89 (dd, J = 18.7, 7.0 Hz, 1H, 7), 5.67 (d, J = 18.7 Hz, 1H, 7), 3.75 (s, 3H, 7), 3.74 (s, 3H, 8), 3.71 (s, 3H), 3.40 (dd, J = 12.0, 4.1 Hz, 1H, 7), 3.34 (dd, J = 12.0, 4.0 Hz, 1H, 8), 2.37 (ddd, J = 12.6, 4.0, 2.2 Hz, 1H), 2.11 (dt, J = 12.8, 2.0 Hz, 1H), 1.96–1.74 (m, 3H), 1.60–1.50 (m, 1H, 8), 1.40 (t, J = 13.0 Hz, 1H, 8), 0.99 (s, 3H, 8), 0.95 (s, 3H, 7), 0.94–0.80 (m, 2H, 8) 0.77 (s, 3H, 7), 0.73 (s, 3H, 8), 0.72–0.61 (m, 1H, 8), 0.35–0.20 (m, 1H, 8), 0.04 (s, 9H, 7), 0.03 (s, 9H, 8). 13C-NMR (100 MHz, CDCl3) δ(ppm): 172.2 (C), 171.9 (C), 171.6 (C), 171.5 (C), 145.2 (CH, 7), 132.6 (CH, 7), 74.6 (CH), 74.4 (CH), 54.9 (C), 54.8 (C), 53.0 (CH3), 52.9 (CH3), 52.8 (CH3), 52.7 (CH3), 49.1 (CH), 45.7 (CH), 38.7 (C), 38.1 (C), 34.8 (CH2), 34.6 (CH2), 31.7 (CH2), 31.2 (CH2), 25.6 (CH3), 25.0 (CH3), 23.2 (CH2, 8), 15.1 (CH2, 8), 12.4 (CH3), 12.1 (CH3), −1.6 (CH3), −1.1 (CH3). HRMS (TOF MS ES+) m/z calcd for C17H30O5Si [M]+: 342.1863, found: 342.1867. HRMS for compound 8 was not found.
Compound 10.
Colorless oil; 53% yield. 1H-NMR (500 MHz, CDCl3) δ(ppm): 5.74–5.62 (m, 1H), 5.06 (d, J = 16.1 Hz, 1H), 5.05 (d, J = 11.3 Hz, 1H), 3.71 (s, 6H), 3.41 (d, J = 10.9 Hz, 1H), 3.36 (d, J = 10.9 Hz, 1H), 2.59–2.49 (m, 1H), 2.43 (d, J = 14.2 Hz, 1H), 2.42–2.37 (m, 1H), 2.28 (t, J = 12.8 Hz, 1H), 2.06 (d, J = 14.2 Hz, 1H), 0.82 (s, 3H); NOE-diff. experiment: proton irradiated, (NOEs observed): H3-8, (H-2b, H2-9, H-6), H-6, (H2-5, H-4, H3-8), H-4, (H-6, H2-7, H2-5, H2-9). 13C-NMR (125 MHz, CDCl3) δ(ppm): 173.5 (C), 172.9 (C), 137.3 (CH), 116.7 (CH2), 69.3 (CH2), 57.9 (C), 53.1 (CH3), 52.9 (CH3), 48.1 (CH), 46.9 (C), 43.5 (CH2), 38.5 (CH2), 19.3 (CH3). HRMS (TOF MS ES+) m/z calcd for C13H20O5Na [M + Na]+: 279.1202, found: 279.1200.
Compound 12.
Compound 12 was obtained as a 7/3 mixture of cis/trans diastereoisomers. Colorless oil; 60% yield. 1H-NMR (300 MHz, CDCl3) δ(ppm): 5.85 (ddd, J = 17.3, 10.2, 8.5 Hz, 1H, cis-12), 5.70 (ddd, J = 17.4, 10.1, 8.1 Hz, 1H, trans-12), 5.09 (d, J = 16.6 Hz, 1H, cis-12), 5.05 (d, J = 8.6 Hz, 1H, cis-12), 5.07–4.95 (m, 2H, trans-12), 3.72 (bs, 6H), 3.68–3.52 (m, 2H, trans-12), 3.61 (dd, J = 11.1, 6.4 Hz, 1H, cis-12), 3.48 (dd, J = 11.1, 6.3 Hz, 1H, cis-12), 2.88–2.74 (m, 1H), 2.54–2.23 (m, 3H), 2.19–1.98 (m, 2H). 13C-NMR (75 MHz, CDCl3) δ(ppm): 173.1 (C), 173.0 (C), 172.9 (C), 172.0 (C), 140.3 (CH), 137.8 (CH), 116.1 (CH2), 115.9 (CH2), 64.4 (CH2), 63.2 (CH2), 59.2 (C), 58.8 (C), 53.0 (CH3), 52.9 (CH3), 47.3 (CH), 46.5 (CH), 45.1 (C), 44.9 (CH), 40.9 (CH2), 39.1 (CH2), 37.1 (CH2), 36.5 (CH2). HRMS (TOF MS ES+) m/z calcd for C12H18O5Na [M + Na]+: 265.1046, found: 265.1054. Compound 12 was oxidized to simplify the 1H-NMR spectrum and confirm the ratio of diastereomers obtained. See ESI† for further details.
Compounds 14 and 15.
Compounds 14 and 15 were both obtained as a mixture of diastereoisomers. Yellowish oil; 55% yield. Compounds 14 and 15 were not separated. 1H-NMR (400 MHz, CDCl3) δ(ppm): 5.90–5.73 (m, 1H, 14 or 15), 5.63–5.48 (m, 1H, 14 or 15), 5.10–4.96 (m, 2H), 3.82–3.72 (m, 1H, 14 or 15), 3.73 (bs, 3H, 14 or 15), 3.71 (bs, 3H), 3.69 (bs, 3H, 14 or 15), 3.37–3.26 (m, 1H, 14 or 15), 2.61–2.39 (m, 2H), 2.35–2.14 (m, 2H), 2.08–1.96 (m, 1H), 1.93–1.72 (m, 1H), 1.69–1.54 (m, 1H), 1.15 (d, J = 4.3 Hz, 3H, 15), 0.96 (d, J = 6.0 Hz, 3H, 14). HRMS (TOF MS ES+) m/z calcd for C13H20O5Na [M + Na]+: 279.1202, found: 279.1210. The mixture of compounds 14 and 15 was oxidized to simplify the 1H-NMR spectrum and confirm both compounds and dr obtained. See ESI† for further details.
Compound 17.
Colorless oil; 50% yield. 1H-NMR (400 MHz, CDCl3) δ(ppm): 5.29 (s, 1H), 5.02 (s, 1H), 4.54 (s, 2H), 4.20 (q, J = 7.1 Hz, 2H), 3.75 (s, 3H), 3.70 (s, 3H), 3.42 (dd, J = 12.0, 4.0 Hz, 1H), 2.39 (ddd, J = 13.2, 4.0, 2.3 Hz, 1H), 2.14 (dt, J = 13.7, 2.6 Hz, 1H), 2.00 (t, J = 14.2 Hz, 1H), 1.91–1.85 (m, 1H), 1.84 (t, J = 14.2 Hz, 1H), 1.31 (t, J = 7.1 Hz, 3H), 0.95 (s, 3H), 0.87 (s, 3H); NOE-diff. experiment: proton irradiated (NOEs observed): H-3, (H-2a, H-5). 13C-NMR (100 MHz, CDCl3) δ(ppm): 171.7 (C), 171.3 (C), 155.1 (C), 143.5 (C), 115.3 (CH2), 74.7 (CH), 71.1 (CH2), 64.2 (CH2), 54.9 (C), 53.0 (CH3), 52.8 (CH3), 44.6 (CH), 39.1 (C), 34.6 (CH2), 32.5 (CH2), 25.7 (CH3), 14.4 (CH3), 12.7 (CH3). HRMS (TOF MS ES+) m/z calcd for C18H28O8Na [M + Na]+: 395.1676, found: 395.1668.
Compound 19.
Colorless oil; 54% yield. 1H-NMR (500 MHz, CDCl3) δ(ppm): 7.31 (t, J = 7.3 Hz, 2H), 7.25–7.20 (m, 1H), 7.14 (dd, J = 8.3, 1.4 Hz, 2H), 5.43 (ddd, J = 17.5, 10.4, 7.1 Hz, 1H), 4.83 (d, J = 16.1 Hz, 1H), 4.81 (d, J = 9.4 Hz, 1H), 3.86 (td, J = 11.2, 4.7 Hz, 1H), 3.82 (s, 3H), 3.75 (s, 3H), 2.74 (ddd, J = 13.1, 4.7, 2.2 Hz, 1H), 2.55–2.44 (m, 2H), 2.27 (dd, J = 11.2, 10.6 Hz, 1H), 1.82 (dd, J = 13.1, 11.2 Hz, 1H), 1.74 (dd, J = 13.4, 12.2 Hz, 1H). NOE-diff. experiment: proton irradiated (NOEs observed): H-7 (H2-8, H-4, H-6b), H-4 (H-2b, H-6b), H-3 (H2-2, H-5). 13C-NMR (125 MHz, CDCl3) δ(ppm): 171.8 (C), 171.4 (C), 140.1 (C), 139.6 (CH), 128.8 (CH), 127.3 (CH), 115.5 (CH2), 71.2 (CH), 57.4 (CH), 54.8 (C), 53.1 (CH3), 52.9 (CH3), 42.3 (CH), 38.1 (CH2), 36.6 (CH2). HRMS (TOF MS ES+) m/z calcd for C18H22O5Na [M + Na]+: 341.1359, found: 341.1359.
Compound 21.
Colorless oil; 58% yield. 1H-NMR (500 MHz, CDCl3) δ(ppm): 5.64 (ddd, J = 17.2, 10.1, 9.0 Hz, 1H), 5.10 (dd, J = 17.2, 1.5 Hz, 1H), 5.03 (dd, J = 10.1, 1.5 Hz, 1H), 3.75 (s, 3H), 3.69 (s, 3H), 3.62 (dd, J = 11.0, 4.4 Hz, 1H), 3.40 (dd, J = 11.0, 6.1 Hz, 1H), 2.41 (ddd, J = 13.5, 6.0, 3.2 Hz, 1H), 2.35–2.27 (m, 1H), 2.10–1.96 (m, 1H), 1.84 (ddd, J = 13.6, 7.1, 3.9 Hz, 1H), 1.71 (td, J = 13.6, 3.9 Hz, 1H), 1.63–1.53 (m, 1H), 1.39–1.14 (m, 2H). 2D-NOESY spectra observed: H-5 (H-9), H-5 (H-6), H-5 (H-3a), H-4 (H2-11), H-4 (H-3b). 13C-NMR (125 MHz, CDCl3) δ(ppm): 172.69 (C), 171.52 (C), 141.76 (CH), 115.87 (CH2), 66.33 (CH2), 54.90 (C), 52.85 (CH3), 52.67 (CH3), 43.34 (CH), 41.80 (CH), 37.44 (CH2), 30.73 (CH2), 25.59 (CH2). HRMS (TOF MS ES+) m/z calcd for C13H20O5Na [M + Na]+: 279.1201, found: 279.1215.
Compound 23.
White solid; m.p. 121–123 °C. 76% yield. 1H-NMR (500 MHz, CDCl3) δ(ppm): 7.65 (d, J = 8.3 Hz, 2H), 7.33 (d, J = 8.3 Hz, 2H), 5.58 (ddd, J = 17.1, 10.4, 8.6 Hz, 1H), 5.12 (d, J = 10.4 Hz, 1H), 5.09 (d, J = 17.1 Hz, 1H), 3.65 (ddd, J = 11.2, 4.8, 1.7 Hz, 1H), 3.54 (dd, J = 10.5, 4.8 Hz, 1H), 3.46 (ddd, J = 11.8, 4.2, 1.7 Hz, 1H), 2.44 (s, 3H), 2.35 (dd, J = 23.9, 11.4 Hz, 2H), 2.21–2.14 (m, 1H), 0.97 (s, 3H), 0.65 (s, 3H). NOE-diff. experiment: proton irradiated (NOEs observed): H-5 (H-6, H-3), H-3 (H-2, H-5). 13C-NMR (125 MHz, CDCl3) δ(ppm): 143.7 (C), 134.3 (CH), 129.8 (CH), 127.7 (CH), 118.7 (CH2), 74.2 (CH), 49.2 (CH), 47.4 (CH2), 46.0 (CH2), 37.1 (C), 25.2 (CH3), 21.7 (CH3), 12.3 (CH3). HRMS (TOF MS ES+) m/z calcd for C16H24NO3S [M + H]+: 310.1471, found: 310.1481.
Compound 25.
Colorless oil; 74% yield. 1H-NMR (400 MHz, CDCl3) δ(ppm): 7.70 (d, J = 8.2 Hz, 2H), 7.31 (d, J = 8.2 Hz, 2H), 5.51 (ddd, J = 17.1, 10.3, 8.5 Hz, 1H), 5.06 (d, J = 10.3 Hz, 1H), 5.00 (d, J = 17.1 Hz, 1H), 3.49 (dd, J = 9.9, 8.0 Hz, 1H), 3.34 (dd, J = 17.9, 10.9 Hz, 2H), 3.29 (d, J = 9.8 Hz, 1H), 3.13 (t, J = 9.9 Hz, 1H), 3.06 (d, J = 9.8 Hz, 1H), 2.57 (dd, J = 17.1, 8.0 Hz, 1H), 2.42 (s, 3H), 0.71 (s, 3H). NOE-diff. experiment: proton irradiated (NOEs observed): H-6 (H-5, H2-9, H3-8), H-4 (H-6, H2-7, H-5, H2-9), H3-8 (H-2, H2-9, H-6). 13C-NMR (100 MHz, CDCl3) δ(ppm): 143.6 (C), 134.3 (CH), 129.7 (CH), 127.5 (CH), 118.4 (CH2), 67.0 (CH2), 56.6 (CH2), 51.0 (CH2), 47.1 (CH), 46.4 (C), 21.6 (CH3), 16.9 (CH3). HRMS (TOF MS ES+) m/z calcd for C15H22NO3S [M + H]+: 296.1314, found: 296.1326.
Compound trans-27.
Vitreous solid; 39% yield. 1H-NMR (500 MHz, CDCl3) δ(ppm): 8.14 (dd, J = 8.5, 1.2 Hz, 2H), 8.02 (dd, J = 8.5, 1.2 Hz, 2H), 7.74 (dd, J = 15.3, 7.2 Hz, 2H), 7.63 (dd, J = 15.3, 7.2 Hz, 4H), 5.70 (ddd, J = 17.2, 10.9, 8.3 Hz, 1H), 5.14 (d, J = 10.9 Hz, 1H), 5.13 (d, J = 17.2 Hz, 1H), 4.27 (d, J = 11.4 Hz, OH), 3.60 (ddd, J = 11.4, 4.9, 2.0 Hz, 1H), 2.92 (dd, J = 16.5, 4.9 Hz, 1H), 2.81 (ddd, J = 12.4, 8.3, 3.8 Hz, 1H), 2.52 (d, J = 18.6 Hz, 1H), 2.49 (t, J = 13.0 Hz, 1H), 2.18 (ddd, J = 15.7, 3.8, 1.7 Hz, 1H), 1.04 (s, 3H), 0.82 (s, 3H). NOE-diff. experiment: proton irradiated, (NOEs observed): H-5, (H-7, H-6), H-3, (H-2, H-6). 13C-NMR (125 MHz, CDCl3) δ(ppm): 137.47 (CH), 135.78 (C), 135.04 (CH), 134.94 (C), 134.84 (CH), 132.04 (CH), 131.75 (CH), 128.83 (CH), 128.79 (CH), 117.67 (CH2), 89.02 (C), 74.24 (CH), 40.32 (CH), 36.98 (C), 28.59 (CH2), 27.34 (CH2), 25.57 (CH3), 19.34 (CH3). HRMS (TOF MS ES+) m/z calcd for C22H26O5S2Na [M + Na]+: 457.1113, found: 457.1100
Compound cis-27.
White solid; m.p. 153–156 °C. 32% yield. 1H-NMR (400 MHz, CDCl3) δ(ppm): 8.07 (d, J = 7.6 Hz, 2H), 8.02 (d, J = 7.6 Hz, 2H), 7.76–7.67 (m, 2H), 7.65–7.56 (m, 4H), 5.64 (ddd, J = 17.3, 10.8, 8.8 Hz, 1H), 5.11 (d, J = 10.8 Hz, 1H), 5.10 (d, J = 17.3 Hz, 1H), 4.24–4.09 (m, 1H), 2.68 (ddd, J = 12.5, 8.8, 3.8 Hz, 1H), 2.52–2.24 (m, 3H), 2.06–1.95 (m, 1H), 1.02 (s, 3H), 0.71 (s, 3H). NOE-diff. experiment: proton irradiated (NOEs observed): H-5, (H-7, H-3, H-6), H-3, (H-5, H-2). 13C-NMR (100 MHz, CDCl3) δ(ppm): 137.0 (CH), 135.9 (C), 134.8 (CH), 134.6 (CH), 131.7 (CH), 131.3 (CH), 128.7 (CH), 117.7 (CH2), 88.6 (C), 73.0 (CH), 45.8 (CH), 37.6 (C), 30.4 (CH2), 27.0 (CH2), 25.3 (CH3), 11.7 (CH3). HRMS (TOF MS ES+) m/z calcd for C22H26O5S2Na [M + Na]+: 457.1113, found: 457.1092.
Compound 29.
Colorless oil; 62% yield. 1H-NMR (400 MHz, CDCl3) δ(ppm): 5.30 (s, 1H), 5.07 (s, 1H), 4.54 (s, 2H), 4.22 (q, J = 7.1 Hz, 2H), 3.87 (dd, J = 12.2, 4.2 Hz, 1H), 3.77 (s, 3H), 3.71 (s, 3H), 3.60 (d, J = 10.8 Hz, 1H), 3.38 (d, J = 10.8 Hz, 1H), 2.42 (ddd, J = 13.2, 4.1, 2.2 Hz, 1H), 2.19 (dt, J = 13.5, 2.7 Hz, 1H), 2.16–2.12 (m, 1H), 2.01 (t, J = 13.1 Hz, 1H), 1.95 (t, J = 12.5 Hz, 1H), 1.32 (t, J = 7.1 Hz, 3H), 0.92 (s, 3H). 2D-NOESY spectra observed: H-3 (H-2a), H-3 (H-5), H-3 (H-11), H-5 (H-11). 13C-NMR (100 MHz, CDCl3) δ(ppm): 171.6 (C), 171.2 (C), 155.2 (C), 143.3 (C), 116.1 (CH2), 71.2 (CH), 71.1 (CH2), 68.3 (CH2), 64.4 (CH2), 54.7 (C), 53.1 (CH3), 52.9 (CH3), 43.1 (C), 39.0 (CH), 34.2 (CH2), 32.4 (CH2), 14.4 (CH3), 9.3 (CH3). HRMS (TOF MS ES+) m/z calcd for C18H28O9Na [M + Na]+: 411.1625, found: 411.1627.
Compound 31.
Yellowish oil; 73% yield. 1H-NMR (400 MHz, CDCl3) δ(ppm): 5.69 (ddd, J = 17.2, 10.2, 8.8 Hz, 1H), 5.09 (d, J = 17.2 Hz, 1H), 5.05 (d, J = 10.2 Hz, 1H), 3.75 (s, 3H), 3.70 (s, 3H), 3.34 (d, J = 11.0 Hz, 1H), 3.29 (d, J = 11.0 Hz, 1H), 2.30–2.20 (m, 2H), 2.15 (ddd, J = 11.0, 3.5, 2.4 Hz, 1H), 1.89 (td, J = 13.7, 3.5 Hz, 1H), 1.82 (t, J = 13.0 Hz, 1H), 1.50 (td, J = 13.9, 3.7 Hz, 1H), 1.35 (dt, J = 13.9, 3.6 Hz, 1H), 0.84 (s, 3H). NOE-diff. experiment: proton irradiated, (NOEs observed): H-7, (H-8, H3-9), H2-10, (H-5, H3-9), H3-9, (H-7, H2-10). 13C-NMR (100 MHz, CDCl3) δ(ppm): 172.7 (C), 171.5 (C), 139.3 (CH), 116.4 (CH2), 71.8 (CH2), 55.0 (C), 52.8 (CH3), 52.6 (CH3), 42.8 (CH), 37.3 (C), 32.4 (CH2), 31.5 (CH2), 26.4 (CH2), 14.9 (CH3). HRMS (TOF MS ES+) m/z calcd for C14H22O5Na [M + Na]+: 293.1365, found: 293.1359.
Acknowledgements
This research was funded by the Ministerio de Ciencia e Innovación (Spain) (project CTQ-2011.22455). I.R.M. thanks the MEC (Spain) for a predoctoral FPU fellowship. A.M. thanks the University of Granada for a postdoctoral contract (‘Contrato Puente’). A.G.C. thanks the MICINN (Spain) for a postdoctoral contract ‘Juan de la Cierva’ and University of Granada.
Notes and references
-
B. M. Trost and I. Fleming, Comprehensive Organic Synthesis, Pergamon Press plc, Oxford, 1991, vol. 4 Search PubMed
.
-
R. G. Larock, Comprehensive Organic Transformations, VCH Publishers, 1989 Search PubMed
.
- S. H. Krake and S. C. Bergmeier, Tetrahedron, 2010, 66, 7337–7360 CrossRef CAS PubMed
.
- I. Fleming, A. Barbero and D. Walter, Chem. Rev., 1997, 97, 2063–2192 CrossRef CAS PubMed
.
- D. Miguel, A. G. Campaña, J. Justicia and J. M. Cuerva, e-EROS. Encycl. Reagents Org. Synth., 2013 DOI:10.1002/047084289X.rd090m.pub2
; For more recent applications of Cp2TiCl, see for example:
(a) J. Streuff, M. Feurer, P. Bichovski, G. Frey and U. Gellrich, Angew. Chem., Int. Ed., 2012, 51, 8661–8664 CrossRef CAS PubMed
;
(b) G. Frey, H.-T. Luu, P. Bichovski, M. Feurer and J. Streuff, Angew. Chem., Int. Ed., 2013, 52, 7131–7134 CrossRef CAS PubMed
;
(c) J. Muñoz-Bascón, C. Hernández-Cervantez, N. M. Padial, M. Álvarez-Corral, A. Rosales, I. Rodríguez-García and J. E. Oltra, Chem.–Eur. J., 2014, 20, 801–810 CrossRef PubMed
;
(d) Y. Zhao and D. J. Weix, J. Am. Chem. Soc., 2014, 136, 48–51 CrossRef CAS PubMed
.
- W. A. Nugent and T. V. RajanBabu, J. Am. Chem. Soc., 1988, 110, 8561–8562 CrossRef CAS
.
- T. V. RajanBabu and W. A. Nugent, J. Am. Chem. Soc., 1989, 111, 4525–4527 CrossRef CAS
.
- T. V. RajanBabu, W. A. Nugent and M. S. Beattie, J. Am. Chem. Soc., 1990, 112, 6408–6409 CrossRef CAS
.
- T. V. Rajanbabu and W. A. Nugent, J. Am. Chem. Soc., 1994, 116, 986–997 CrossRef CAS
.
- A. Gansäuer, H. Bluhm and M. Pierobon, J. Am. Chem. Soc., 1998, 120, 12849–12859 CrossRef
.
- A. Gansäuer, T. Lauterbach, H. Bluhm and M. Noltemeyer, Angew. Chem., Int. Ed., 1999, 38, 2909–2910 CrossRef
.
- A. Gansäuer, H. Bluhm, B. Rinker, S. Narayan, M. Schick, T. Lauterbach and M. Pierobon, Chem.–Eur. J., 2003, 9, 531–542 CrossRef PubMed
.
- A. Cangönül, M. Behlendorf, A. Gansäuer and M. van Gastel, Inorg. Chem., 2013, 52, 11859–11866 CrossRef PubMed
.
- A. Fernández-Mateos, P. Herrero Teijón and R. Rubio González, Tetrahedron, 2013, 69, 1611–1616 CrossRef PubMed
.
- A. Fernández-Mateos, S. E. Madrazo, P. H. Teijón, R. R. Clemente, R. R. González and F. S. González, J. Org. Chem., 2013, 78, 9571–9578 CrossRef PubMed
.
- A. F. Barrero, J. F. Quílez del Moral, E. M. Sánchez and J. F. Arteaga, Eur. J. Org. Chem., 2006, 1627–1641 CrossRef CAS
.
- J. M. Cuerva, J. Justicia, J. L. Oller-López and J. E. Oltra, Top. Curr. Chem., 2006, 264, 63–91 CrossRef CAS
.
- A. Gansäuer, J. Justicia, C.-A. Fan, D. Worgull and F. Piestert, Top. Curr. Chem., 2007, 279, 25–52 CrossRef
.
- S. P. Morcillo, Á. Martínez-Peragón, V. Jakoby, A. J. Mota, C. Kube, J. Justicia, J. M. Cuerva and A. Gansauer, Chem. Commun., 2014, 50, 2211–2213 RSC
.
- J. Justicia, A. Rosales, E. Buñuel, J. L. Oller-López, M. Valdivia, A. Haïdour, J. E. Oltra, A. F. Barrero, D. J. Cárdenas and J. M. Cuerva, Chem.–Eur. J., 2004, 10, 1778–1788 CrossRef CAS PubMed
.
- J. Justicia, J. L. Oller-López, A. G. Campaña, J. E. Oltra, J. M. Cuerva, E. Buñuel and D. J. Cárdenas, J. Am. Chem. Soc., 2005, 127, 14911–14921 CrossRef CAS PubMed
.
- T. Jiménez, S. P. Morcillo, A. Martín-Lasanta, D. Collado-Sanz, D. J. Cárdenas, A. Gansäuer, J. Justicia and J. M. Cuerva, Chem.–Eur. J., 2012, 18, 12825–12833 CrossRef PubMed
.
- J. Justicia, T. Jiménez, D. Miguel, R. Contreras-Montoya, R. Chahboun, E. Álvarez-Manzaneda, D. Collado-Sanz, D. J. Cárdenas and J. M. Cuerva, Chem.–Eur. J., 2013, 19, 14484–14495 CrossRef CAS PubMed
.
-
(a) J. Justicia, L. Á. de Cienfuegos, A. G. Campaña, D. Miguel, V. Jakoby, A. Gansäuer and J. M. Cuerva, Chem. Soc. Rev., 2011, 40, 3525–3537 RSC
;
(b) S. P. Morcillo, D. Miguel, A. G. Campaña, L. Álvarez de Cienfuegos, J. Justicia and J. M. Cuerva, Org. Chem. Front., 2014, 1, 15–33 RSC
.
- V. Domingo, J. F. Arteaga, J. L. López Pérez, R. Peláez, J. F. Quílez del Moral and A. F. Barrero, J. Org. Chem., 2012, 77, 341–350 CrossRef CAS PubMed
.
- M. C. Pérez Morales, J. V. Catalán, V. Domingo, M. Jaraíz, M. M. Herrador, J. F. Quílez del Moral, J. López-Pérez and A. F. Barrero, Chem.–Eur. J., 2013, 19, 6598–6612 CrossRef PubMed
.
- M. Paradas, A. G. Campaña, R. E. Estévez, L. Alvarez de Cienfuegos, T. Jiménez, R. Robles, J. M. Cuerva and J. E. Oltra, J. Org. Chem., 2009, 74, 3616–3619 CrossRef CAS PubMed
.
- M. Paradas, A. G. Campaña, M. L. Marcos, J. Justicia, A. Haidour, R. Robles, D. J. Cárdenas, J. E. Oltra and J. M. Cuerva, Dalton Trans., 2010, 39, 8796 RSC
.
- A. F. Barrero, J. M. Cuerva, M. M. Herrador and M. V. Valdivia, J. Org. Chem., 2001, 66, 4074–4078 CrossRef CAS
.
- A. F. Barrero, A. Rosales, J. M. Cuerva and J. E. Oltra, Org. Lett., 2003, 5, 1935–1938 CrossRef CAS PubMed
.
- K. V. Bhaskar and L. N. Mander, Tetrahedron Lett., 1996, 37, 719–722 CrossRef CAS
.
- It is known that in the presence of a better H-atom source the reduction process yielding alcohols is favored:
(a) J. Justicia, J. E. Oltra, A. F. Barrero, A. Guadaño, A. González-Coloma and J. M. Cuerva, Eur. J. Org. Chem., 2005, 712–718 CrossRef CAS
;
(b) T. Jiménez, A. G. Campaña, B. Bazdi, M. Paradas, D. Arráez-Román, A. Segura-Carretero, A. Fernández-Gutiérrez, J. E. Oltra, R. Robles, J. Justicia and J. M. Cuerva, Eur. J. Org. Chem., 2010, 4288–4295 CrossRef
Nevertheless, in this case the reduction process can take place
on intermediate radicals type-V (Scheme 1), thus competing with the desired cyclization reaction.
- K. Daasbjerg, H. Svith, S. Grimme, M. Gerenkamp, C. Mück-Lichtenfeld, A. Gansäuer, A. Barchuk and F. Keller, Angew. Chem., Int. Ed., 2006, 45, 2041–2044 CrossRef CAS PubMed
.
- A. L. J. Beckwith and C. H. Schiesser, Tetrahedron, 1985, 41, 3925–3941 CrossRef CAS
.
- D. C. Spellmeyer and K. N. Houk, J. Org. Chem., 1987, 52, 959–974 CrossRef CAS
.
Footnote |
† Electronic supplementary information (ESI) available. See DOI: 10.1039/c4qo00012a |
|
This journal is © the Partner Organisations 2014 |