Improving alkynyl(aryl)iodonium salts: 2-anisyl as a superior aryl group†
Received
13th March 2014
, Accepted 6th May 2014
First published on 6th May 2014
Abstract
The majority of alkynyl(aryl)iodonium salts reported in the literature are derived from iodobenzene. This article describes the effects of varying this iodoarene building block on the synthesis, reactivity and stability of these salts. Two procedures to synthesize a variety of known and novel alkynyl(aryl)iodonium tosylates directly from the iodoarene are reported. In the reactions of these salts, those derived from 2-iodoanisole gave superior results than the others tested in every reaction. Isothermal microcalorimetry indicated that these novel salts were significantly more stable and less prone to decomposition than all of the other derivatives.
Introduction
Interest in hypervalent iodine chemistry has surged in recent years with new iodine(III) and iodine(V) compounds being reported regularly alongside novel reactions using these species.1 There are two main types of hypervalent organoiodine compounds: those with one carbon ligand and those with two. Compounds with one carbon ligand include iodobenzene diacetate (also called diacetoxyiodobenzene or phenyliodide diacetate) and Dess–Martin periodinane. Generally, these types of hypervalent iodine compounds are powerful oxidants. Hypervalent iodine compounds with two carbon ligands are commonly called iodonium salts and these include diaryliodonium salts, alkenyl(aryl)iodonium salts and alkynyl(aryl)iodonium salts. These compounds are not strong oxidants.
Iodonium salts are increasingly popular reagents in organic synthesis because of the range of useful reactivities that they exhibit.2 The most investigated iodonium salts are the diaryliodonium salts, which can, in principal, donate either of their aryl groups in reactions with nucleophiles (Scheme 1).3 Whereas, in general, alkynyl(aryl)- and alkenyl(aryl)iodonium salts only donate the alkyne or alkenyl groups respectively. This means that the aryl group is, essentially, a spectator group in these two types of iodonium salts. However, the effect of changing this spectator group on the reactivity of these salts has not been studied. Indeed, the majority of research with these two types of salts has been with those derived from iodobenzene.4 Cyclic species such as triisopropylsilylethynyl-1,2-benziodoxol-3(1H)-one (TIPS-EBX) are known, but these have only been reported in alkynylation reactions.5
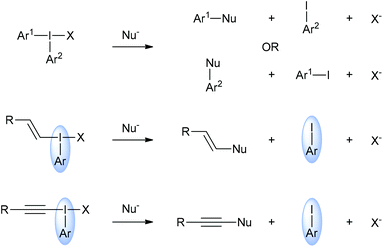 |
| Scheme 1 General reactivities of iodonium salts with nucleophiles highlighting the “spectator” role of the aryl iodide in alkenyl(aryl)- and alkynyl(aryl)iodonium salts. | |
Results and discussion
We identified two different reactions from the literature that alkynyl(aryl)iodonium salts undergo and decided to compare and contrast the effect of varying the aryl moiety on the reaction outcomes. The two reactions were (i) conjugate addition of a nucleophile followed by carbene formation and rearrangement to generate a new alkyne6 and (ii) conjugate addition of a nucleophile followed by carbene formation and intramolecular 1,5-insertion into a C–H bond (Scheme 2).7
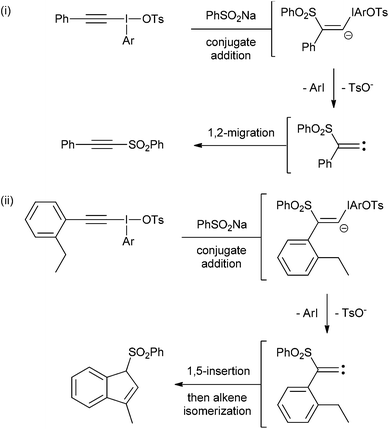 |
| Scheme 2 Two reactions of alkynyl(aryl)iodonium salts. | |
Our first challenge was to prepare the requisite alkynyliodonium salts necessary for this study. There are several synthetic methods available; however, as alluded to earlier, their effectiveness with regards to preparing salts from different aryl iodides has not been reported. We elected to use the method reported by Merritt and Olofsson for direct formation of the salts from terminal alkynes and aryl iodides using 3-chloroperbenzoic acid and 4-toluenesulfonic acid in dichloromethane.8 This procedure was only reported for the preparation of salt 1a but its simplicity makes it an attractive method. However, our attempts to make pure samples of derivatives by this method failed in most cases, with the major impurity being the hydroxy(tosyloxy)aryliodine(III) compound. In 2012, Bouma and Olofsson published a new protocol using alkynyl boronates instead of terminal alkynes and the authors stated that this was a general route to high purity salts.9 Other methods have been reported which also require initial preparation of the corresponding alkynyl silanes,10 borons11 or stannanes.12 We wished to develop the method using terminal alkynes as this is more direct and atom efficient.
During our study, we noted that aryl iodides bearing electron-donating substituents behaved differently to those with electron-withdrawing groups; therefore, we optimized reaction conditions for these derivatives separately. For aryl iodides with electron-donating substituents, slow addition of the oxidant over several hours to the reaction mixture at 0 °C provided high purity iodonium salts upon crystallization from the reaction mixture (Table 1). This slow addition of the oxidant to a cold solution of the other components was found to effectively inhibit thermal decomposition of the intermediate iodine(III) species formed during this process. Additionally, by using an excess of the alkyne it was found, as expected, that the amount of the major hydroxy(tosyloxy)aryliodine(III) impurity was reduced, leading to products of higher purity and in superior yield. If necessary, recrystallization effectively removed any trace impurities.
Table 1 Synthesis of alkynyl(aryl)iodonium tosylates with electron-donating aryl substituentsa
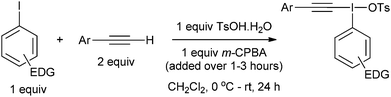
|
Yields are for pure isolated compounds.
|
|
For aryl iodides with electron-withdrawing substituents, the hydroxy(tosyloxy)iodine(III) species were first prepared in situ and then treated with the alkyne to generate the iodonium salts in high purity after crystallization from the reaction mixture (Table 2). Where the conditions reported by Merritt and Olofsson were applied to the synthesis of these aryl iodides it was found that the material obtained was of very low purity; as little as 50%. We assume, therefore, that the presence of electron withdrawing substituents reduces the rate of formation of the alkynyliodonium salts from the corresponding hydroxy(tosyloxy)iodine(III) species and that the low purities were a consequence of incomplete reactions. By forming the hydroxy(tosyloxy)iodine(III) species first, we reduce the amount of time for thermal decomposition of the product alkynyliodonium salts, while an excess of the alkyne results in further gains in both the yield and purity of the product alkynyliodonium salts obtained. Again, any impurities present could be removed by recrystallization, but this was rarely necessary. All of the alkynyliodonium salts synthesised could be stored indefinitely at −20 °C.
Table 2 Synthesis of alkynyl(aryl)iodonium tosylates with electron-withdrawing aryl substituentsa
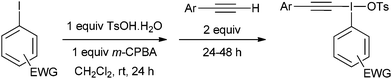
|
Yields are for pure isolated compounds.
|
|
With these salts in hand, the addition–rearrangement reaction with sodium phenylsulfinate was investigated (Table 3).6 The iodobenzene derived salt 1a worked well delivering an average of 81% yield over three runs, with 74% yield of isolated compound (entry 1). The 2-methoxy derivative 1b provided a better yield of 90%, with 83% yield of isolated compound (entry 2) whereas the 3- and 4-methoxy derivatives 1c and 1d both led to diminished yields (entries 3 and 4). This suggests that the improvement in yield for 1b is not due to electronic effects. Indeed, 1b appeared to show increased solubility compared to 1c and 1d. The nitro derivative 1e was a poor performer (entry 5), whereas m-xylyl derivative 1f delivered 73% product, p-tolyl 1g gave a superior 88% and 4-chloro 1h was similar to parent 1a with 79%.
Table 3 Addition–rearrangement reaction
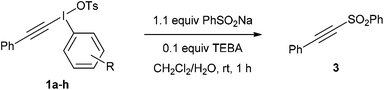
|
Entry |
R |
Salt |
Yielda (%) |
Yields are an average of three runs and were determined by 1H NMR analysis of the crude reaction mixtures with reference to a standard. Yields of isolated compounds are given in parentheses.
|
1 |
H |
1a
|
81 (74) |
2 |
2-OMe |
1b
|
90 (83) |
3 |
3-OMe |
1c
|
49 |
4 |
4-OMe |
1d
|
53 |
5 |
2-NO2 |
1e
|
44 |
6 |
3,5-diMe |
1f
|
73 |
7 |
4-Me |
1g
|
88 |
8 |
4-Cl |
1h
|
79 |
These results suggest that 2-iodoanisole is the best “spectator” group of those tested in this reaction. To get further insights into salt 1b a single crystal X-ray structure was obtained (Fig. 1).13 This iodonium salt exists as a dimer in the solid state with the bond angles and lengths around the methoxy substituent being distorted somewhat suggesting some coordination to the I(III) center. The C(–I)–C–O bond angle is 116.62° compared to 120.83° for the C(–I)–C–H bond angle. Likewise, the C(–I)–C(–O)–C bond angle is 117.93° and the C(–I)–C(–H)–C bond angle is 118.27°. The distance between the iodine and oxygen atoms is 3.029 Å, which is reasonable for a van der Waals interaction. Recently, Zhdankin and co-workers demonstrated the synthesis and chemistry of iodonium ylides with ortho-alkoxy groups bound to the aryl ring.14 The reported crystal structure shows similar distortions around the I(III) center and the ortho-n-propoxy oxygen I(III) distance is reported as 2.978 Å. The authors note that these species display superior solubility and reactivity in comparison with their phenyl analogs.
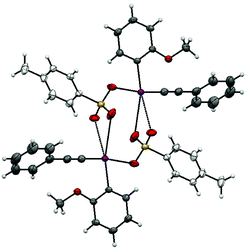 |
| Fig. 1 X-ray crystal structure of 1b. | |
The addition–C–H insertion reaction was investigated next (Table 4).7 In our hands, this reaction proved difficult with only 12% yield being achieved with salt 2a (entry 1). However, the 2-methoxy salt 2b once again proved to be superior and 51% yield of product was obtained (entry 2). The other salts provided low amounts of products, with xylyl derivative 2e furnishing <5% (entry 5).
Table 4 Addition/C–H insertion reaction
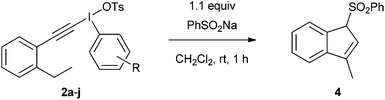
|
Entry |
R |
Salt |
Yielda (%) |
Yields are an average of three runs and were determined by 1H NMR analysis of the crude reaction mixtures with reference to a standard. Yields of isolated compounds are given in parentheses.
|
1 |
H |
2a
|
12 (5) |
2 |
2-OMe |
2b
|
51 (46) |
3 |
3-NO2 |
2c
|
32 |
4 |
4-NO2 |
2d
|
10 |
5 |
3,5-diMe |
2e
|
<5 |
6 |
4-Cl |
2f
|
32 |
In order to investigate the effect of the counterions on reaction yields, alkynyl(phenyl)iodonium salts were prepared from trimethyl(phenylethynyl)silane by reaction with iodosobenzene followed by treatment with aqueous solutions of a variety of lithium or sodium salts. The crude products were directly treated with sodium benzenesulfinate to effect the addition–rearrangement process (Table 5). The product 3 was formed in up to 50% yield from 7 (entry 1). The yields for the different salts were very close, with only a 5% difference, apart from for the mesylate which was 13% lower than the best (entry 4).
Table 5 Effect of counterion on overall yield
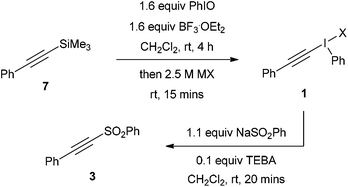
|
Entry |
MX |
Overall yielda (%) |
Yields are an average of three runs and were determined by 1H NMR analysis of the crude reaction mixtures with reference to a standard. Yields of isolated compounds are given in parentheses.
|
1 |
NaBF4 |
50 (36) |
2 |
LiOTf |
47 |
3 |
NaOTs |
45 |
4 |
NaOMs |
37 |
5 |
NaNO3 |
46 |
6 |
NaPF6 |
48 |
This process was then repeated but with variation of the iodosoarene and the tetrafluoroborate counterion was used (Table 6). The different iodosoarenes required were synthesized by hydrolysis of the corresponding iodoarene diacetates with 3 M NaOH solution.15 Yields varied from 0–92% for this process and the products were insoluble polymeric solids. Upon running the test reaction, a 50% overall yield of 3 was obtained for the phenyl parent and 36% yield was isolated (entry 1). The 2-iodosoanisole appeared to decompose upon addition of the Lewis acid at room temperature, therefore the Lewis acid was added dropwise at −78 °C to the solution of silylalkyne 7 and 2-iodosoanisole. The reaction mixture was then warmed to room temperature and the aqueous NaBF4 solution added. Addition of the NaSO2Ph to this salt at room temperature led to addition/insertion occurring in a remarkable 96% overall yield (entry 2). The other iodosoarenes tested gave moderate yields.
Table 6 Conversion of silylalkyne 7 to sulfone 3 without isolation of the alkynyliodonium salt 1
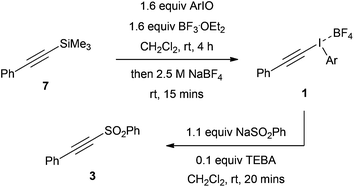
|
Entry |
Ar |
Overall yielda (%) |
Yields are an average of three runs and were determined by 1H NMR analysis of the crude reaction mixtures with reference to a standard. Yields of isolated compounds are given in parentheses.
First stage of the iodonium salt formation performed at −78 °C.
|
1 |
Ph |
50 (36) |
2 |
2-MeOC6H4 |
96b (87) |
3 |
2-NO2C6H4 |
38 |
4 |
3,5-diMeC6H3 |
48 |
5 |
4-MeC6H4 |
49 |
6 |
4-ClC6H4 |
62 |
In a similar fashion, the addition–insertion reaction was investigated starting from the silane 8 but without isolation of the intermediate alkynyliodonium salt 9 (Table 7). Upon running the reaction, a 30% overall yield of 10 was obtained for the phenyl parent (entry 1). The 2-iodoanisole derivative gave a superior 71% yield, 64% isolated, which was substantially better than all the other derivatives tested (entry 2). This result is particularly notable for a non-activated aliphatic C–H insertion reaction occurring at room temperature. A small amount of the 1,2-rearrangement product was also formed in this reaction (<20%).
Table 7 Conversion of silylalkyne 8 to cyclopentene 10 without isolation of the alkynyliodonium salt 9
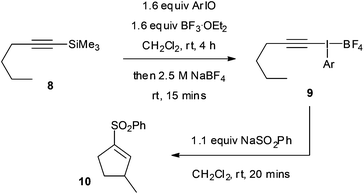
|
Entry |
Ar |
Overall yielda (%) |
Yields are an average of three runs and were determined by 1H NMR analysis of the crude reaction mixtures with reference to a standard. Yields of isolated compounds are given in parentheses.
First stage of the iodonium salt formation performed at −78 °C.
|
1 |
Ph |
30 (22) |
2 |
2-MeOC6H4 |
71b (64) |
3 |
2-NO2C6H4 |
35 |
4 |
3,5-diMeC6H3 |
57 |
5 |
4-MeC6H4 |
54 |
6 |
4-ClC6H4 |
46 |
In order to rationalize these results, we decided to analyze the alkynyl(aryl)iodonium salts by isothermal microcalorimetry (IMC). Holding the samples for seven days at 30 °C under an air atmosphere both as solids (Fig. 2) and in solution (Fig. 3) led to rapid decomposition of all of the salts apart from the 2-iodoanisole derivative. This salt was unchanged after seven days, whereas the others decomposed within minutes. This remarkable stability is postulated to arise from coordination of the oxygen atom to the iodine(III) centre (vide supra) as the corresponding 4-iodoanisole derivative rapidly decomposed, suggesting that simple electronic effects are not responsible. Assuming that this effect is also present in the iodonium reaction intermediates, we can rationalize the observed yields in the reactions studied by consideration of the stabilities of the iodine(III) species and the likelihood of their undergoing unproductive decomposition before the desired reaction. These species are not sensitive to water or air and no decompositions have been detected by exposing them to daylight, although storage in the dark is recommended.
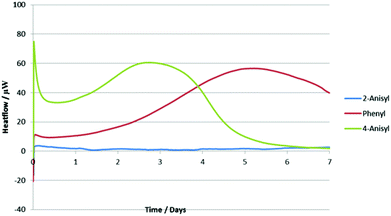 |
| Fig. 2 IMC data for three alkynyl(aryl)iodonium salts in the solid state at 30 °C. | |
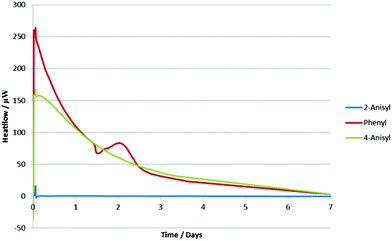 |
| Fig. 3 IMC data for three alkynyl(aryl)iodonium salts in 1,2-dichloroethane at 30 °C. | |
Conclusions
We have developed two new modified procedures for the preparation of alkynyl(aryl)iodonium tosylates directly from iodoarenes and terminal acetylenes. We have also demonstrated that the reactivity of alkynyl(aryl)iodonium salts vary considerably depending on which aryl iodide they are derived from.16 Thus, the aryl iodide is not merely a spectator group but has a profound impact on the reactivity. Importantly, the stability of the iodine(III) species has been shown to be critical for achieving high yields. Of those tested, 2-iodoanisole has been shown to be the optimum choice as it provided augmented yields of products in subsequent reactions compared to other derivatives. It is expected that the use of 2-iodoanisole derived alkynyl(aryl)iodonium salts, and other iodine(III) species, will deliver superior results in other reactions.14
Experimental
General methods
1H NMR spectra were recorded at 400 MHz. Chemical shifts are reported in ppm from tetramethylsilane with the solvent resonance as the internal standard (CDCl3: 7.26 ppm). 13C NMR were recorded with complete proton decoupling. Chemical shifts are reported in ppm from tetramethylsilane with the solvent as the internal standard (CDCl3: 77.4 ppm). Mass spectrometry (m/z) was performed in ESI mode, with only molecular ions being reported. Infrared (IR) spectra νmax are reported in cm−1. Petroleum ether refers to the fraction boiling at 40–60 °C. All purchased reagents were used as received without further purification. All reactions were performed under a N2 atmosphere.
Preparation of alkynyl(aryl)iodonium salts with electron-donating substituents.
To a solution of iodoarene (4.90 mmol, 1 equiv.), TsOH·H2O (0.93 g, 4.90 mmol, 1 equiv.) and an aryl alkyne (9.80 mmol, 2 equiv.) in CH2Cl2 (25 mL) at 0 °C, was added m-CPBA (70–75% purity, 1.22 g, 4.90 mmol, 1 equiv.) slowly over a period of 1 hour. The resulting solution was slowly warmed to room temperature and stirred for 24 h after which it was reduced to half volume (approx. 12 mL) in vacuo at ambient temperature. Diethyl ether (12 mL) was added to this solution in a dropwise manner, with gentle swirling. The resulting homogeneous solution was again reduced to half volume in vacuo at ambient temperature, upon which time crystals started to be formed. Over a period of one hour, diethyl ether (75 mL) was added with gentle swirling, and the resulting suspension was allowed to stand for 1 h. The precipitate was filtered, washed with diethyl ether and dried under vacuum, yielding the product as a powder. Analytically pure material was obtained by recrystallization in CH2Cl2 and ether.
Preparation of alkynyl(aryl)iodonium salts with electron-donating substituents.
To a solution of iodoarene (4.90 mmol, 1 equiv.), TsOH·H2O (0.93 g, 4.90 mmol, 1 equiv.) in CH2Cl2 (25 mL) was added m-CPBA (70–75% purity, 1.22 g, 4.90 mmol, 1 equiv.) in one portion. The solution was allowed to stir for 24 hours, upon which time an aryl alkyne (9.80 mmol, 2 equiv.) was added, followed by a further 24 hours stirring. Removal of the volatiles in vacuo at ambient temperature yielded a residue, which was dissolved in the minimum amount of CH2Cl2 and added dropwise to diethyl ether (75 mL). After 2 h, the precipitate was filtered, washed with diethyl ether and dried under vacuum, yielding the product as a powder. Analytically pure material was obtained by recrystallization in CH2Cl2 and ether.
General synthesis of 2-(benzenesulfonyl)ethynylbenzene (3) from trimethyl(phenylethynyl)silane (7).
To a suspension of a fine powder of iodosylarene (0.5 mmol) in CH2Cl2 (5 mL) under an inert atmosphere was added trimethyl(phenylethynyl)silane 7 (0.052 g, 0.3 mmol), then, over a period of 5 min, boron trifluoride etherate (0.5 mmol). [For 2-(iodosyl)anisole, the suspension was cooled to −78 °C and boron trifluoride was added over a period of 1 h.] The reaction was allowed to stir for 4 h, which yielded a bright yellow homogenous solution. An aqueous solution of a sodium or lithium salt (2.5 M, 1.8 mmol) was added and the resulting emulsion was stirred vigorously for 15 min. The aqueous layer was separated and extracted with CH2Cl2 (2 × 5 mL). The combined organic layers were washed with brine, dried over MgSO4, filtered and concentrated in vacuo to yield the crude alkynyl(aryl)iodonium salts as viscous yellow oils. The crude residue was dissolved in CH2Cl2 (2 mL) and a solution of benzenesulfinic acid sodium salt (0.019 g, 0.116 mmol) and triethylbenzyl ammonium chloride (TEBA) (0.0024 g, 0.011 mmol) in water (1 mL) was added. The reaction mixture was stirred at room temperature for 1 hour, after which time the aqueous layer was separated and extracted with CH2Cl2 (2 × 5 mL). The combined organic layers were washed with water, then brine, and dried over anhydrous MgSO4, filtered and concentrated in vacuo, which yielded a viscous yellow oil. Purification by flash column chromatography (9
:
1 petroleum ether–ethyl acetate) provided a pale yellow solid. Melting point: 70–71 °C. 1H NMR (400 MHz, CDCl3): δ 8.11 (dd, 2H, J = 7.3 Hz, J = 1.3 Hz), 7.73–7.70 (m, 1H), 7.64–7.61 (m, 2H), 7.55 (dd, 2H, J = 7.1 Hz, J = 1.3 Hz), 7.52–7.48 (m, 1H), 7.41–7.38 (m, 2H). 13C NMR (100 MHz, CDCl3): δ 141.7, 134.1, 132.7, 131.6, 129.3, 128.7, 127.4, 117.8, 93.5, 85.3. HRMS: cald for C14H10NaO2S [MNa]+ 265.0294; found 265.0303.
General synthesis of 1-benzenesulfonyl-3-methyl-indene (4) from 1-ethyl-2-ethynylbenzene(aryl)iodonium tosylates (2).
To a solution of 1-ethyl-2-ethynylbenzene(aryl)iodonium tosylate 2 (0.099 mmol) in CH2Cl2 (2 mL) was added benzenesulfinic acid sodium salt (0.018 g, 0.11 mmol). The suspension was stirred at room temperature for one hour upon which time deionized water (2 mL) was added. After an additional 5 min the aqueous layer was separated and extracted with CH2Cl2 (2 × 5 mL). The combined organic layers were washed with brine, dried over MgSO4, filtered and concentrated in vacuo, which yielded a yellow oil. Purification by flash column chromatography (9
:
1 petroleum ether–ethyl acetate) furnished a pale yellow liquid. 1H NMR (400 MHz, CDCl3): δ 7.77 (d, 1H, J = 6.7 Hz), 7.41–7.35 (m, 3H), 7.26–7.15 (m, 4H), 6.97 (d, 1H, J = 6.3 Hz), 6.05 (s, 1H), 4.91 (s, 1H), 1.88 (s, 3H). 13C NMR (100 MHz, CDCl3): δ 146.5, 145.6, 136.0, 135.0, 133.4, 129.1, 128.9, 127.8, 126.3, 125.6, 122.5, 119.5, 72.4, 12.8. HRMS: cald for C16H14SO2Na [MNa]+ 293.0607; found 293.0609.
General synthesis of (3-methylcyclopenten-1-yl)sulfonylbenzene (10) from alkynylsilane (8).
To a suspension of an iodosylarene (0.5 mmol) in CH2Cl2 (5 mL) under an inert atmosphere was added hex-1-yn-1-yltrimethylsilane 8 (0.046 g, 0.3 mmol), then, over a period of 5 min, boron trifluoride etherate (0.5 mmol). [For 2-(iodosyl)anisole, the suspension was cooled to −78 °C and boron trifluoride was added over a period of 1 h.] The reaction was stirred for 4 h, which yielded a bright yellow homogenous solution. An aqueous solution of sodium tetrafluoroborate (2.5 M, 1.8 mmol) was added to the mixture and the resultant emulsion was stirred vigorously for 15 min. The aqueous layer was separated and extracted with CH2Cl2 (2 × 5 mL). The combined organic layers were washed with brine, dried over MgSO4, filtered and concentrated in vacuo at ambient temperature, yielding a viscous yellow oil, which was dissolved in CH2Cl2 (3 mL). To this solution was added benzenesulfinic acid sodium salt (0.059 g, 0.36 mmol). The suspension was held at room temperature for 20 minutes upon which time deionized water (3 mL) was added. After an additional five min the aqueous layer was separated and extracted with CH2Cl2 (2 × 5 mL). The combined organic layers were washed with brine, dried over MgSO4, filtered and concentrated in vacuo, which yielded a yellow oil. Purification by flash column chromatography (9
:
1 petroleum ether–ethyl acetate) furnished a colorless oil. 1H NMR (400 MHz, CDCl3): δ 7.92 (d, 2H, J = 7.4 Hz), 7.67–7.55 (m, 3H), 6.66 (d, 1H, J = 1.8 Hz), 3.01–2.92 (m, 1H), 2.62–2.56 (m, 1H), 2.54–2.45 (m, 1H), 2.30–2.22 (m, 1H), 1.59–1.53 (m, 1H), 1.11 (d, 3H, J = 7.0 Hz). 13C NMR (100 MHz, CDCl3): δ 148.1, 143.5, 139.6, 133.3, 129.1, 127.9, 40.6, 32.4, 30.4, 19.5. HRMS: cald for C12H14NaO2S [MNa]+ 245.0607; found 245.0618.
Acknowledgements
We thank Prof. Craig Rice for assistance with the X-ray crystal structures, Mr James Rooney for assistance with the isothermal microcalorimetry experiments and the University of Huddersfield for funding. We also thank Miss Olivia Davis for experimental assistance and the Nuffield Foundation for a research placement bursary.
Notes and references
- For reviews on hypervalent iodine chemistry, see:
(a) A. Parra and S. Reboredo, Chem. – Eur. J., 2013, 19, 17244 CrossRef CAS PubMed;
(b) V. V. Zhdankin, J. Org. Chem., 2011, 76, 1185 CrossRef CAS PubMed;
(c) V. V. Zhdankin, ARKIVOC, 2009, i, 1 CrossRef;
(d) T. Dohi and Y. Kita, Chem. Commun., 2009, 2073 RSC;
(e) V. V. Zhdankin and P. J. Stang, Chem. Rev., 2008, 108, 5299 CrossRef CAS PubMed;
(f) T. Wirth, Angew. Chem., Int. Ed., 2005, 44, 3656 CrossRef CAS PubMed;
(g) D. Magdziak, S. J. Meek and T. R. R. Pettus, Chem. Rev., 2004, 104, 1383 CrossRef CAS PubMed;
(h) A. N. French, S. Bissmire and T. Wirth, Chem. Soc. Rev., 2004, 33, 354 RSC;
(i) V. V. Zhdankin and P. J. Stang, Chem. Rev., 2002, 102, 2523 CrossRef CAS PubMed;
(j) G. F. Koser, Aldrichimica Acta, 2001, 34, 89 CAS;
(k) V. V. Zhdankin and P. J. Stang, Chem. Rev., 1996, 96, 1123 CrossRef PubMed.
- For reviews of iodonium salts in organic synthesis, see:
(a) M. S. Yusubov, A. V. Maskaev and V. V. Zhdankin, ARKIVOC, 2011, i, 370 CrossRef;
(b) E. A. Merritt and B. Olofsson, Angew. Chem., Int. Ed., 2009, 48, 9052 CrossRef CAS PubMed;
(c) T. Okuyama, Acc. Chem. Res., 2002, 35, 12 CrossRef CAS PubMed;
(d) M. Ochiai, J. Organomet. Chem., 2000, 611, 494 CrossRef CAS;
(e) V. V. Grushin, Chem. Soc. Rev., 2000, 29, 315 RSC;
(f) N. S. Pirkuliev, V. K. Brel and N. S. Zefirov, Russ. Chem. Rev., 2000, 69, 105 CrossRef CAS PubMed;
(g) V. V. Zhdankin and P. J. Stang, Tetrahedron, 1998, 54, 10927 CrossRef CAS;
(h) T. Umemoto, Chem. Rev., 1996, 96, 1757 CrossRef CAS PubMed.
- M. Fujita, E. Mishima and T. Okuyama, J. Phys. Org. Chem., 2007, 20, 241 CrossRef CAS.
- Selected examples of reactions with alkynyl(phenyl)iodonium salts:
(a) B. L. Williamson, P. J. Stang and A. M. Arif, J. Am. Chem. Soc., 1993, 115, 2590 CrossRef CAS;
(b) B. L. Williamson, R. R. Tywinski and P. J. Stang, J. Am. Chem. Soc., 1994, 116, 93 CrossRef CAS;
(c) M. Ochiai, K. Miyamoto, T. Suefuji, S. Sakamoto, K. Yamaguchi and M. Shiro, Angew. Chem., Int. Ed., 2003, 42, 2191 CrossRef CAS PubMed;
(d) I. F. D. Hyatt and M. P. Croatt, Angew. Chem., Int. Ed., 2012, 51, 7511 CrossRef CAS PubMed. Selected examples of reactions with alkenyl(phenyl)iodonium salts:
(e) M. G. Suero, E. D. Bayle, B. S. L. Collins and M. J. Gaunt, J. Am. Chem. Soc., 2013, 135, 5332 CrossRef CAS PubMed;
(f) T. Okuyama and M. Fujita, Acc. Chem. Res., 2005, 38, 679 CrossRef CAS PubMed.
- For a review of the chemistry of alkynyl 1,2-benziodoxolones see: J. P. Brand and J. Waser, Chem. Soc. Rev., 2012, 41, 4165 RSC ; for an example of their use as electrophilic alkynylating reagents see: J. P. Brand, C. Chevalley, R. Scopelliti and J. Waser, Chem. – Eur. J., 2012, 18, 5655 CrossRef CAS PubMed.
- Z. Liu and Z. Chen, Synth. Commun., 1992, 22, 1997 CrossRef CAS.
-
(a) A. E. Koumbis, C. M. Kyzas, A. Savva and A. Varvoglis, Molecules, 2005, 10, 1340 CrossRef CAS. For other examples, see:
(b) D. J. Wardrop and J. Fritz, Org. Lett., 2006, 8, 3659 CrossRef CAS PubMed;
(c) K. S. Feldman, A. L. Perkins and K. M. Masters, J. Org. Chem., 2004, 69, 7928 CrossRef CAS PubMed;
(d) K. S. Feldman, J. C. Saunders and M. L. Wrobleski, J. Org. Chem., 2002, 67, 7096 CrossRef CAS PubMed;
(e) R. R. Tykwinski, J. A. Whiteford and P. J. Stang, J. Chem. Soc., Chem. Commun., 1993, 1800 RSC;
(f) M. Ochiai, M. Kunishima, Y. Nagao, K. Fuji, M. Shiro and E. Fujita, J. Am. Chem. Soc., 1986, 108, 8281 CrossRef CAS.
- E. A. Merritt and B. Olofsson, Eur. J. Org. Chem., 2011, 3690 CrossRef CAS.
- M. J. Bouma and B. Olofsson, Chem. – Eur. J., 2012, 18, 14242 CrossRef CAS PubMed.
-
(a) M. Ochiai, M. Kunishima, K. Sumi, Y. Nagao, E. Fujita, M. Arimoto and H. Yamaguchi, Tetrahedron Lett., 1985, 26, 4501 CrossRef CAS;
(b) L. Rebrovic and G. F. Koser, J. Org. Chem., 1984, 49, 4700 CrossRef CAS;
(c) A. E. Koumbis, C. M. Kyzas, A. Savva and A. Varvoglis, Molecules, 2005, 10, 1340 CrossRef CAS.
-
(a) H.-J. Frohn and V. V. Bardin, Z. Anorg. Allg. Chem., 2008, 634, 82 CrossRef CAS;
(b) M. Yoshida, K. Osafune and S. Hara, Synthesis, 2007, 1542 CrossRef CAS PubMed.
-
(a) P. J. Stang, B. L. Williamson and V. V. Zhdankin, J. Am. Chem. Soc., 1991, 113, 5870 CrossRef CAS;
(b) K. S. Feldman, M. M. Bruendl, K. Schildknegt and A. C. Bohnstedt, J. Org. Chem., 1996, 61, 5440 CrossRef CAS.
- CCDC 940260 contains the supplementary crystallographic data for this molecule.
-
(a) C. Zhu, A. Yoshimura, P. Solntsev, L. Ji, Y. Wei, V. N. Nemykin and V. V. Zhdankin, Chem. Commun., 2012, 48, 10108 RSC;
(b) C. Zhu, A. Yoshimura, L. Ji, Y. Wei, V. N. Nemykin and V. V. Zhdankin, Org. Lett., 2012, 14, 3170 CrossRef CAS PubMed.
- H. Saltzman and J. G. Sharefkin, Org. Synth., 1963, 43, 60 CrossRef CAS.
- In a recent study by Carroll and co-workers the authors concluded that varying the aryl group in alkynyl(aryl)iodonium salts had little effect on reactivity in a formal cycloaddition reaction, however only four aryl derivatives were assessed (not 2-iodoanisole) and a 12% variation in yields was observed: L. I. Dixon, M. A. Carroll, T. J. Gregson, G. J. Ellames, R. W. Harrington and W. Clegg, Eur. J. Org. Chem., 2013, 2334 CrossRef CAS.
Footnote |
† Electronic supplementary information (ESI) available: Characterization data and copies of 1H and 13C NMR spectra. CCDC 940260. For ESI and crystallographic data in CIF or other electronic format see DOI: 10.1039/c4ob00556b |
|
This journal is © The Royal Society of Chemistry 2014 |