Asymmetric organocatalytic synthesis of 4,6-bis(1H-indole-3-yl)-piperidine-2 carboxylates†
Received
30th January 2014
, Accepted 21st March 2014
First published on 21st March 2014
Abstract
We developed an asymmetric organocatalytic synthesis of 4,6-bis(1H-indole-3-yl)-piperidine-2-carboxylates using 10 mol% of a chiral phosphoric acid. The products, which are novel bisindole-piperidine-amino acid hybrids, can be obtained in one step from 3-vinyl indoles with imino esters in dichloromethane at room temperature after 1 h of reaction time. A variety of these compounds could be synthesized in up to 70% yield and 99% ee, and they were experimentally and computationally analyzed regarding their relative and absolute stereochemistry.
Introduction
Bisindole alkaloids can be widely found in nature, exhibiting various interesting biological activities which can be medicinally important. There are numerous compounds of marine origin bearing two isolated indoles on one heterocycle (Fig. 1), such as nortopsentins (1a–d)1,2 and their analogs which exhibit antitumor,2d,3–8 antiproliferative, antiplasmodial,9 and antifungal3 activities. The dragmacidins10 (e.g.2a–b) possess antitumor,11,12 phosphatase inhibitory,13 and antiviral14 activities and their derivatives hamacanthins15 (e.g.3) have antitumor,16 antifungal,17 and antibacterial16,18 properties.
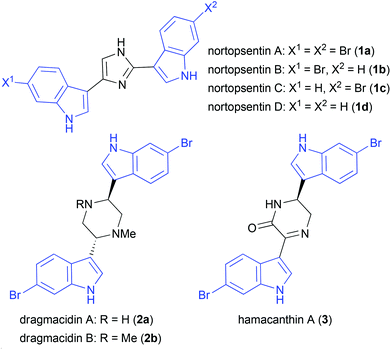 |
| Fig. 1 Structures of some indole alkaloids containing two isolated indole moieties. | |
In the late 80s, 3-vinylindole (4a) was found to be a versatile building block for the synthesis of heterocycles fused to an indole moiety. This is due to the fact that 3-vinylindole acts as a diene in the Diels–Alder reaction with various electron-deficient olefins and aza dienophiles, e.g. nitrosobenzene, DEAD, diethyl mesoxalate, benzoquinones, and maleimides.19–22 The reactivity of 3-vinylindoles can be utilized in the synthesis of carbazoles.19,20,23,24 Also reactions with singlet oxygen are reported.25 In the last few years, the first asymmetric organocatalytic Diels–Alder reactions of 3-vinylindoles were reported, including thiourea-catalyzed reactions with maleimides or quinones26 and indolones.27 Furthermore, the reactivity of 3-vinylindole can be that of a dienophile, furnishing Povarov-type products when reacted with electron-rich arylimines.28 In that work, it was proven that the intermediate could be trapped by an excess of 3-vinylindole (5 equiv.) leading to an interesting and complex bisindole consisting of two units of vinylindole and one unit of arylimine. However, the work of Ricci et al. mainly focused on the asymmetric Povarov reaction and they made no further investigations towards the synthesis and the relative/absolute configuration of the resulting bisindole-piperidine-hybrid.
Results and discussion
Reaction between 3-vinylindole (4a) and glyoxylate imine (8a)
We took on the interesting and dual reactivity of 3-vinylindoles and explored their behaviour towards various types of imines in order to create nitrogen heterocycles bearing new stereocenters. Imines 5–8a were prepared according to established procedures29–32 and treated with 3-vinylindole (4a)33 in toluene (Scheme 1). Only in the case of ethyl glyoxylate-derived imine 8a a reaction was observed. It turned out that 3-vinylindole did not act as a diene in this reaction, but the formed product arised from a multicomponent addition of two equivalents of 3-vinylindole (4a) and one equivalent of imino ester 8a. Interestingly, this reaction occurred at room temperature with a short reaction time (3.5 h) without any addition of the catalyst. The product is similar to that reported by Ricci et al. but has an ethyl ester moiety at the 2-position (therefore being an amino acid derivative) instead of a phenyl substituent and does not require a large excess of vinylindole.
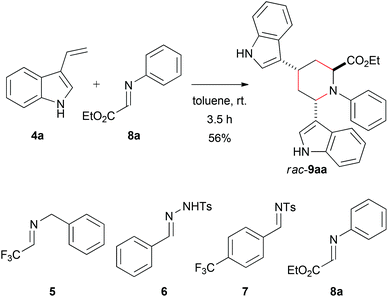 |
| Scheme 1 Racemic synthesis of bisindole rac-9aa with imino ester 8a (see ESI† for more information) and attempted reactions with other imines 5–7. | |
A plausible mechanism for this reaction is given in Scheme 2. After the first addition, the second molecule 3-vinylindole (4a) is able to attack the stabilized intermediate 10. This step proceeds considerably faster than the nucleophilic attack of the aromatic phenyl ring at position 4, since only traces of the corresponding Povarov reaction product were found. An intramolecular ring closure of 11 finally gives piperidine-2-carboxylic ester rac-9aa as the only possible regioisomer.
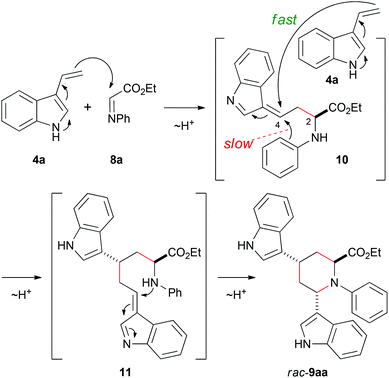 |
| Scheme 2 Proposed mechanism for the formation of bisindole rac-9aa. | |
For the reaction, a free NH group is necessary on the indole moiety, as in a reaction of N-methylated derivative (1-methyl-3-vinyl-1H-indole) with glyoxalate imine 8a, no product could be isolated. This supports the proposed mechanism for the racemic formation of 9aa in Scheme 2, which involves several proton transfers of the indole NH. Furthermore, following attempts to protect the indole-NH functionalities in the reaction product 9aa with trifluoroacetate or tosylate failed.
Asymmetric synthesis of bisindole 9aa and optimization
Next, the asymmetric reaction of 4a and 8a was investigated. It was envisioned that chiral thiourea catalysts, such as 12–14 (Fig. 2), would be able to interact strongly with imine 8avia H-bond catalysis.26,27,34–37 However, the results employing these catalysts were not satisfying, giving almost no enantioinduction with 7.5% ee at most and often strongly diminished yields (Table 1) compared to those without employing any catalyst. The enantioselectivity could not be improved when the temperature was lowered (entry 2). A considerable improvement of the yield was observed when utilizing catalyst 14 in CH2Cl2; however, in this case the product was nearly racemic (entry 4).
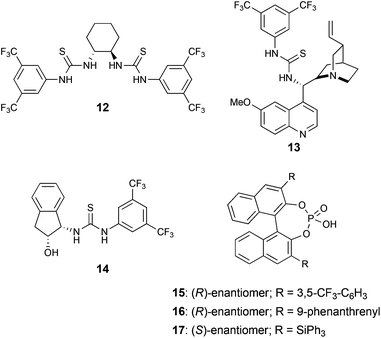 |
| Fig. 2 Screened thiourea and phosphoric acid catalysts. | |
Table 1 Screening of thiourea catalysts (upper part), phosphoric acid catalysts (middle part), and solvents (lower part)
Entry |
Cat. [mol%] |
Solvent |
Time |
Temp. |
Yielda |
eeb |
Unless stated otherwise, the catalyst and 3-vinylindole (4a, 0.1 mmol) were added to a solution of imine 8a (0.05 mmol) in an absolute solvent (1 mL). After consumption of the starting material the crude mixture was purified via preparative TLC or column chromatography (PE–EtOAc 3 : 1). Isolated yield. Determined by HPLC. Reaction was carried out with 0.1 mmol of 4a and 0.1 mmol of 8a. Imine 8a was prepared in situ from ethyl glyoxylate and aniline. Reaction was performed on a gram scale (14 mmol of 4a and 7 mmol of 8a). Reaction was carried out with 0.3 mmol of 4a and 0.1 mmol of 8a. |
1c |
12 [20] |
n-Hexane–toluene 2 : 1 |
4 h |
rt. |
22% |
3% |
2 |
12 [20] |
CH2Cl2 |
2 h |
−78 °C |
13% |
3% |
3c |
13 [20] |
n-Hexane–toluene 2 : 1 |
18 h |
rt. |
15% |
0% |
4 |
14 [20] |
CH2Cl2 |
16 h |
rt. |
52% |
2% |
5 |
14 [20] |
n-Hexane |
16 h |
rt. |
13% |
7.5% |
6 |
15 [10] |
Toluene |
2 h |
rt. |
46% |
69% |
7 |
15 [10] |
Toluene |
2.5 h |
0 °C |
51% |
56% |
8d |
15 [10] |
Toluene |
5 h |
0 °C |
54% |
54% |
9 |
16 [10] |
Toluene |
2 h |
rt. |
47% |
88% |
10 |
16 [10] |
Toluene |
5 h |
−78 °C |
Traces |
— |
11 |
16 [10] |
Toluene |
4 h |
−40 °C |
30% |
79% |
12c |
16 [10] |
Toluene |
1 h |
rt. |
60% |
92% |
13c |
16 [10] |
Toluene |
20 min |
50 °C |
69% |
91% |
14c |
17 [10] |
Toluene |
1 h |
rt. |
43% |
72% |
15c |
16 [10] |
THF |
4.5 h |
rt. |
70% |
91% |
16c |
16 [10] |
Et2O |
1.17 h |
rt. |
65% |
93% |
17c |
16 [10] |
CH2Cl2 |
1 h |
rt. |
64% |
94% |
18c |
16 [10] |
MeCN |
1.5 h |
rt. |
67% |
87% |
19e |
16 [10] |
CH2Cl2 |
1 h |
rt. |
57% |
94% |
20f |
16 [10] |
CH2Cl2 |
1 h |
rt. |
61% |
98% |
Chiral phosphoric acids38 represent another catalyst class widely used in organic chemistry, especially in the strongly related asymmetric (vinylogous) Mannich-type reactions and also employed by Ricci et al. in the aforementioned paper.28 Three phosphoric acid catalysts 15–17 (Fig. 2), which are commercially available, and different reaction conditions were screened towards their ability to promote an asymmetric reaction of 3-vinylindole (4a) and imine 8a to yield the substituted piperidine 9aa.
The following conclusions can be drawn considering the results in Table 1: (i) all of the screened chiral phosphoric acid catalysts give good enantiomeric excesses; (ii) good yields could be obtained while only using 1 equivalent of each reactant; (iii) in some cases, employing a catalyst leads to a considerable improvement of the chemical yield to 60% or more (entries 12 and 13) compared to the reaction without any catalyst; (iv) the best catalyst in terms of yield and ee (>90%) is catalyst 16 (entries 12 and 13). At 50 °C, the reaction is complete after only 20 min with a yield close to 70% and an ee of 91% (entry 13); (v) lowering the temperature did not improve the results, it even gave diminished yields and ees (entries 10 and 11); (vi) preparing imine 8ain situ from ethyl glyoxylate and aniline gives product 9aa, too, but with no significant change of yield and ee (entry 8), nevertheless this shows that even a four component reaction to form 9aa is feasible. Also, in the catalyzed reaction, the formation of Povarov products was completely suppressed.
Having found an efficient catalyst 16, several solvents were screened in order to improve the enantiomeric excess. The yields in any solvent remained quite stable (57–70%, entries 15–20). The enantiomeric excess was optimized in dichloromethane (up to 98%, entry 20). Acetonitrile caused the ee value to drop (entry 18).
Relative and absolute configuration of 9aa
The relative configuration was identified through NOE correlations (Fig. 3). In the proposed conformer structure, both indole-3-yl groups are standing in the equatorial position, thus minimizing steric interactions between one another, and the ethyl ester is standing axially, possibly to avoid the interaction with the N-phenyl group. In addition, crystals of 9aa could be obtained by recrystallization from iPrOH. The absolute configuration was unequivocally proven by X-ray crystallography using the effects of anomalous dispersion (Fig. 3). Thus a (2S,4S,6S) configuration could be confirmed (see also ESI† and CCDC 977608).
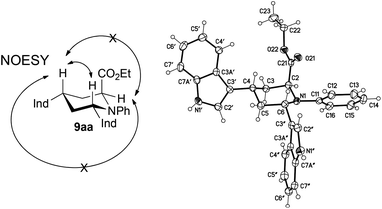 |
| Fig. 3 Structure of bisindole 9aa, left: experimentally determined NOE correlations; right: molecular structure of 9aa (displacement parameters are drawn at 50% probability level, CCDC 977608). | |
As our sample of 9aa had an enantiomeric purity of ee = 94%, it is generally possible that the single crystal used for the X-ray diffraction study consisted of the minor enantiomer. To exclude this eventuality, we measured the electronic circular dichroism (CD) spectrum, which is a property of the bulk compound, in methanol at 20 °C. Furthermore, using the TURBOMOLE program package,39 the spectrum was calculated with time-dependent density functional theory methods40 (along with the C-4 epimer of 9aa, which was clearly ruled out as a possible product, see also ESI†). Because of good agreement of the calculated spectrum of 9aa with the experimental spectrum, we can ensure the proposed (2S,4S,6S) configuration (Fig. 4).
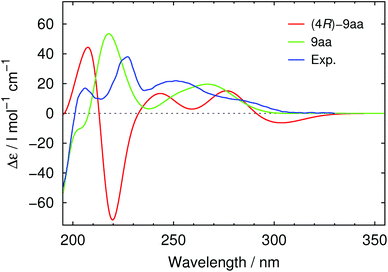 |
| Fig. 4 Experimental CD spectrum (blue curve, 94% ee) and calculated CD spectra of 9aa (green curve) and its C-4 epimer (red curve); conditions: c = 0.05 mg mL−1, MeOH, 20 °C. | |
Scope of the reaction
As the reaction conditions were optimized, the scope of the reaction was explored by using differently substituted 3-vinylindoles 4a–f and glyoxalate imines 8a–d. The reactions were carried out on a 0.1 mmol scale with catalyst 16 in dichloromethane. In all cases, high ees were achieved, while depending on the substitution pattern and varying stability of the different vinylindoles the yields were sometimes considerably lower than for the unsubstituted substrate 4a (Table 2). We believe that in the case of vinylindoles 4d and 4e (entries 7 and 8), the low yields are due to the fast decomposition of the starting material. Derivatives 4d and 4e must be used immediately after preparation and even when stored in the freezer at −20 °C, they decompose within a few days. Furthermore, we discovered that the reaction proceeded diastereoselectively, leading to one main product. Apart from the shown isomers, we observed, in most cases, traces of one or two other diastereomers which could not completely be separated from the main product. In the 1H NMR spectra, their signals can be seen and distinguished especially in the range of 4.5–5.5 ppm adjacent to the main product signals (see ESI†).
Table 2 Synthesis of bisindole derivatives 9xy
We also found that this kind of reactivity in a three-component reaction is only possible with 3-vinylindoles and not the isomeric 2-vinylindole (18), which certainly supports the necessity of a free electron pair on the nitrogen in conjugation to the vinyl group. Under the same reaction conditions with catalyst 16, we observed the typical reactivity of unsubstituted indoles with electrophiles, which is that of a spontaneous Friedel–Crafts addition,41,42 giving amino acid derivative 19 with only poor enantioselectivity (Scheme 3).
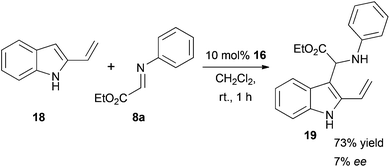 |
| Scheme 3 Synthesis of amino acid derivative 19. | |
Having proven the generality of the reaction by applying the conditions on various substrates, we explored whether an up-scaling of the reaction was possible. Upscaling (2 g scale) the reaction of bisindole 9aa still gave a comparable yield of 57% with an unchanged ee of 94%. Furthermore, we exemplarily showed that catalyst 16 was recyclable (see ESI† for more information).
Conclusions
In conclusion, we developed a powerful three-component, enantioselective, and organocatalytic synthesis of 4,6-bis(1H-indole-3-yl)piperidine 2-carboxylates 9xy utilizing easily accessible 3-vinylindoles and imino esters and a chiral phosphoric acid. The reaction proceeds fast (1 h) and diastereoselectively at room temperature, building three new bonds and three new stereogenic centers in one step, and furnishes the products in yields up to 70% and ees up to 99%. The reaction can be conducted on a gram scale and the catalyst is recyclable; furthermore, it is also possible to run it as a four-component reaction. The products are highly functionalized, as they are bisindole-piperidine-amino acid hybrids, and they resemble medicinally interesting natural products. Their activity towards various test organisms is currently under investigation.
Acknowledgements
We thank Dr. Jochen Bürck for CD spectral measurements, De Wang for helpful discussions, and Angela Wandler for experimental assistance. Financial support was realized by the Deutsche Telekom Stiftung, the Karlsruhe House of Young Scientists (KHYS) (fellowships to S. Zhong), the Deutsche Forschungsgemeinschaft (CFN, project C3.3), and the Sino-German collaboration program GZ417.
Notes and references
-
H. H. Sun, S. Sakemi, S. Gunasekera, Y. Kashman, M. Lui, N. Burres and P. McCarthy, U.S., US 4970226 A 19901113, 1990 Search PubMed.
- Syntheses and synthetic studies:
(a) I. Kaswasaki, M. Yamashita and S. Ohta, J. Chem. Soc., Chem. Commun., 1994, 2085 RSC;
(b) I. Kawaski, M. Yamashita and S. Ohta, Chem. Pharm. Bull., 1996, 44, 1831 CrossRef CAS;
(c) X.-H. Gu, X.-Z. Wan and B. Jiang, Bioorg. Med. Chem. Lett., 1999, 9, 569 CrossRef CAS;
(d) C. J. Moody and J. R. A. Roffey, ARKIVOC, 2000, 1, 393 CrossRef CAS;
(e) B. Jiang and X.-H. Gu, Bioorg. Med. Chem., 2000, 8, 363 CrossRef CAS;
(f) F. Y. Miyake, K. Yakushijin and D. A. Horne, Org. Lett., 2000, 2, 2121 CrossRef CAS PubMed;
(g) P. M. Fresneda, P. Molina and M. A. Sanz, Synlett, 2001, 218 CAS.
- S. Sakemi and H. H. Sun, J. Org. Chem., 1991, 56, 4304 CrossRef CAS.
- I. Mancini, G. Guella, C. Debitus, J. Waikedre and F. Pietra, Helv. Chim. Acta, 1996, 79, 2075 CrossRef CAS.
- X.-H. Gu, X.-Z. Wan and B. Jiang, Bioorg. Med. Chem. Lett., 1999, 9, 569 CrossRef CAS.
-
(a) P. Diana, A. Carbone, P. Barraja, A. Montalbano, A. Martorana, G. Dattolo, O. Gia, L. Dalla Via and G. Cirrincione, Bioorg. Med. Chem. Lett., 2007, 17, 2342 CrossRef CAS PubMed;
(b) P. Diana, A. Carbone, P. Barraja, G. Kelter, H.-H. Fiebig and G. Cirrincione, Bioorg. Med. Chem., 2010, 18, 4524 CrossRef CAS PubMed.
- A. Carbone, B. Parrino, P. Barraja, V. Spanò, G. Cirrincione, P. Diana, A. Maier, G. Kelter and H.-H. Fiebig, Mar. Drugs, 2013, 11, 643 CrossRef CAS PubMed.
- A. Carbone, M. Pennati, B. Parrino, A. Lopergolo, P. Barraja, A. Montalbano, V. Spanò, S. Sbarra, V. Doldi, M. De Cesare, G. Cirrincione, P. Diana and N. Zaffaroni, J. Med. Chem., 2013, 56, 7060 CrossRef CAS PubMed.
- S. Alvarado, B. F. Roberts, A. E. Wright and D. Chakrabarti, Antimicrob. Agents Chemother., 2013, 57, 2362 CrossRef CAS PubMed.
- Syntheses and synthetic studies:
(a) B. Jiang, J. M. Smallheer, C. Amaral-Ly and M. A. Wuonola, J. Org. Chem., 1994, 59, 6823 CrossRef CAS;
(b) C. R. Whitlock and M. P. Cava, Tetrahedron Lett., 1994, 35, 371 CrossRef CAS;
(c) T. Kawasaki, H. Enoki, K. Matsumura, M. Ohyama, M. Inagawa and M. Sakamoto, Org. Lett., 2000, 2, 3027 CrossRef CAS PubMed;
(d) B. Jiang and X.-H. Gu, Heterocycles, 2000, 53, 1559 CrossRef CAS;
(e) F. Y. Miyake, K. Yakushijin and D. A. Horne, Org. Lett., 2000, 2, 3185 CrossRef CAS PubMed;
(f) N. K. Garg, R. Sarpong and B. M. Stoltz, J. Am. Chem. Soc., 2002, 124, 13179 CrossRef CAS PubMed;
(g) T. Kawasaki, K. Ohno, H. Enoki, Y. Umemoto and M. Sakamoto, Tetrahedron Lett., 2002, 43, 4245 CrossRef CAS;
(h) C.-G. Yang, G. Liu and B. Jiang, J. Org. Chem., 2002, 67, 9392 CrossRef CAS PubMed;
(i) C.-G. Yang, J. Wang and B. Jiang, Tetrahedron Lett., 2002, 43, 1063 CrossRef CAS;
(j) C.-G. Yang, J. Wang, X.-X. Tang and B. Jiang, Tetrahedron: Asymmetry, 2002, 13, 383 CrossRef CAS;
(k) N. K. Garg, D. D. Caspi and B. M. Stoltz, J. Am. Chem. Soc., 2004, 126, 9552 CrossRef CAS PubMed;
(l) N. K. Garg and B. M. Stoltz, Tetrahedron Lett., 2005, 46, 2423 CrossRef CAS PubMed;
(m) K. S. Feldman and P. Ngernmeesri, Org. Lett., 2005, 7, 5449 CrossRef CAS PubMed;
(n) N. K. Garg, D. D. Caspi and B. M. Stoltz, J. Am. Chem. Soc., 2005, 127, 5970 CrossRef CAS PubMed;
(o) N. K. Garg, D. D. Caspi and B. M. Stoltz, Synlett, 2006, 3081 CAS;
(p) F. Tonsiengsom, F. Y. Miyake, K. Yakushijin and D. A. Horne, Synthesis, 2006, 49 CAS;
(q) M. Anstiss and A. Nelson, Org. Biomol. Chem., 2006, 4, 4135 RSC;
(r) N. K. Garg and B. M. Stoltz, Chem. Commun., 2006, 3769 RSC;
(s) R. J. Huntley and R. L. Funk, Org. Lett., 2006, 8, 4775 CrossRef CAS PubMed;
(t) M. Ikoma, M. Oikawa and M. Sasaki, Tetrahedron Lett., 2008, 49, 7197 CrossRef CAS PubMed;
(u) M. Seto, J. L. Roizen and B. M. Stoltz, Angew. Chem., Int. Ed., 2008, 47, 6873 CrossRef CAS PubMed;
(v) K. S. Feldman and P. Ngernmeesri, Org. Lett., 2011, 13, 5704 CrossRef CAS PubMed;
(w) D. Mandal, A. D. Yamaguchi, J. Yamaguchi and K. Itami, J. Am. Chem. Soc., 2011, 133, 19660 CrossRef CAS PubMed;
(x) K. S. Feldman and P. Ngernmeesri, Synlett, 2012, 1882 CrossRef CAS PubMed.
- S. Kohmoto, Y. Kashman, O. J. McConnell, K. L. Rinehart Jr., A. Wright and F. Koehn, J. Org. Chem., 1988, 53, 3116 CrossRef CAS.
- A. E. Wright, S. A. Pomponi, S. S. Cross and P. McCarthy, J. Org. Chem., 1992, 57, 4772 CrossRef CAS.
- R. J. Capon, F. Rooney, L. M. Murray, E. Collins, A. T. R. Sim, J. A. P. Rostas, M. S. Butler and A. R. Carroll, J. Nat. Prod., 1998, 61, 660 CrossRef CAS PubMed.
- A. Cutignano, G. Bifulco, I. Bruno, A. Casapullo, L. Gomez-Paloma and R. Riccio, Tetrahedron, 2000, 56, 3743 CrossRef CAS.
- Syntheses and synthetic studies:
(a) T. Kawasaki, T. Kouko, H. Totsuka and K. Hiramatsu, Tetrahedron Lett., 2003, 44, 8849 CrossRef CAS PubMed;
(b) T. Kouko, K. Matsumura and T. Kawasaki, Tetrahedron, 2005, 61, 2309 CrossRef CAS PubMed.
- B. Bao, Q. Sun, X. Yao, J. Hong, C. Lee, Y. H. Cho and J. H. Jung, J. Nat. Prod., 2007, 70, 2 CrossRef CAS PubMed.
- S. P. Gunasekera, P. J. McCarthy and M. Kelly-Borges, J. Nat. Prod., 1994, 57, 1437 CrossRef CAS.
- K.-B. Oh, W. Mar, S. Kim, J.-Y. Kim, T.-H. Lee, J.-G. Kim, D. Shin, C. J. Sim and J. Shin, Biol. Pharm. Bull., 2006, 29, 570 CAS.
- E. M. Beccalli, A. Marchesini and T. Pilati, Tetrahedron, 1996, 52, 3029 CrossRef CAS.
-
(a) U. Pindur and L. Pfeuffer, Chimia, 1986, 40, 124 Search PubMed;
(b) U. Pindur and L. Pfeuffer, Monatsh. Chem., 1986, 110, 95 CAS;
(c) U. Pindur, Heterocycles, 1988, 27, 1253 CrossRef CAS PubMed;
(d) U. Pindur and L. Pfeuffer, Monatsh. Chem., 1989, 120, 27 CrossRef;
(e) U. Pindur and M. H. Kim, Chem. Ztg., 1991, 115, 237 CAS.
- B. Saroja and P. C. Srinivasan, Synthesis, 1986, 748 CrossRef CAS PubMed.
- J. D. Lambert and Q. N. Porter, Aust. J. Chem., 1981, 34, 1483 CrossRef CAS.
- W. E. Noland and S. R. Wand, J. Org. Chem., 1979, 44, 4402 CrossRef CAS.
- H. Hagiwara, T. Choshi, H. Fujimoto, E. Sugino and S. Hibino, Tetrahedron, 2000, 56, 5807 CrossRef CAS.
- X. Zhang, S. I. Khan and C. S. Foote, J. Org. Chem., 1993, 58, 7839 CrossRef CAS.
-
(a) C. Gioia, A. Hauville, L. Bernardi, F. Fini and A. Ricci, Angew. Chem., Int. Ed., 2008, 47, 9236 CrossRef CAS PubMed;
(b) C. Gioia, L. Bernardi and A. Ricci, Synthesis, 2010, 161 CAS.
- B. Tan, G. Hernández-Torres and C. F. Barbas III, J. Am. Chem. Soc., 2011, 133, 12354 CrossRef CAS PubMed.
- G. Bergonzini, L. Gramigna, A. Mazzanti, M. Fochi, L. Bernardi and A. Ricci, Chem. Commun., 2010, 46, 327 RSC.
- Imine 8a was synthesized similar to: E. Borrione, M. Prato, G. Scorrano, M. Stivanello and V. Lucchini, J. Heterocycl. Chem., 1988, 25, 1831 CrossRef CAS.
- Synthesis of 5: Q.-Y. Zhao, Z.-L. Yuan and M. Shi, Adv. Synth. Catal., 2011, 353, 637 CrossRef CAS.
- Synthesis of 6: V. K. Aggarwal, E. Alonso, I. Bae, G. Hynd, K. M. Lydon, M. J. Palmer, M. Patel, M. Porcelloni, J. Richardson, R. A. Stenson, J. R. Studley, J.-L. Vasse and C. L. Winn, J. Am. Chem. Soc., 2003, 125, 10926 CrossRef CAS PubMed.
- Synthesis of 7: Z. Li, X. Ren, Y. Shi and P. Ouyang, Synth. Commun., 2007, 37, 713 CrossRef CAS.
- Synthesis of 4a: this compound was synthesized with a slightly modified procedure according to ref. 26a, see also ESI.†.
-
(a) Y. Sohtome, A. Tanatani, Y. Hashimoto and K. Nagasawa, Tetrahedron Lett., 2004, 45, 5589 CrossRef CAS PubMed;
(b) N. J. A. Martin, L. Ozores and B. List, J. Am. Chem. Soc., 2007, 129, 8976 CrossRef CAS PubMed;
(c) Y. Zhang, Y.-K. Liu, T.-R. Kang, Z.-K. Hu and Y.-C. Chen, J. Am. Chem. Soc., 2008, 130, 2456 CrossRef CAS PubMed;
(d) C. Rampalakos and W. D. Wulff, Adv. Synth. Catal., 2008, 350, 1785 CrossRef CAS PubMed;
(e) Y. Sohtome, N. Takemura, R. Takagi, Y. Hashimoto and K. Nagasawa, Tetrahedron, 2008, 64, 9423 CrossRef CAS PubMed;
(f) X. Li, H. Deng, S. Luo and J.-P. Cheng, Eur. J. Org. Chem., 2008, 4350 CrossRef CAS;
(g) D. Enders, K. Gottfried and G. Raabe, Adv. Synth. Catal., 2010, 352, 3147 CrossRef CAS.
-
(a) J. Song, Y. Wang and L. Deng, J. Am. Chem. Soc., 2006, 128, 6048 CrossRef CAS PubMed;
(b) J. Song, H.-W. Shih and L. Deng, Org. Lett., 2007, 9, 603 CrossRef CAS PubMed;
(c) L. Cheng, L. Liu, H. Jia, D. Wang and Y.-J. Chen, J. Org. Chem., 2009, 74, 4650 CrossRef CAS PubMed;
(d) B.-J. Li, L. Jiang, M. Liu, Y.-C. Chen, L.-S. Ding and Y. Wu, Synlett, 2005, 603 CAS;
(e) B. Vakulya, S. Varga, A. Csampai and T. Soos, Org. Lett., 2005, 7, 1967 CrossRef CAS PubMed.
- R. P. Herrera, V. Sgarzani, L. Bernardi and A. Ricci, Angew. Chem., Int. Ed., 2005, 44, 6576 CrossRef CAS PubMed.
- Some original work on thiourea catalysis:
(a) M. S. Sigman, P. Vachal and E. N. Jacobsen, Angew. Chem., Int. Ed., 2000, 39, 1279 CrossRef CAS;
(b) P. R. Schreiner and A. Wittkopp, Org. Lett., 2002, 4, 217 CrossRef CAS PubMed.
- Reviews:
(a) M. Terada, Chem. Commun., 2008, 4097 RSC;
(b) M. Terada, Synthesis, 2010, 1929 CrossRef CAS PubMed;
(c) S. J. Connon, Angew. Chem., Int. Ed., 2006, 45, 3909 CrossRef CAS PubMed;
(d) D. Kampen, C. M. Reisinger and B. List, Top. Curr. Chem., 2010, 291, 395 CrossRef CAS.
- TURBOMOLE, Version 6.4; University of Karlsruhe and Forschungszentrum Karlsruhe GmbH, 1989–2007, Turbomole GmbH since 2007; http://www.turbomole.com.
-
(a) F. Furche, R. Ahlrichs, C. Wachsmann, E. Weber, A. Sobanski, F. Vögtle and S. Grimme, J. Am. Chem. Soc., 2000, 122, 1717 CrossRef CAS;
(b) S. Grimme, F. Furche and R. Ahlrichs, Chem. Phys. Lett., 2002, 361, 321–328 CrossRef CAS.
- B. Jiang and Z.-G. Huang, Synthesis, 2005, 2198 CrossRef CAS PubMed.
- Y.-Q. Wang, J. Song, R. Hong, H. Li and L. Deng, J. Am. Chem. Soc., 2006, 128, 8156 CrossRef CAS PubMed.
Footnote |
† Electronic supplementary information (ESI) available: Experimental details, analytic data (including NMR spectra, HPLC traces), details of CD spectral calculations, and crystallographic data for 9aa. CCDC 977608. For ESI and crystallographic data in CIF or other electronic format see DOI: 10.1039/c4ob00234b |
|
This journal is © The Royal Society of Chemistry 2014 |
Click here to see how this site uses Cookies. View our privacy policy here.