DOI:
10.1039/C3GC41548A
(Paper)
Green Chem., 2014,
16, 351-356
AIBN-initiated metal free amidation of aldehydes using N-chloroamines†
Received
31st July 2013
, Accepted 25th October 2013
First published on 25th October 2013
Abstract
An efficient and environmentally benign amidation of aldehydes with N-chloroamines has been developed using AIBN as an initiator. This methodology offers a metal free and base free approach and is endowed with mild reaction conditions, high yields, and good functional group tolerance.
Amides constitute an extremely important class of compounds having a variety of biological activities and are prevalent in a number of natural products, pharmaceuticals and polymers (Fig. 1).1 In 2005 the American Chemical Society, the Green Chemistry Institute and several leading global pharmaceutical corporations developed the ACS GCI Pharmaceutical Roundtable, and the meeting voted ‘amide formation avoiding poor atom economy reagents’ as the top challenge for organic chemistry.2 Traditional methods for the synthesis of amides include the condensation of carboxylic acid derivatives with amines. But they all invariably suffer from severe drawbacks such as lability of activated carboxylic acid derivatives and the use of stoichiometric excess of coupling reagents. To address these concerns, a great deal of effort has been made towards the development of alternative methods for the synthesis of amides, which include the Beckmann rearrangement,3 aminocarbonylation of aryl halides4 and alkynes,5 cross coupling of formamides with alkyl/aryl halides,6 umpolung reaction of amines with α-halo nitro alkanes,7 and transamidation.8
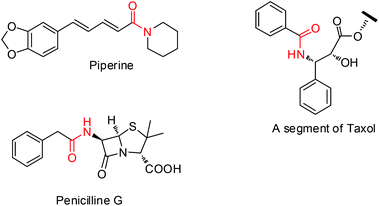 |
| Fig. 1 Some medicinally important molecules containing the amide group. | |
A fascinating development in this direction is the oxidative amidation of aldehydes with amines. In the past few years, both metal catalysed and metal free syntheses of amides by the oxidative coupling of aldehydes or alcohols with amines have been reported.9 Although these methods possess their own merits, they make use of expensive transition metals and stoichiometric excess of reagents and have limited substrate scope. N-Chloroamines are useful aminating agents that undergo substitution reactions with organometallics,10 heteroaromatics,11 and enolates.12 While the C–N bond formation for the synthesis of amides using N-chloroamines is less explored,13 metal free C–N bond formation employing N-chloroamines has not yet been reported. In view of the above and as a part of our interest in exploring newer protocols for amide formation14 and others,15 we report herein for the first time an AIBN (a radical initiator) initiated convenient and metal free amidation of aldehydes with N-chloroamines under mild conditions (Scheme 1).
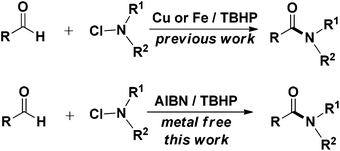 |
| Scheme 1 Amidation of aldehydes with N-chloroamines. | |
Based on insights from the literature on the mechanism of amidation of aldehydes, it was comprehended that the reaction involved the formation of a carbinolamine intermediate,9h,16 which subsequently underwent oxidation to give amide. A nitrogen–halide bond (N–X) enjoys high reactivity and is known to undergo homolytic or heterolytic bond-cleavage readily.17 As a result, it was envisioned that the use of N-chloroamines as a source of amine partners could generate radicals to be successively involved in the reaction to furnish metal free amidation. Azobisisobutylonitrile [abbreviated AIBN, 2,2′-Azobis(2-methylpropionitrile)] is an inexpensive and commonly used radical initiator in organic synthesis, which sets off intramolecular radical arylations,18 and is employed in the synthesis of biaryls.19 Therefore it was thought worthwhile to employ AIBN as an initiator for the envisaged amidation reaction, and to our utmost delight the preliminary reaction between p-tolualdehyde and N-chlorobenzylamine worked well. An experiment involving the parent amine and N-chloroamine under the same conditions, however, did not afford the desired amide product (Scheme 2), which is evidently suggestive of the involvement of nitrogen centred radicals.
 |
| Scheme 2 Control experiment. | |
In order to optimize the reaction conditions, a model reaction using N-chlorobenzylamine (prepared in situ from benzylamine and N-chlorosuccinimide) and p-tolualdehyde was screened in detail and the outcomes are given in Table 1. To begin with, the reaction was undertaken in the presence of AIBN (0.2 equiv.) in acetonitrile at 80 °C, which gave rise to 55% product yield in 48 h (Table 1, entry 3). A further increase in reaction time and temperature did not improve the yield (entries 4 & 5). No product formation was observed when the reaction was conducted at room temperature. Increasing the stoichiometry of AIBN up to 1 equiv. had shown no useful improvement (entry 6). Since all attempts to improve yield failed, it was decided to resort to the use of oxidants/additives in combination with AIBN. Different trials using diverse additives and oxidants were made (Table 1, entries 7–17). Out of all the trials to improve the yield, TBHP was found to be the best. The use of oxidants viz. K2S2O8 and CAN as well as the application of a nitrogen atmosphere was ineffective. Copper salts, when employed as additives, diminished the yield rather than improving it (entries 14, 15 & 17). AIBN without TBHP gave rise to 57% yield in 72 h (entry 4), whereas a considerably low product yield (15%) is obtained in the presence of TBHP without the use of AIBN (entry 13). Employing TBHP in combination with AIBN showed a profound effect on the product yield and required a considerably reduced reaction time (entry 10), thereby suggestive of the role of TBHP as a supporting oxidant. As regards the stoichiometry of TBHP, 4 equiv. was concluded to be optimal; a decrease in the stoichiometric amount, however, resulted in diminished product yield. With respect to the molar proportion of the reactants, a 2
:
1 molar ratio of N-chloroamine and aldehyde was found to be adequate, as a further increase in the proportion showed no improvement.
Table 1 Optimisation of reaction conditions with AIBN as an initiatora

|
Entry |
Initiator |
Additive/oxidant |
Time (h) |
Temp. (°C) |
Yieldb (%) |
Reaction conditions: p-tolualdehyde (1 mmol), N-chlorobenzylamine (2 mmol), the oxidant/additive (1 equiv.) AIBN (20 mol%) in 6 mL acetonitrile, unless otherwise stated.
Isolated yield,
AIBN (1 equiv.),
TBHP (4 equiv.). r.t. = room temp.
|
1 |
— |
— |
24 |
80 |
0 |
2 |
AIBN |
— |
36 |
80 |
45 |
3 |
AIBN |
— |
48 |
80 |
55 |
4 |
AIBN |
— |
72 |
80 |
57 |
5 |
AIBN |
— |
48 |
100 |
55 |
6 |
AIBNc |
— |
48 |
80 |
56 |
7 |
AIBN |
N2 |
48 |
80 |
57 |
8 |
AIBN |
K2S2O8 |
24 |
80 |
0 |
9 |
AIBN |
TBHP |
24 |
80 |
70 |
10
|
AIBN
|
TBHP
d
|
24
|
80
|
85
|
11 |
AIBN |
TBHP |
36 |
80 |
85 |
12 |
AIBN |
TBHP |
24 |
r.t. |
0 |
13 |
— |
TBHP |
48 |
80 |
15 |
14 |
AIBN |
Cu(OAc)2·H2O |
24 |
80 |
0 |
15 |
AIBN |
CuI |
24 |
80 |
0 |
16 |
AIBN |
CAN |
24 |
80 |
0 |
17 |
AIBN |
Cu(OAc)2 |
24 |
80 |
15 |
Encouraged by these results and having high yielding conditions in our hands, the applicability of this optimization was further tested using different aldehydes and N-chloroamines. The outcome is summarised in Table 2. Aromatic aldehydes containing both the electron-donating groups such as methyl and methoxy, and electron-withdrawing groups such as chloro, bromo and nitro, were well tolerated during the course of the reaction providing the desired amides 3a–k, 3m–v, 3y, 3z and 3ab in moderate to excellent yields. Thiophene-2-carboxaldehyde also reacted well to give the desired amide 3l, although furfuraldehyde did not react at all. The reaction of heptanaldehyde with N-chlorobenzylamine and N-chloromorpholine was also successful in providing the corresponding amides 3w and 3x. The reaction was also examined with diverse primary and secondary N-chloroamines. N-Chloroamines derived from benzylamine, cyclohexylamine, hexylamine, butylamine, morpholine, piperidine, and N-methylbenzylamine invariably responded effectively in the reaction, affording good yields. N-Chloro-1-phenylethanamine gave better yield as compared to N-chloro-2-phenylethanamine. The optical purity of the product 3k has been determined and is found to be 81.6%. To study the role of steric effects in the reaction, ortho substituted aromatic aldehydes such as 2-bromobenzaldehyde and salicylaldehyde were employed. 2-Bromobenzaldehyde worked well but salicylaldehyde did not work at all. To gain further insights into the reaction, 4-hydroxy-3-methoxybenzaldehyde was employed, but the reaction did not occur. It reveals the presence of the hydroxyl group to be crucial to crash the reaction both in the case of vanillin and salicylaldehyde. When 2,4-dichlorobenzaldehyde was made to react with N-chlorobenzylamine the reaction did not happen, which may be attributed to the instability of the resulting acyl radical coming from this aldehyde. During the course of the reaction using heptanal, the mixture became sticky and thus decreased the yield.
Table 2 AIBN initiated amidation of various aldehydes with N-chloroaminesa

|
Reaction conditions: aldehydes (0.5 mmol), N-chloroamines (1 mmol), AIBN (20 mol%), aq. TBHP (4 equiv.) for 24 h at 80 °C. Isolated yields reported.
|
|
Based on the above results and the literature, a plausible mechanism is outlined to explain the desired transformation (Scheme 3). AIBN is decomposed into a cyanoisopropyl radical (Cp*), which abstracts a hydrogen atom from the aldehyde to generate an acyl radical. N-Chloroamine readily undergoes cleavage to give a nitrogen centred radical,17a which eventually reacts with an acyl radical to give the amide. To look into the mechanistic insights, radical trapping experiments using ascorbic acid,20 BHT,21 and TEMPO were undertaken; however, the reaction progress was completely inhibited. No further evidence could be obtained using TEMPO, as the formation of an ester or any other by-product was not observed and the reactions were inhibited. TBHP is assumed to promote the formation of acyl radicals,13 thereby facilitating the reaction.
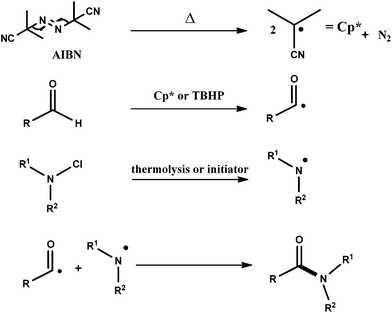 |
| Scheme 3 Proposed mechanism. | |
Conclusions
In conclusion, we have developed a new, efficient and green methodology for AIBN initiated C–N bond formation for the synthesis of amides using N-chloroamines as aminating agents under metal- and base-free conditions.
Experimental section
General reaction procedure for metal free amidation of aldehydes with N-chloroamines
N-Chlorosuccinimide (1.2 mmol) was added to a solution of amine (1 mmol) in acetonitrile (3 mL) and stirred at room temperature for 3 hours. To this solution were then added the aldehyde (0.5 mmol), AIBN (20 mol%) and TBHP (70% aq., 4 equiv.). The reaction mixture was heated at 80 °C in an oil bath while stirring for 24 h. After the reaction was complete (TLC), the mixture was extracted with ethyl acetate (3 × 10 mL). The combined organic phase was dried over anhydrous Na2SO4, filtered and evaporated under reduced pressure. The crude product thus obtained was purified by silica gel column chromatography using n-hexane–ethyl acetate as an eluent.
N-Benzylbenzamide (3a)22.
White solid; 1H NMR (CDCl3, 300 MHz): δ 7.79 (d, J = 7.2 Hz, 2H), 7.50–7.32 (m, 8H), 6.61 (bs, 1H), 4.62 (d, J = 5.7 Hz, 2H); 13C NMR (CDCl3, 75 MHz): δ 167.3, 138.2, 134.3, 131.5, 128.7, 128.5, 127.8, 127.5, 126.9, 44.0.
N-Benzyl-4-methylbenzamide (3b)28.
Yellow solid; 1H NMR (CDCl3, 300 MHz): δ 7.78–7.65 (m, 2H), 7.36–7.12 (m, 7H), 6.93 (bs, 1H), 4.54 (d, J = 4.8 Hz, 2H), 2.34 (s, 3H); 13C NMR (CDCl3, 75 MHz): δ 167.4, 141.7, 138.3, 131.3, 129.0, 128.5, 127.6, 127.2, 126.9, 43.8, 21.2.
N-Benzyl-4-methoxybenzamide (3c)26.
White solid; 1H NMR (CDCl3, 300 MHz): δ 7.77 (d, J = 8.1 Hz, 2H), 7.34 (bs, 5H), 6.92 (d, J = 8.4 Hz, 2H), 6.36 (bs, 1H), 4.64 (d, J = 5.4 Hz, 2H), 3.84 (s, 3H); 13C NMR (CDCl3, 75 MHz): δ 166.8, 162.2, 138.4, 132.3, 128.7, 127.9, 127.5, 126.6, 113.7, 55.3, 44.1.
N-Benzyl-4-chlorobenzamide (3d)28.
Yellow solid; 1H NMR (CDCl3, 300 MHz): δ 7.69–7.60 (m, 2H), 7.33–7.28 (m, 7H), 6.48 (s, 1H), 4.52 (d, J = 4.8, 2H); 13C NMR (CDCl3, 75 MHz): δ 166.4, 138.0, 137.8, 131.5, 128.8, 128.4, 127.8, 127.6, 126.9, 44.3.
N-Benzyl-4-nitrobenzamide (3e)23.
Yellow solid; 1H NMR (CDCl3, 300 MHz): δ 8.27 (m, 2H), 7.95 (m, 2H), 7.35–7.26 (m, 5H), 6.64 (bs, 1H), 4.66 (d, J = 5.4, 2H); 13C NMR (CDCl3, 75 MHz): δ 165.3, 149.6, 139.9, 137.4, 128.9, 128.6, 128.2, 127.9, 123.8, 44.4.
N-Benzyl-3-chlorobenzamide (3f)27.
Yellow solid; 1H NMR (CDCl3, 300 MHz): δ 7.79–7.76 (m, 1H), 7.66–7.63 (m, 1H), 7.47–7.34 (m, 7H), 6.44 (bs, 1H), 4.63 (d, J = 5.4, 2H); 13C NMR (CDCl3, 75 MHz): δ 166.3, 138.0, 137.7, 132.7, 131.5, 131.0, 128.7, 128.4, 127.8, 127.6, 126.9, 44.1.
4-Methoxy-N-(1-phenylethyl)benzamide (3g)34.
White solid; 1H NMR (CDCl3, 300 MHz): δ 7.75–7.72 (m, 2H), 7.37–7.25 (m, 5 Hz), 6.91 (d, J = 8.7 Hz, 2H) 5.34–5.29 (m, 1H), 3.83 (s, 3H), 1.60 (d, J = 6.9 Hz, 3H); 13C NMR (CDCl3, 75 MHz): δ 166.1, 162.1, 143.3, 128.7, 127.3, 126.8, 126.2, 113.7, 55.3, 49.2, 21.8.
(4-Chlorophenyl)(piperidin-1-yl)methanone (3h)25.
Yellow solid; 1H NMR (CDCl3, 300 MHz): δ 7.39–7.31 (m, 4H), 3.68 (bs, 2H), 3.33 (bs, 2H), 1.76–1.44 (m, 6H); 13C NMR (CDCl3, 75 MHz): δ 169.2, 135.4, 134.8, 128.6, 128.4, 48.8, 43.3, 25.8, 25.6, 24.5.
N-Cyclohexylbenzamide (3i)33.
Yellow solid; 1H NMR (CDCl3, 300 MHz): δ 7.76 (d, J = 6.8 Hz, 2H), 7.47–7.40 (m, 3H), 6.05 (bs, 1H), 3.99 (bs, 1H), 2.04–2.01 (m, 2H), 1.77–1.67 (m, 3H), 1.44–1.22 (m, 5H); 13C NMR (CDCl3, 75 MHz): δ 166.6, 135.1, 131.2, 128.4, 126.8, 48.6, 33.2, 25.5, 24.9.
N-Butylbenzamide (3j)26.
Yellow liquid; 1H NMR (CDCl3, 300 MHz): δ 7.77–7.74 (m, 2H), 7.47–7.38 (m, 3H), 6.32 (bs, 1H), 3.45 (dd, J = 6.6, 13.2 Hz, 2H), 1.62–1.54 (m, 2H), 1.44–1.34 (m, 2H), 0.95 (t, J = 7.2 Hz, 3H); 13C NMR (CDCl3, 75 MHz): δ 167.5, 134.8, 131.2, 128.4, 126.8, 39.8, 31.7, 20.1, 13.7.
N-(1-Phenylethyl)benzamide (3k)24.
White solid; 1H NMR (CDCl3, 300 MHz): δ 7.77–7.75 (m, 2H), 7.47–7.29 (m, 8H), 6.41 (bs, 1H), 5.35–5.30 (m, 1H), 1.60 (d, J = 6.6 Hz, 3H); 13C NMR (CDCl3, 75 MHz): δ 166.5, 143.1, 134.6, 131.4, 128.7, 128.5, 127.4, 126.9, 126.2, 49.1, 21.6.
N-Benzylthiophene-2-carboxamide (3l)27.
Yellow solid; 1H NMR (CDCl3, 300 MHz): δ 7.52–7.25 (m, 7H), 7.06–7.03 (m, 1H), 6.43 (bs, 1H), 4.60 (d, J = 5.4, 2H); 13C NMR (CDCl3, 75 MHz): δ 161.8, 138.7, 138.0, 131.5, 130.0, 128.7, 128.5, 128.1, 127.9, 127.6, 126.9, 43.9.
N-Phenethylbenzamide (3m)31.
Yellow solid; 1H NMR (CDCl3, 300 MHz): δ 7.71–7.69 (m, 2H), 7.41–7.15 (m, 8H), 6.74 (bs, 1H), 3.68–3.61 (m, 2H), 2.88(t, J = 7.0 Hz, 2H); 13C NMR (CDCl3, 75 MHz): δ 167.6, 138.8, 134.4, 131.2, 128.6, 128.4, 128.3, 126.7, 126.3, 41.1, 35.5.
N-Hexylbenzamide (3n)25.
White solid; 1H NMR (CDCl3, 300 MHz): δ 7.80–7.75 (m, 2H), 7.48–7.35 (m, 3H), 6.59 (bs, 1H), 3.44–3.37 (m, 2H), 1.61–1.56 (m, 2H), 1.33 (m, 6H), 0.93–0.86 (m, 3H); 13C NMR (CDCl3, 75 MHz): δ 167.5, 134.8, 131.1, 128.3, 126.8, 40.0, 31.4, 29.5, 26.6, 22.4, 13.9.
N-Hexyl-4-methylbenzamide (3o)25.
White solid; 1H NMR (CDCl3, 300 MHz): δ 7.67 (d, J = 7.8 Hz, 2H), 7.22 (d, J = 7.8 Hz, 2H), 6.18 (bs, 1H), 3.51–3.40 (m, 2H), 2.38 (s, 3H), 1.60–1.57 (m, 2H), 1.32–1.18 (m, 6H), 0.91–0.87 (m, 3H); 13C NMR (CDCl3, 75 MHz): δ 167.5, 141.6, 132.0, 129.1, 126.8, 40.0, 31.5, 29.6, 26.6, 22.5, 21.4, 14.0.
Morpholino(phenyl)methanone (3p)29.
Yellow liquid; 1H NMR (CDCl3, 300 MHz): δ 7.58–7.36 (m, 5H), 3.7 (bs, 8H); 13C NMR (CDCl3, 75 MHz): δ 170.3, 135.2, 129.7, 128.4, 126.9, 66.7, 48.0, 42.5.
Morpholino(p-tolyl)methanone (3r)29.
Yellow liquid; 1H NMR (CDCl3, 300 MHz): δ 7.31 (d, J = 7.8 Hz, 2H), 7.21 (d, J = 7.8 Hz, 2H), 3.68 (bs, 8H), 2.37 (s, 3H); 13C NMR (CDCl3, 75 MHz): δ 170.4, 139.9, 132.0, 128.9, 127.0, 66.6, 48.1, 42.4, 21.2.
4-Chloro-N-hexylbenzamide (3s)25.
White solid; 1H NMR (CDCl3, 300 MHz): δ 7.74–7.68 (m, 2H), 7.43–7.37 (m, 2H), 6.18 (bs, 1H), 3.46–3.39 (m, 2H), 1.71–1.55 (m, 2H), 1.33–1.25 (m, 6H), 0.89–0.87 (m, 3H); 13C NMR (CDCl3, 75 MHz): δ 166.4, 137.5, 133.2, 128.7, 128.3, 40.2, 31.5, 29.6, 26.6, 22.5, 14.0.
4-Chloro-N-phenethylbenzamide (3t)30.
Yellow solid; 1H NMR (CDCl3, 300 MHz): δ 7.69–7.60 (m, 2H), 7.47–7.21 (7H, m), 6.12 (bs, 1H), 3.74–3.67 (m, 2H), 2.93 (t, J = 6.9 Hz, 2H); 13C NMR (CDCl3, 75 MHz): δ 166.1, 138.7, 134.7, 131.4, 129.8, 128.7, 127.2, 126.6, 124.8, 41.2, 35.6.
4-Nitro-N-phenethylbenzamide (3u)31.
Yellow solid; 1H NMR (CDCl3, 300 MHz): δ 8.24 (d, J = 8.4 Hz, 2H), 7.84 (d, J = 8.4 Hz, 2H), 7.35–7.21 (m, 5H), 6.40 (bs, 1H) 3.76–3.70 (m, 2H), 2.95 (t, J = 6.9 Hz, 2H); 13C NMR (CDCl3, 75 MHz): δ 165.4, 149.4, 140.1, 138.4, 128.7, 128.0, 126.7, 123.7, 41.3, 35.4.
N-Benzyl-N-methylbenzamide (3v)32.
Yellow liquid; 1H NMR (CDCl3, 300 MHz): δ 7.35–7.05 (m, 10H), 4.65 (s, 1H), 4.38 (s, 1H), 2.91 (s, 1.5H), 2.72 (s, 1.5H); 13C NMR (CDCl3, 75 MHz): δ 171.5, 170.9, 136.5, 136.1, 135.7, 129.0, 128.4, 128.2, 127.8, 127.5, 127.2, 126.9, 126.3, 54.4, 50.1, 36.3, 32.5.
N-Benzyl-2-bromobenzamide (3y)35.
Yellow solid; 1H NMR (CDCl3, 300 MHz): δ 7.58 (d, J = 7.8 Hz, 1H), 7.5–7.22 (m, 8H), 6.47 (bs, 1H), 4.60 (d, J = 5.7 Hz, 2H); 13C NMR (CDCl3, 75 MHz): δ 167.5, 137.6, 137.6, 133.2, 131.1, 129.4, 128.6, 128.4, 127.9, 127.4, 119.2, 44.0.
4-Chloro-N-(1-phenylethyl)benzamide (3z)36.
White solid; 1H NMR (CDCl3, 300 MHz): δ 7.77–7.74 (m, 2H), 7.38–7.25 (m, 7H), 6.40 (bs, 1H), 5.34–5.25 (m, 1H), 1.60 (d, J = 7.2 Hz, 3H); 13C NMR (CDCl3, 75 MHz): δ 165.5, 142.9, 137.7, 132.9, 128.8, 128.4, 127.5, 126.2, 49.3, 21.6.
4-Nitro-N-(1-phenylethyl)benzamide (3ab)37.
Yellow solid; 1H NMR (CDCl3, 300 MHz): δ 8.23 (d, J = 8.7 Hz, 2H), 7.91 (d, J = 8.4 Hz, 2H) 7.37–7.26 (m, 7H), 6.61 (bs, 1H), 5.35–5.26 (m, 1H), 1.63 (d, J = 7.2 Hz, 3H); 13C NMR (CDCl3, 75 MHz): δ 164.6, 149.5, 142.4, 140.1, 128.8, 128.1, 127.7, 126.2, 123.7, 49.7, 21.5.
Acknowledgements
We are thankful to the Department of Science and Technology (DST), New Delhi for financial support. R. V. and T. G. are thankful to CSIR for their fellowships.
Notes and references
-
(a) J. M. Humphrey and A. R. Chamberlin, Chem. Rev., 1997, 97, 2243 CrossRef CAS PubMed;
(b)
R. C. Larock, Comprehensive Organic Transformation, VCH, New York, 1999 Search PubMed.
- D. J. C. Constable, P. J. Dunn, J. D. Hayler, G. R. Humphrey, J. L. Leazer Jr., R. J. Linderman, K. Lorenz, J. Manley, B. A. Pearlman, A. Wells, A. Zaks and T. Y. Zhang, Green Chem., 2007, 9, 41 RSC.
-
(a) C. Ramalingan and Y.-T. Park, J. Org. Chem., 2007, 72, 4536 CrossRef CAS PubMed;
(b) A. Martínez-Asencio, M. Yus and D. J. Ramón, Tetrahedron, 2012, 68, 3948 CrossRef;
(c) F. Xu, N.-G. Wang, Y.-P. Tian, Y.-M. Chen and W.-C. Liu, Synth. Commun., 2012, 42, 3532 CrossRef CAS;
(d) A. Maia, D. C. M. Albanese and D. Landini, Tetrahedron, 2012, 68, 1947 CrossRef CAS;
(e) J. K. Augustine, R. Kumar, A. Bombrun and A. B. Mandal, Tetrahedron Lett., 2011, 52, 1074 CrossRef CAS.
-
(a) A. Brennführer, H. Neumann and M. Beller, Angew. Chem., Int. Ed., 2009, 48, 4114 CrossRef PubMed;
(b) N. Iranpoor, H. Firouzabadi, S. Motevalli and M. Talebi, Tetrahedron, 2013, 69, 418 CrossRef CAS;
(c) B. Roberts, D. Liptrot, L. Alcaraz, T. Luker and M. J. Stocks, Org. Lett., 2010, 12, 4280 CrossRef CAS PubMed;
(d) Y. Jo, J. Ju, J. Choe, K. H. Song and S.-W. Lee, J. Org. Chem., 2009, 74, 6358 CrossRef CAS PubMed;
(e) P. Nordeman, L. R. Odell and M. Larhed, J. Org. Chem., 2012, 77, 11393 CrossRef CAS PubMed.
-
(a) K. M. Driller, S. Prateeptongkum, R. Jackstell and M. Beller, Angew. Chem., Int. Ed., 2011, 50, 537 CrossRef CAS PubMed;
(b) S. T. Gadge, M. V. Khedkar, S. R. Lanke and B. M. Bhanage, Adv. Synth. Catal., 2012, 354, 2049 CrossRef CAS;
(c) T. Fujihara, Y. Katafuchi, T. Iwai, J. Terao and Y. Tsuji, J. Am. Chem. Soc., 2010, 132, 2094 CrossRef CAS PubMed;
(d) A. Brennführer, H. Neumann and M. Beller, ChemCatChem, 2009, 1, 28 CrossRef.
-
(a) R. F. Cunico and B. C. Maity, Org. Lett., 2002, 4, 4357 CrossRef CAS PubMed;
(b) D. N. Sawant, Y. S. Wagh, K. D. Bhatte and B. M. Bhanage, J. Org. Chem., 2011, 76, 5489 CrossRef CAS PubMed;
(c) D. N. Sawant, Y. S. Wagh, K. D. Bhatte and B. M. Bhanage, Eur. J. Org. Chem., 2011, 6719 CrossRef CAS;
(d) Y. Jo, J. Ju, J. Choe, K. H. Song and S.-W. Lee, J. Org. Chem., 2009, 74, 6358 CrossRef CAS PubMed;
(e) J. Ju, M. Jeong, J. Moon, H. M. Jung and S. Lee, Org. Lett., 2007, 9, 4615 CrossRef CAS PubMed;
(f) R. F. Cunico and R. K. Pandey, J. Org. Chem., 2005, 70, 9048 CrossRef CAS PubMed;
(g) Y. Wan, M. Alterman, M. Larhed and A. Hallberg, J. Org. Chem., 2002, 67, 6232 CrossRef CAS PubMed.
- B. Shen, D. M. Makley and J. N. Johnston, Nature, 2010, 465, 1027 CrossRef CAS PubMed.
-
(a) N. A. Stephenson, J. Zhu, S. H. Gellman and S. S. Stahl, J. Am. Chem. Soc., 2009, 131, 10003 CrossRef CAS PubMed;
(b) J. M. Hoerter, K. M. Otte, S. H. Gellman, Q. Cui and S. S. Stahl, J. Am. Chem. Soc., 2008, 130, 647 CrossRef CAS PubMed;
(c) P. Starkov and T. D. Sheppard, Org. Biomol. Chem., 2011, 9, 1320 RSC;
(d) M. Tamura, T. Tonomura, K.-I. Shimizu and A. Satsuma, Green Chem., 2012, 14, 717 RSC;
(e) M. Zhang, S. Imm, S. Bähn, L. Neubert, H. Neumann and M. Beller, Angew. Chem., Int. Ed., 2012, 51, 3905 CrossRef CAS PubMed;
(f) C. L. Allen, B. N. Atkinson and J. M. J. Williams, Angew. Chem., Int. Ed., 2012, 51, 1383 CrossRef CAS PubMed;
(g) T. B. Nguyen, J. Sorres, M. Q. Tran, L. Ermolenko and A. Al-Mourabit, Org. Lett., 2012, 14, 3202 CrossRef CAS PubMed.
-
(a) W.-J. Yoo and C.-J. Li, J. Am. Chem. Soc., 2006, 128, 13064 CrossRef CAS PubMed;
(b) J. W. W. Chang and P. W. H. Chan, Angew. Chem., Int. Ed., 2008, 47, 1138 CrossRef CAS PubMed;
(c) A. Tillack, I. Rudloff and M. Beller, Eur. J. Org. Chem., 2001, 523 CrossRef CAS;
(d) S. Muthaiah, S. C. Ghosh, J.-E. Jee, C. Chen, J. Zhang and S. H. Hong, J. Org. Chem., 2010, 75, 3002 CrossRef CAS PubMed;
(e) S. Seo and T. J. Marks, Org. Lett., 2008, 10, 317 CrossRef CAS PubMed;
(f) S. C. Ghosh, J. S. Y. Ngiam, A. M. Seayad, D. H. Tuan, C. L. L. Chai and A. Chen, J. Org. Chem., 2012, 77, 8007 CrossRef CAS PubMed;
(g) C. Qian, X. Zhang, J. Li, F. Xu, Y. Zhang and Q. Shen, Organometallics, 2009, 28, 3856 CrossRef CAS;
(h) S. C. Ghosh, J. S. Y. Ngiam, C. L. L. Chai, A. M. Seayad, D. T. Tuan and A. Chen, Adv. Synth. Catal., 2012, 354, 1407 CrossRef CAS.
-
(a) G. H. Oleman, J. Am. Chem. Soc., 1933, 55, 3001 CrossRef;
(b) P. Sinha and P. Knochel, Synlett, 2006, 3304 CAS;
(c) T. J. Barker and E. R. Jarvo, J. Am. Chem. Soc., 2009, 131, 15598 CrossRef CAS PubMed.
- T. Awano, K. Hirano, T. Satoh and M. Miura, J. Am. Chem. Soc., 2010, 132, 6900 CrossRef PubMed.
- S. P. Bew, D. L. Hughes, N. J. Palmer, V. Savic, K. M. Soapi and M. A. Wilson, Chem. Commun., 2006, 4338 RSC.
-
(a) A. Porcheddu, G. Giacomelli and L. D. Luca, Adv. Synth. Catal., 2012, 354, 2949 CrossRef CAS;
(b) R. Cadoni, A. Porcheddu, G. Giacomelli and L. D. Luca, Org. Lett., 2012, 14, 5014 CrossRef CAS PubMed.
-
(a) R. Vanjari, T. Guntreddi and K. N. Singh, Org. Lett., 2013, 15, 4908 CrossRef CAS PubMed;
(b) R. Vanjari, B. K. Allam and K. N. Singh, RSC Adv., 2013, 3, 1691 RSC;
(c) R. Vanjari, B. K. Allam and K. N. Singh, Tetrahedron Lett., 2013, 54, 2553 CrossRef CAS.
-
(a) T. Guntreddi, B. K. Allam and K. N. Singh, Synlett, 2012, 2635 CAS;
(b) T. Guntreddi, B. K. Allam and K. N. Singh, RSC Adv., 2013, 3, 9875 RSC;
(c) D. S. Raghuvanshi, A. K. Gupta and K. N. Singh, Org. Lett., 2012, 14, 4326 CrossRef CAS PubMed;
(d) N. Singh, B. K. Allam, D. S. Raghuvanshi and K. N. Singh, Adv. Synth. Catal., 2013, 355, 1840 CrossRef CAS;
(e) R. Singh, B. K. Allam, D. S. Raghuvanshi and K. N. Singh, Tetrahedron, 2013, 69, 1038 CrossRef CAS;
(f) K. Kumari, D. S. Raghuvanshi and K. N. Singh, Tetrahedron, 2013, 69, 82 CrossRef CAS.
-
(a) K. Ekoue-Kovi and C. Wolf, Org. Lett., 2007, 9, 3429 CrossRef CAS PubMed;
(b) Z. Liu, J. Zhang, S. Chen, E. Shi, Y. Xu and X. Wan, Angew. Chem., Int. Ed., 2012, 51, 3231 CrossRef CAS PubMed.
-
(a) P. Kovacic, M. K. Lowery and K. W. Field, Chem. Rev., 1970, 70, 639 CrossRef CAS;
(b) S. Minakata, Acc. Chem. Res., 2009, 42, 1172 CrossRef CAS PubMed;
(c) P. G. Gassman, D. K. Dygos and J. E. Trent, J. Am. Chem. Soc., 1970, 92, 2084 CrossRef CAS.
- W. R. Bowman, H. Heaney and B. M. Jordan, Tetrahedron, 1991, 47, 10119 CrossRef CAS.
-
(a) V. Martinez-Barrasa, A. Garcia de Viedma, C. Burgos and J. Alvarez-Builla, Org. Lett., 2000, 2, 3933 CrossRef CAS PubMed;
(b) D. L. Clive and S. Kang, Tetrahedron Lett., 2000, 41, 1315 CrossRef CAS.
- J. J. Warren and J. M. Mayer, J. Am. Chem. Soc., 2010, 132, 7784 CrossRef CAS PubMed.
- J. Wang, C. Liu, J. Yuan and A. Lei, Angew. Chem., Int. Ed., 2013, 52, 2256 CrossRef CAS PubMed.
- L. U. Nordstrom, H. Vogt and R. Madsen, J. Am. Chem. Soc., 2008, 130, 17672 CrossRef CAS PubMed.
- H. Lundberg, F. Tinnis and H. Adolfsson, Chem.–Eur. J., 2012, 18, 3822 CrossRef CAS PubMed.
- C. Cazorla, E. Métay and M. Lemaire, Tetrahedron, 2011, 67, 8615 CrossRef CAS.
- T. Ohshima, Y. Hayashi, K. Agura, Y. Fujii, A. Yoshiyama and K. Mashima, Chem. Commun., 2012, 48, 5434 RSC.
- W. Ren and M. Yamane, J. Org. Chem., 2010, 75, 3017 CrossRef CAS PubMed.
- S. D. Sarkar and A. Studer, Org. Lett., 2010, 12, 1992 CrossRef PubMed.
- X. Cui, Y. Zhang, F. Shi and Y. Deng, Chem.–Eur. J., 2011, 17, 1021 CrossRef CAS PubMed.
- T. T. Dang, Y. Zhu, S. C. Ghosh, A. Chen, C. L. L. Chai and A. M. Seayad, Chem. Commun, 2012, 48, 1805 RSC.
- K. Hioki, S. Kameyama, S. Tani and M. Kunishima, Chem. Pharm. Bull., 2007, 55, 825 CrossRef CAS PubMed.
- H. Yao and K. Yamamoto, Chem. Asian J., 2012, 7, 1542 CrossRef CAS PubMed.
- J. Wang, J. Li, F. Xu and Q. Shen, Adv. Synth. Catal., 2009, 351, 1363 CrossRef CAS.
- W. Ren and M. Yamane, J. Org. Chem., 2010, 75, 8410 CrossRef CAS PubMed.
- Y. H. Ye, J. Zhang, G. Wang, S. Y. Chen and X. Q. Yu, Tetrahedron, 2011, 67, 4649 CrossRef CAS.
- A. Rolfe, K. Young and P. R. Hanson, Eur. J. Org. Chem., 2008, 5254 CrossRef CAS PubMed.
- Y. Kasashima, A. Uzawa, K. Hashimoto, Y. Yokoyama, T. Mino, M. Sakamoto and T. J. Fujita, Oleo Sci., 2010, 59, 607 CrossRef CAS.
- Z. Wang, Y. Zhang, H. Fu, Y. Jiang and Y. Zhao, Org. Lett., 2008, 10, 1863 CrossRef CAS PubMed.
Footnote |
† Electronic supplementary information (ESI) available: Experimental procedure and spectral data. See DOI: 10.1039/c3gc41548a |
|
This journal is © The Royal Society of Chemistry 2014 |