DOI:
10.1039/C4FO00113C
(Paper)
Food Funct., 2014,
5, 1441-1451
Study on chemical, bioactive and food preserving properties of Laetiporus sulphureus (Bull.: Fr.) Murr.
Received
15th February 2014
, Accepted 5th April 2014
First published on 7th April 2014
Abstract
Laetiporus sulphureus (Bull.: Fr.) Murr. was studied to determine the nutritional value, bioactive compounds, in vitro antioxidants, and antimicrobial and antitumor activities. The studied mushroom is a rich source of carbohydrates and proteins. Mannitol and trehalose were the main free sugars. In addition, the polyunsaturated fatty acids α-, γ- and δ-tocopherols were found. Oxalic and citric acids were the most abundant organic acids; cinnamic and p-hydroxybenzoic acids were quantified in the methanolic extract and could be related to the antioxidant properties. It was the polysaccharidic extract that exhibited higher antioxidant and antimicrobial activities, indicating that the compounds present in this extract possess stronger bioactivity. Only the polysaccharidic extract revealed antiproliferative activity in human tumor cell lines. In addition, a suitable model system with chicken pâté was developed to test the antimicrobial preserving properties of L. sulphureus. The methanolic extract was used to examine in situ preserving properties against Aspergillus flavus and demonstrated excellent preserving potential.
Introduction
Mushrooms are consumed in many countries as a culinary delicacy, particularly for their taste.1 In recent years, scientists have expanded their research area concerning other uses of mushrooms, particularly for medicinal and food-preserving purposes.
Regarding medicinal value, wild mushrooms have emerged as an important source of antioxidant compounds, which is very important because the intake of foods rich in compounds that could eliminate free radicals has been recommended. These reactive species are produced as a part of the normal process of aerobic metabolism, but they can cause structural damage to cells and have been linked to multiple chronic diseases such as various types of cancer, cardiovascular diseases, and diabetes.2,3 Particularly, cancer is among the most common diseases. According to World Health Organization, more than 10 million new cases of cancer are diagnosed every year, and the statistical trends indicate that this number will double by 2020.4 Studies and practice in Eastern medicine have shown that certain mushrooms have extraordinary effects on people dealing with various types of cancer, and some of them have been used since the 16th or 17th centuries.5,6
Regarding food-preserving properties, a number of successful studies have been recently carried out,7–9 making clear the considerable differences among the chemical composition, nutritional value and bioactive effects of the mushroom species. Therefore, a detailed chemical analysis of each source of bioactive compounds is necessary.
Conversely, increasing evidence that artificial preservatives (e.g., butylated hydroxyanisole – BHA, butylated hydroxytoluene – BHT and tertiary butyl hydroquinone – TBHQ) are carcinogenic has pressured the food industry to start searching for novel substances to prolong the shelf life of products. Accordingly, natural sources appeared as excellent alternatives for replacing synthetic antioxidants.10,11
Meat and meat products are highly susceptible to spoilage due to microbial growth, oxidation and enzymatic autolysis. Traditional methods such as salting, drying and smoking are still used,12 but they do not meet standards of novel consumers who demand natural ingredients as preservatives. Bearing in mind that food spoilage is related to the growth of microorganisms and free-radical production, natural sources of antioxidant and antimicrobial compounds should be explored. Laetiporus sulphureus (Bull.: Fr.) Murr. is a suitable candidate for any survey because it can be easily found and recognized in nature, but it can also be easily cultivated.13 Therefore, this edible mushroom is considered as a promising candidate for biotechnology studies.
The objective of the present study was to evaluate the chemical composition of L. sulphureus and in vitro bioactive properties, particularly the antioxidant, antitumor and antimicrobial components of its methanolic and polysaccharidic extracts. Furthermore, the preservative efficiency of the methanolic extract was studied using chicken pâté as model system and the mould A. flavus as the contaminant fungus. It should be noted that this study was carried out under in situ conditions, using actual foods and general storage conditions, rather than microbiological media (in vitro systems), which is a significant advantage over other available studies regarding meat-preserving activity through natural matrices.
Materials and methods
Mushroom species
L. sulphureus was collected from the trees of Salix alba in Jojkićev branch of the Danube River, near Pančevo, Northern Serbia, during May 2011 and authenticated by Dr Jasmina Glamočlija (Institute for Biological Research). A voucher specimen has been deposited at the Fungal Collection Unit of the Mycological Laboratory, Department for Plant Physiology, Institute for Biological Research “Siniša Stanković”, Belgrade, Serbia, under the number Ls-610-2011. Fresh fruiting bodies were randomly divided to smaller samples and immediately dried by lyophilization (LH Leybold, Lyovac GT2, Frenkendorf). Upon reaching constant mass, the specimens were milled to a fine powder, mixed to obtain a homogenate sample and kept at 4 °C until further analysis.
Standards and reagents
Acetonitrile 99.9%, n-hexane 95% and ethyl acetate 99.8% were of HPLC grade from Fisher Scientific (Lisbon, Portugal). The fatty acid methyl ester (FAME) reference standard mixture 37 (standard 47885-U) was purchased from Sigma (St. Louis, MO, USA), as also other individual fatty-acid isomers and standards of tocopherols, sugars, organic acids and phenolic compounds, and trolox (6-hydroxy-2,5,7,8-tetramethylchroman-2-carboxylic acid). Racemic tocol, 50 mg mL−1, was purchased from Matreya (PA, USA). 2,2-Diphenyl-1-picrylhydrazyl (DPPH) was obtained from Alfa Aesar (Ward Hill, MA, USA). Mueller-Hinton agar (MH) and malt agar (MA) were obtained from the Institute of Immunology and Virology, Torlak (Belgrade, Serbia). Dimethylsulfoxide (DMSO; Merck KGaA, Germany) was used as a solvent. Phosphate-buffered saline (PBS) was obtained from Sigma Chemical Co. (St. Louis, USA). Fetal bovine serum (FBS), L-glutamine, Hank's Balanced Salt Solution (HBSS), trypsin–EDTA (ethylenediaminetetraacetic acid), non-essential amino acids solution (2 mM), penicillin/streptomycin solution (100 U mL−1 and 100 mg mL−1, respectively), RPMI-1640 and DMEM media were obtained from HyClone (Logan, USA). Acetic acid, ellipticine, sulforhodamine B (SRB), trypan blue, trichloroacetic acid (TCA) and Tris were from Sigma Chemical Co. (St. Louis, Mo., USA). Methanol and all other chemicals and solvents were of analytical grade and purchased from common sources. Water was treated in a Milli-Q water purification system (TGI Pure Water Systems, USA).
Chemical characterization of L. sulphureus
Nutritional value.
The samples were analyzed for chemical composition (moisture, proteins, fats, carbohydrates and ash) by using the procedures described by the Association of Official Analytical Chemists (AOAC).14 The crude protein content (N × 4.38) of the samples was estimated by the macro-Kjeldahl method; the crude fat was determined by extracting a known weight of powdered sample with petroleum ether, using a Soxhlet apparatus; the ash content was determined by incineration at 600 ± 15 °C. Total carbohydrates were calculated by their difference. Energy was calculated according to the following equation: energy (kcal) = 4 × (g protein + g carbohydrate) + 9 × (g fat).
Sugars composition.
Free sugars were determined by a high-performance liquid chromatography (HPLC) system consisting of an integrated system with a pump (Knauer, Smartline system1000), degasser system (Smartline manager 5000) and an auto-sampler (AS-2057 Jasco), coupled with a refraction index detector (RI detector KnauerSmartline 2300) as previously described by the authors.15 Sugar identifications were made by comparing the relative retention times of sample peaks with standards. Data were analyzed using Clarity 2.4 Software (DataApex). Quantification was based on the RI signal response of each standard, using the internal standard (IS, raffinose) method and by using calibration curves obtained from the commercial standards of each compound. The results were expressed in g per 100 g of dry weight.
Fatty acids composition.
Fatty acids were determined after a transesterification procedure as described previously by the authors,15 using a gas chromatographer (DANI 1000) equipped with a split/splitless injector and a flame ionization detector (GC-FID). Fatty acid identification was made by comparing the relative retention times of FAME peaks from samples with standards. The results were recorded and processed using CSW 1.7 software (DataApex 1.7), and expressed in the relative percentage of each fatty acid.
Tocopherols composition.
Tocopherols were determined following a procedure previously optimized and described by the authors.16 The analysis was performed by HPLC (equipment previously described) and a fluorescence detector (FP-2020; Jasco) programmed for excitation at 290 nm and emission at 330 nm. The compounds were identified by chromatographic comparisons with authentic standards. Quantification was based on the fluorescence signal response of each standard, using the IS (tocol) method and by using calibration curves obtained from commercial standards of each compound. The results were expressed in μg per 100 g of dry weight.
Organic acids composition.
Organic acids were determined following a procedure previously optimized and described by the authors.17 Analysis was performed by an ultra-fast liquid chromatograph (UFLC) coupled with a photodiode array detector (PDA), using a Shimadzu 20A series UFLC (Shimadzu Corporation). Detection was carried out in a PDA, using 215 nm and 245 nm as preferred wavelengths. The organic acids were quantified by the comparison of the area of their peaks recorded at 215 nm with calibration curves obtained from commercial standards of each compound. The results were expressed in g per 100 g of dry weight.
Phenolic compounds composition.
Phenolic acids were determined by HPLC (Hewlett-Packard 1100, Agilent Technologies, Santa Clara, CA, USA) as previously described by Barros et al.18 Detection was carried out in a diode array detector (DAD) by using 280 nm as the preferred wavelength. The phenolic compounds were quantified by comparison of the area of their peaks recorded at 280 nm with the calibration curves obtained from the commercial standards of each compound. The results were expressed in μg per 100 g of dry weight.
Preparation of methanolic and polysaccharidic extracts
The powder of L. sulphureus (10 g) was extracted by stirring with 250 mL of methanol at −20 °C for 6 h. The extract was sonicated for 15 minutes, centrifuged at 4000 g for 10 minutes, and subsequently filtered through Whatman no. 4 paper. The residue was then extracted with two additional portions of methanol (l50 mL of solvent each time). The combined extract was evaporated at 40 °C (rotary evaporator Büchi R-210) to dryness and re-dissolved in 30% ethanol.18 The yield of methanolic extract was 9.8%.
Polysaccharides were extracted from the powder mushrooms (1.5 g) by stirring with boiling water (50 mL) for 2 h before being subsequently filtered through Whatman no. 4 paper. The residue was then extracted with two further portions of boiling water over a total extraction time of 6 h. The combined extracts were lyophilized before 95% ethanol (10 mL) was added, and polysaccharides were precipitated overnight at 4 °C. The precipitated polysaccharides were collected after centrifugation at 3.100 g for 40 min, followed by filtration before being lyophilized, resulting in a crude polysaccharidic sample.19 The yield of the extract was 2.5%.
The extracts were re-dissolved in (i) methanol or water (final concentration 20 mg mL−1) for antioxidant activity evaluation, and (ii) water for antitumor (final concentration 8 mg mL−1) and antimicrobial (final concentration 1.5 mg mL−1) activity evaluation. The final solutions were further diluted to different concentrations to be submitted for distinct bioactivity evaluations in vitro assays. The results were expressed in (i) EC50 values (sample concentration providing 50% of antioxidant activity or 0.5 of absorbance in the reducing power assay) for antioxidant activity; (ii) GI50 values (sample concentration that inhibited 50% of the net cell growth) for antiproliferative activity in human tumor cell lines and non-tumor liver primary cell culture; and (iii) MIC (minimum inhibitory concentration) and MBC/MFC (minimum bactericidal concentration/minimum fungicidal concentration) values for antimicrobial activity. Trolox and ellipticine were used as positive controls in antioxidant and antitumor activity evaluation assays, respectively. Streptomycin and ampicillin were used as positive controls in the antibacterial assay, while bifonazole and ketokonazole were used as positive controls in the antifungal susceptibility test.
Antioxidant activity of L. sulphureus methanolic and polysaccharidic extracts
DPPH (2,2-diphenyl-1-picrylhydrazyl) scavenging activity.
The reaction mixture on 96 well plates consisted of a solution by the well of the extract solutions with different concentrations (30 μL) and a methanol solution (270 μL) containing DPPH radicals (6 × 10−5 mol L−1). The mixture was left to stand for 30 min in darkness, and the absorption was measured at 515 nm by an ELX800 Microplate Reader (Bio-Tek Instruments).20 The radical scavenging activity (RSA) was calculated as a percentage of DPPH discoloration using the equation % RSA = [(ADPPH − AS)/ADPPH] × 100, where AS is the absorbance of the solution containing the sample, and ADPPH is the absorbance of the DPPH solution.
Reducing power
The extract solutions with different concentrations (0.5 mL) were mixed with a sodium phosphate buffer (200 mmol L−1, pH 6.6, 0.5 mL) and potassium ferricyanide (1% w/v, 0.5 mL). The mixture was incubated at 50 °C for 20 min, and trichloroacetic acid (10% w/v, 0.5 mL) was added. The mixture (0.8 mL) was poured in the 48 well plates, the same with deionized water (0.8 mL) and ferric chloride (0.1% w/v, 0.16 mL), and the absorbance was measured at 690 nm by the aforementioned microplate reader.20
β-Carotene bleaching inhibition
A solution of β-carotene was prepared by dissolving β-carotene (2 mg) in chloroform (10 mL). Two milliliters of this solution were pipetted into a round-bottom flask. Chloroform was removed at 40 °C under vacuum. Linoleic acid (40 mg), Tween 80 emulsifier (400 mg) and distilled water (100 mL) were added to the flask with vigorous shaking. Aliquots (4.8 mL) of this emulsion were transferred into test tubes containing extract solutions with various concentrations (0.2 mL). The tubes were shaken and incubated at 50 °C in a water bath. As soon as the emulsion was added to each tube, the zero time absorbance was measured at 470 nm.20 β-Carotene bleaching inhibition was calculated using the following equation: (absorbance after 2 h of assay/initial absorbance) × 100.
TBARS (thiobarbituric acid reactive substances) inhibition
Porcine (Susscrofa) brains were obtained from official slaughtering animals, dissected and homogenized with a Polytron in an ice-cold Tris–HCl buffer (20 mM, pH 7.4) to produce a 1
:
2 w/v brain tissue homogenate, which was centrifuged at 3000 g for 10 min. An aliquot (100 μL) of the supernatant was incubated with different concentrations of the sample solutions (200 μL) in the presence of FeSO4 (10 mM; 100 μL) and ascorbic acid (0.1 mM; 100 μL) at 37 °C for 1 h. The reaction was stopped by the addition of trichloroacetic acid (28% w/v, 500 μL), followed by thiobarbituric acid (TBA 2%, w/v, 380 μL), and the mixture was then heated at 80 °C for 20 min. After centrifugation at 3000 g for 10 min to remove the precipitated protein, the color intensity of the malondialdehyde (MDA)–TBA complex in the supernatant was measured by its absorbance at 532 nm.20 The inhibition ratio (%) was calculated using the following formula: inhibition ratio (%) = [(A − B)/A] × 100%, where A and B were the absorbance of the control and the sample solution, respectively.
Antiproliferative activity of L. sulphureus methanolic and polysaccharidic extracts against human tumor cell lines and non-tumor liver primary cells
Five human tumor cell lines were tested: MCF-7 (breast adenocarcinoma), NCI-H460 (non-small-cell lung cancer), HCT-15 (colon carcinoma), HeLa (cervical carcinoma) and HepG2 (hepatocellular carcinoma). Cells were routinely maintained as adherent cell cultures in RPMI-1640 medium containing 10% heat-inactivated FBS (MCF-7, NCI-H460 and HCT-15) and 2 mM glutamine or in DMEM supplemented with 10% FBS, 2 mM glutamine, 100 U mL−1 penicillin and 100 mg mL−1 streptomycin (HeLa and HepG2 cells), at 37 °C, in a humidified air incubator containing 5% CO2. Each cell line was plated at an appropriate density (7.5 × 103 cells per well for MCF-7, NCI-H460 and HCT-15 or 1.0 × 104 cells per well for HeLa and HepG2) in 96-well plates and allowed to attach for 24 h. Cells were then treated for 48 h with the diluted fraction/extract solutions. Following this incubation period, the adherent cells were fixed by adding cold 10% trichloroacetic acid (TCA, 100 μL) and incubated for 60 min at 4 °C. Plates were then washed with deionized water and dried. Sulforhodamine B solution (0.1% in 1% acetic acid, 100 μL) was then added to each plate well and incubated for 30 min at room temperature. Unbound SRB was removed by washing with 1% acetic acid. Plates were air-dried, the bound SRB was solubilised with 10 mM Tris (200 μL, pH 7.4) and the absorbance was measured at 540 nm in the aforementioned microplate reader.21
A cell culture, which was designed as PLP2, was also prepared from a freshly harvested porcine liver obtained from a local slaughterhouse. Briefly, the liver tissues were rinsed in Hank's balanced salt solution containing 100 U mL−1 penicillin and 100 μg mL−1 streptomycin, and divided into 1 × 1 mm3 explants. Some of these explants were placed in 25 cm2 tissue flasks in DMEM, supplemented with 10% fetal bovine serum, 2 mM nonessential amino acids, 100 U mL−1 penicillin and 100 mg mL−1 streptomycin, and incubated at 37 °C in a humidified atmosphere containing 5% CO2. The medium was changed every two days. Cultivation of the cells was continued with direct monitoring every two to three days using a phase contrast microscope. Before confluence, the cells were sub-cultured and plated in 96-well plates at a density of 1.0 × 104 cells per well, and cultivated in a DMEM medium with 10% FBS, 100 U mL−1 penicillin and 100 μg mL−1 streptomycin.22 The cells were treated for 48 h with the different diluted sample solutions, and the same procedure described for the SRB assay was followed.
Antimicrobial activity of L. sulphureus methanolic and polysaccharidic extracts
Antibacterial activity assays.
The following Gram-negative bacteria—Escherichia coli (ATCC 35210), Pseudomonas aeruginosa (ATCC 27853), Salmonella typhimurium (ATCC 13311)—and Gram-positive bacteria—Staphylococcus aureus (ATCC 6538), Micrococcus flavus (ATCC 10240) and Listeria monocytogenes (NCTC 7973)—were used. The microorganisms were obtained from the Mycological Laboratory, Department of Plant Physiology, Institute for Biological Research “Siniša Stanković”, University of Belgrade, Serbia.
The minimum inhibitory (MIC) and minimum bactericidal (MBC) concentrations were determined by the microdilution method. Briefly, a fresh overnight culture of bacteria was adjusted by the spectrophotometer to a concentration of 1 × 105 CFU mL−1. The requested CFU mL−1 corresponded to a bacterial suspension determined in a spectrophotometer at 625 nm (OD625). Dilutions of inocula were cultured on a solid medium to verify the absence of contamination and to ensure the validity of the inoculum. Various extract solutions were carried out over the wells containing 100 μL of Tryptic Soy Broth (TSB) and afterward, 10 μL of inoculum was added to all of the wells. The microplates were incubated for 24 h at 37 °C. The MIC of the samples was detected following the addition of 40 μL of iodonitrotetrazolium chloride (INT) (0.2 mg mL−1) and incubation at 37 °C for 30 min. The lowest concentration that produced a significant inhibition of the growth of the bacteria in comparison with the positive control was identified as the MIC. The MICs obtained from the susceptibility testing of various bacteria to the tested extract were determined also by a colorimetric microbial viability assay based on the reduction of a INT color and compared with a positive control for each bacterial strain.23,24 The MBC was determined by serial sub-cultivation of 10 μL into microplates containing 100 μL of the TSB. The lowest concentration that shows no growth after this sub-culturing was read as the MBC. 5% DMSO was used as the negative control.
Antifungal activity assays.
For the antifungal bioassays, the following microfungi were used: Aspergillus fumigatus (ATCC 1022), Aspergillus ochraceus (ATCC 12066), Aspergillus versicolor (ATCC 11730), Trichoderma viride (IAM 5061), Penicillium ochrochloron (ATCC 9112) and Penicillium verrucosum var. cyclopium (food isolate). The organisms were obtained from the Mycological Laboratory, Department of Plant Physiology, Institute for Biological Research “Siniša Stanković”, Belgrade, Serbia. The micromycetes were maintained on malt agar (MA), and the cultures were stored at 4 °C and sub-cultured once a month.25
The fungal spores were washed from the surface of agar plates with a sterile 0.85% saline containing 0.1% Tween 80 (v/v). The spore suspension was adjusted with sterile saline to a concentration of approximately 1.0 × 105 in a final volume of 100 μL per well. The inocula were stored at 4 °C for further use. Dilutions of the inocula were cultured on the solid MA to verify the absence of contamination and to check the validity of the inoculum.
MIC determination was performed by a serial-dilution technique using 96-well microtitre plates. The solution extracts were added to a broth malt medium with a fungal inoculum. The microplates were incubated for 72 h at 28 °C. The lowest concentrations without visible growth (with a binocular microscope) were defined as the MIC. The minimum fungicidal concentrations (MFCs) were determined by a serial subcultivation of 2 μL in microtitre plates containing 100 μL of malt broth per well and further incubation for 72 h at 28 °C. The lowest concentration with no visible growth was defined as the MFC, indicating a 99.5% killing of the original inoculum. 5% DMSO was used as a negative control.26
Chicken pâté
Chicken pâté, produced from the chicken meat by Neoplanta, Novi Sad, Serbia, was purchased from a supermarket and kept unopened in the refrigerator at 4 °C until further analyses. Upon opening, the pâté was immediately used in the experiments. The sample was used before the expiry date of the product. On the label the nutritional value per 100 g of the product is clearly defined. The composition of the chicken pâté listed on the packaging was proteins, 11 g, fats, 21 g; carbohydrates, 3 g; and energy value per 100 g, 253 kcal. The packaging listed no artificial preservatives. Experiments on inoculating malt agar (MA) and Muller-Hinton agar (MHA) plates with pâté diluted by 1 in 10 with phosphate-buffered saline (PBS) and kept at 25 °C and 37 °C for 48 h, respectively, showed no bacterial or fungal contamination of the product.
Isolation and identification of chicken pâté contaminant micromycete
Chicken pâté was left open at 4 °C for 30 days. After that period, the experiment was repeated with dilutions to check for contaminants. The MA plates with micromycete were inoculated with different dilutions of pâté in PBS and kept at 25 °C to investigate possible fungal contaminants. Pure fungal culture was isolated from inoculated MA plates after 48 h and identified by Dr Milica Ljaljević-Grbić (Faculty of Biology, University of Belgrade) as Aspergillus flavus. The specimen was deposited in the Micromycetes Collection Unit of the Mycological Laboratory, Department for Plant Physiology, Institute for Biological Research “Siniša Stanković”, Belgrade, Serbia, under the number Af-CP-01.
In situ antifungal assay in chicken pâté
Prior to the experiment, chicken pâté was held in a refrigerated state. Packaging was carefully opened under sterile conditions. The surface of the product was prepared for the inoculation by making wells (3 mm deep and 3 mm wide) with a sterile nail, with three wells per package. Each treatment had two replicates with five packages per replicate. Methanolic mushroom extract was dissolved in various concentrations in sterile saline containing 0.2% Tween 80 (v/v). Then 20 μL of the extract was placed into each well. After 1 h, 20 μL of a conidial suspension (106 CFU mL−1) of A. flavus was added to each well. Treated chicken pâté products were put in sterile plastic boxes. The treated samples were stored at 4 °C for 21 days. After the storage period, the number of wells that showed contamination was recorded and contamination incidence (%) was calculated as follows:
The inhibition percentage was also calculated, by comparing growth in non-treated control samples with treated samples that possessed growth of the mould. Diameters of the growth zones in control samples (measured on 0 day, 7th day, 14th day and 21st day) were used as 100% growth, while growth inhibition in treated samples was calculated as follows, and the results were presented graphically:
Results and discussion
Chemical composition
Our literature survey showed that there are available studies concerning L. sulphureus polysaccharides,13 enzymes27 and pigments28 but not in the chemical parameters analysed herein.
Results regarding the nutritional value of L. sulphureus are presented in Table 1. Carbohydrates and proteins are the most abundant compounds (72.64 g per 100 g dw and 15.97 g per 100 g dw, respectively). Mushrooms are generally considered to be a good source of digestable proteins and are reported to contain all of the essential amino acids needed in the human diet.29 Ash content is reported to be low (9.03 g per 100 g dw). L. sulphureus is also poor in fat content (2.35 g per 100 g dw) and caloric value (375 kcal per 100 g dw), which makes this mushroom a good candidate for low-calorie diets. Trehalose was the dominant sugar (4 g per 100 g dw), followed nearly by mannitol (3.54 g per 100 g dw) (Fig. 1A). This is in concordance with previous reports on the composition of mushroom sugars.30
Table 1 Nutritional value, sugars and fatty acid compositions of L. sulphureus (mean ± SD)a
Nutritional parameter |
Amount |
Fat |
2.35 ± 0.50 g per 100 g dw |
Proteins |
15.97 ± 0.13 g per 100 g dw |
Ash |
9.03 ± 0.82 g per 100 g dw |
Carbohydrates |
72.64 ± 0.88 g per 100 g dw |
Energy |
375.62 ± 0.54 kcal per 100 g dw |
Free sugars |
Amount (g per 100 g dw) |
Fructose |
0.46 ± 0.02 |
Mannitol |
3.54 ± 0.06 |
Trehalose |
4.00 ± 0.12 |
Total sugars |
8.00 ± 0.17 |
Fatty acids |
Percentage (%) |
SFA, saturated fatty acids; MUFA, monounsaturated fatty acids; PUFA, polyunsaturated fatty acids; dw, dry weight.
|
C6:0 Caproic acid |
0.08 ± 0.01 |
C8:0 Caprylic acid |
0.10 ± 0.00 |
C10:0 Capric acid |
0.07 ± 0.00 |
C12:0 Lauric acid |
0.12 ± 0.00 |
C14:0 Myristic acid |
0.47 ± 0.01 |
C14:1 Myristoleic acid |
0.00 ± 0.00 |
C15:0 Pentadecanoic acid |
1.41 ± 0.03 |
C16:0 Palmitic acid |
11.68 ± 0.19 |
C16:1 Palmitoleic acid |
0.19 ± 0.00 |
C17:0 Heptadecanoic acid |
1.94 ± 0.04 |
C18:0 Stearic acid |
3.17 ± 0.02 |
C18:1n9c Oleic acid |
14.52 ± 0.02 |
C18:2n6c Linoleic acid |
63.27 ± 0.26 |
C18:3n3 Linolenic acid |
0.17 ± 0.01 |
C20:0 Arachidic acid |
0.19 ± 0.00 |
C20:1 cis-11-Eicosenoic acid |
0.51 ± 0.01 |
C20:2 cis-11,14-Eicosadienoic acid |
0.12 ± 0.01 |
C20:3n3 + C21:0 cis-11,14,17-Eicosatrienoic acid + heneicosanoic acid |
0.17 ± 0.01 |
C20:5n3 cis-5,8,11,14,17-Eicosapentaenoic acid |
0.40 ± 0.02 |
C22:0 Behenic acid |
0.43 ± 0.01 |
C22:1n9 Erucic acid |
0.04 ± 0.01 |
C23:0 Tricosanoic acid |
0.15 ± 0.00 |
C24:0 Lignoceric acid |
0.74 ± 0.00 |
C24:1 Ceramide |
0.07 ± 0.00 |
SFA |
20.54 ± 0.27 |
MUFA |
15.32 ± 0.04 |
PUFA |
64.14 ± 0.23 |
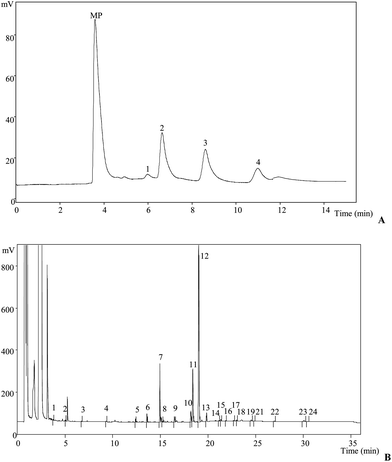 |
| Fig. 1
L. sulphureus individual profile in (A) sugars: 1, fructose; 2, mannitol; 3, trehalose; 4, raffinose (IS); (B) fatty acids: 1, C6:0; 2, C8:0; 3, C10:0; 4, C12:0; 5, C14:0; 6, C15:0; 7, C16:0; 8, C16:1; 9, C17:0; 10, C18:0; 11, C18:1n9; 12, C18:2n6; 13, C18:3n3; 14, 20:0; 15, C20:1; 16, C20:2; 17, C20:3n3 + C21:0; 18, C20:5n3: 19, C22:0; 20, C22:1n9; 21, C23:0; 22, C24:0; and 23, C24:1. | |
Linoleic acid (C18:2n6c) was the most abundant fatty acid (Table 1, 63.27%), followed by oleic acid (C18:1n9c, 14.52%) and palmitic acid (C16:0, 11.68%; Fig. 1B). L. sulphureus is rich in polyunsaturated fatty acids, PUFA (64.14%), while saturated and monounsaturated fatty acids are present in lower amounts (20.54% and 15.32%, respectively). As previously mentioned, mushrooms are known for their low fat content but also for high proportion of PUFA, some of them essential fatty acids, which makes them healthy foods.30,31
In addition, tocopherols were determined because these compounds have the important ability to scavenge free radicals and are believed to protect our systems against degenerative diseases. For a long time, α-tocopherol was considered to be the most active form of vitamin E and was reported to have the highest biological activity. However, recent studies have shown that the other forms are also active.16 For the studied mushroom, α-tocopherol was the most abundant isoform (Table 2, 109.25 μg per 100 g dw), followed by γ-tocopherol (62.07 μg per 100 g dw) and δ-tocopherol (18.42 μg per 100 g dw); β-tocopherol was not present (Fig. 2A).
Table 2 Tocopherols and organic acids and phenolic compound compositions of L. sulphureus (mean ± SD)
Tocopherols (μg per 100 g per dry weight) |
α-Tocopherol |
γ-Tocopherol |
δ-Tocopherol |
Total |
109.25 ± 3.08 |
62.07 ± 2.70 |
18.42 ± 1.31 |
189.91 ± 0.93 |
Organic acids (g per 100 g per dry weight) |
Oxalic acid |
Quinic acid |
Citric acid |
Fumaric acid |
Total |
2.66 ± 0.03 |
0.16 ± 0.01 |
1.24 ± 0.06 |
0.25 ± 0.00 |
4.31 ± 0.01 |
Phenolic and related compounds (μg per 100 g per dry weight) |
p-Hydroxybenzoic acid |
Cinnamic acid |
Total |
30.71 ± 0.20 |
144.57 ± 1.82 |
175.28 ± 1.62 |
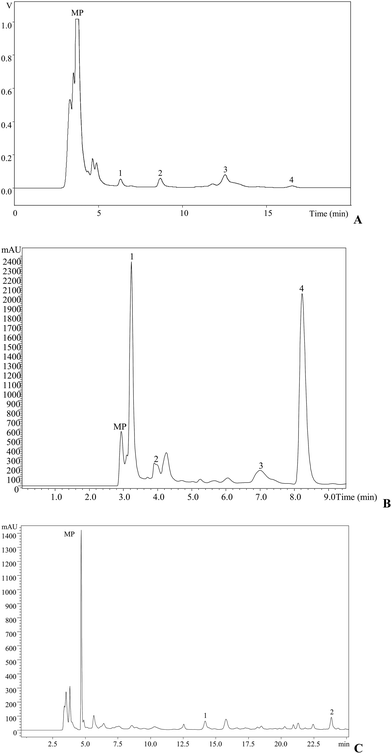 |
| Fig. 2
L. sulphureus individual profile in (A) tocopherols: 1, α-tocopherol; 2, γ-tocopherol; and 3, δ-tocopherol. Organic acid (B): 1, oxalic acid; 2, quinic acid; 3, citric acid; and 4, fumaric acid, recorded at 215 nm. (C) Phenolic compounds: 1, p-hydroxybenzoic acid; and 2, cinnamic acid, recorded at 280 nm. MP, mobile phase. | |
Regarding organic acid composition (Fig. 2B), it was possible to determine and quantify four compounds (Table 2): oxalic (2.66 g per 100 g dw), citric (1.24 g per 100 g dw), fumaric (0.25 g per 100 g dw) and quinic (0.16 g per 100 g dw) acids. Oxalic and fumaric acids are known for their bioactivities, particularly antibacterial, antitumor and antifungal activities.32–34
Antioxidant molecules such as cinnamic (175.28 μg per 100 g) and p-hydroxybenzoic (30.71 μg per 100 g) acids (Fig. 2C) were also identified and quantified after methanolic extraction (Table 2).
In vitro antioxidant, antitumor and antimicrobial properties
Antioxidant activity was measured by four different methods by using methanolic and polysaccharidic extracts. These assays measured free radical scavenging activity, reducing power and lipid peroxidation inhibition. In three of the four assays, polysaccharidic extract exhibited higher activity (lower EC50 values; Table 3). Furthermore, for the TBARS assay, it was the methanolic extract that showed the highest activity (EC50 = 0.78 mg mL−1). The same behavior was previously reported for other mushroom species.35 The observed antioxidant activity may be the consequence of the presence of various antioxidant compounds described in the previous section such as tocopherols (mainly α-tocopherol), organic acids and phenolic compounds. Other authors previously reported high antioxidant activity of the L. sulphureus ethanolic and polysaccharidic extracts,36,37 and other studies report natural products as good antioxidant agents.38,39
Table 3
In vitro antioxidant potential of L. sulphureus (mean ± SD)a
EC50 values (mg mL−1) |
Methanolic extract |
Polysaccharidic extract |
Trolox |
The results are presented in EC50 values, which means that lower values correspond to higher reducing power or antioxidant potential. EC50, extract concentration corresponding to 50% of antioxidant activity or 0.5 of absorbance for the reducing power assay.
|
DPPH scavenging activity |
6.80 ± 0.18 |
2.18 ± 0.10 |
0.04 ± 0.00 |
Reducing power |
1.93 ± 0.02 |
0.72 ± 0.03 |
0.03 ± 0.00 |
β-Carotene bleaching inhibition |
3.56 ± 0.08 |
0.60 ± 0.02 |
0.003 ± 0.00 |
TBARS inhibition |
0.78 ± 0.07 |
4.35 ± 0.23 |
0.004 ± 0.00 |
The results obtained for antiproliferative activity against human tumor cell lines and non-tumor liver primary cells are presented in Table 4. The polysaccharidic extract was the only one that showed in vitro antitumor activity. Moreover, it did not show activity against HepG2 cell line. The most susceptible cell lines were HCT15 (GI50 = 68.96 μg mL−1) and HeLa (GI50 = 72.26 μg mL−1). None of the extracts was toxic for porcine liver cells (GI50 > 400 μg mL−1). Previous studies based on natural matrices were published recently reporting the antitumor activity of natural products.39,40
Table 4
In vitro antiproliferative activity of L. sulphureus against human tumor cell lines and non-tumor liver primary cells (mean ± SD)a
GI50values (μg mL−1) |
Methanolic extract |
Polysaccharidic extract |
Ellipticine |
GI50 values correspond to the sample concentration achieving 50% of growth inhibition in human tumor cell lines or in liver primary cultures, PLP2.
|
MCF-7 (breast carcinoma) |
>400 |
239.57 ± 2.44 |
0.91 ± 0.04 |
NCI–H460 (non-small-cell lung cancer) |
>400 |
181.49 ± 12.58 |
1.42 ± 0.00 |
HCT15 (colon carcinoma) |
>400 |
68.96 ± 6.00 |
1.91 ± 0.06 |
HeLa (cervical carcinoma) |
>400 |
72.26 ± 5.37 |
1.14 ± 0.21 |
HepG2 (hepatocellular carcinoma) |
>400 |
>400 |
3.22 ± 0.67 |
PLP2 (non-tumor liver primary cells) |
>400 |
>400 |
2.06 ± 0.03 |
The polysaccharidic extract also showed higher antibacterial activity than methanolic extract, except against M. flavus and S. typhimurium. The same was observed for antifungal properties, with the exception of P. ochrochloron (Table 5). Comparing antibacterial and antifungal activities of the tested extracts, it could be noted that the bacteria were more sensitive to both extracts than fungi. The higher sensitivity of bacteria could be attributed to the different cell organization between bacteria and microfungi (prokaryotic vs. eukaryotic cells). The antibacterial effect of L. sulphureus ethanolic extract was also previously reported by Turkoglu et al.36
Table 5 Antimicrobial activity of L. sulphureus (mean ± SD)
Bacteria |
Methanolic extract MIC (mg mL−1) MBC (mg mL−1) |
Polysaccharidic extract MIC (mg mL−1) MBC (mg mL−1) |
Ampicillin MIC (mg mL−1) MBC (mg mL−1) |
Streptomycin MIC (mg mL−1) MBC (mg mL−1) |
Micrococcus flavus
|
1.4 ± 0.06 |
3.1 ± 0.06 |
0.3 ± 0.03 |
0.2 ± 0.02 |
1.8 ± 0.10 |
6.2 ± 0.10 |
0.4 ± 0.00 |
0.3 ± 0.02 |
Staphylococcus aureus
|
1.8 ± 0.00 |
0.4 ± 0.00 |
0.3 ± 0.05 |
0.05 ± 0.00 |
3.6 ± 0.06 |
2.4 ± 0.06 |
0.4 ± 0.02 |
0.1 ± 0.00 |
Listeria monocytogenes
|
3.6 ± 0.00 |
3.1 ± 0.00 |
0.4 ± 0.01 |
0.2 ± 0.01 |
7.2 ± 0.10 |
6.2 ± 0.10 |
0.5 ± 0.03 |
0.3 ± 0.00 |
Escherichia coli
|
2.8 ± 0.03 |
1.5 ± 0.03 |
0.3 ± 0.05 |
0.2 ± 0.03 |
3.6 ± 0.00 |
3.1 ± 0.06 |
0.5 ± 0.05 |
0.3 ± 0.02 |
Pseudomonas aeruginosa
|
2.8 ± 0.03 |
1.5 ± 0.03 |
0.8 ± 0.02 |
0.2 ± 0.04 |
3.6 ± 0.06 |
3.1 ± 0.06 |
1.25 ± 0.00 |
0.3 ± 0.03 |
Salmonella typhimurium
|
0.9 ± 0.02 |
0.4 ± 0.00 |
0.3 ± 0.03 |
0.2 ± 0.00 |
1.8 ± 0.00 |
4.8 ± 0.20 |
0.4 ± 0.00 |
0.3 ± 0.01 |
Fungi |
Methanolic extract MIC (mg mL−1) MFC (mg mL−1) |
Polysaccharidic extract MIC (mg mL−1) MFC (mg mL−1) |
Bifonazole MIC (mg mL−1) MFC (mg mL−1) |
Ketoconazole MIC (mg mL−1) MFC (mg mL−1) |
Aspergillus fumigatus
|
2.5 ± 0.10 |
2.0 ± 0.30 |
0.15 ± 0.00 |
0.2 ± 0.00 |
9.0 ± 0.30 |
4.0 ± 0.20 |
0.20 ± 0.02 |
0.5 ± 0.01 |
Aspergillus versicolor
|
4.5 ± 0.10 |
1.0 ± 0.00 |
0.10 ± 0.007 |
0.2 ± 0.06 |
9.0 ± 0.30 |
4.0 ± 0.03 |
0.20 ± 0.008 |
0.5 ± 0.06 |
Aspergillus ochraceus
|
2.5 ± 0.00 |
0.5 ± 0.06 |
0.15 ± 0.02 |
1.5 ± 0.00 |
5.0 ± 0.10 |
4.0 ± 0.06 |
0.20 ± 0.00 |
2.0 ± 0.03 |
Trichoderma viride
|
3.75 ± 0.03 |
2.0 ± 0.00 |
0.10 ± 0.007 |
1.0 ± 0.00 |
5.0 ± 0.00 |
4.0 ± 0.10 |
0.20 ± 0.03 |
1.0 ± 0.03 |
Penicillium ochrochloron
|
1.25 ± 0.06 |
2.0 ± 0.03 |
0.20 ± 0.02 |
2.5 ± 0.20 |
2.5 ± 0.03 |
4.0 ± 0.10 |
0.25 ± 0.01 |
3.5 ± 0.10 |
Penicillium verrucosum
|
4.5 ± 0.06 |
4.0 ± 0.00 |
0.15 ± 0.00 |
0.2 ± 0.03 |
9.0 ± 0.20 |
8.0 ± 0.20 |
0.20 ± 0.02 |
0.3 ± 0.00 |
In situ food preserving properties
The data regarding in situ preserving properties of the L. sulphureus methanolic extract are presented in Tables 6. The contamination incidence (Table 6) was recorded, and the results indicate no contamination at concentrations of 3.56 mg per well or higher. It was obvious that the inhibition of A. flavus growth in pâté is dose dependent and that higher concentrations of L. sulphureus extract completely inhibited mycelial growth (Table 6). It was evident that the lower storage temperature slowed the mycelial growth rather than room temperature (approximately 21 °C), but refrigerated conditions were used because it is an appropriate way to store meat pâté. The first observations of the mycelial growth of A. flavus were registered in the control group (without the methanolic extract) after seven days in storage. In groups with the incorporated methanolic extract in various concentrations, it was noted that all of the tested concentrations successfully inhibited the growth of the food-poisoning mould during the first 14 days of storage. However, during the next seven days, only higher concentrations (3.56 mg per well, 4.75 mg per well and 5.94 mg per well) were sufficiently successful in preventing the micromycete growth. The observation of mycelial growth, spore germination and the measurement of the growth inhibition zones were discontinued after 21 days of storage. This decision was made due to the fact that, at the mentioned time, the control group was completely infected and overgrown with A. flavus. Therefore, it was determined that 21 days of storage were sufficient for A. flavus to fully grow and germinate under exposed conditions of storage. The highest doses used completely retarded the growth of A. flavus with an inhibition percentage of 100%.
Table 6 Contamination incidence of A. flavus in treated and control samples of chicken pâtéa
Mould |
Concentration (mg per well) |
Contamination incidence (%) |
Aspergillus flavus
|
0 |
100.0 |
1.18 |
66 |
2.38 |
60 |
3.56 |
0.0 |
4.75 |
0.0 |
5.94 |
0.0 |
Conc. |
Control |
1.18 mg |
2.38 mg |
3.56 mg |
4.75 mg |
5.49 mg |
Inhibition zones (mm; mean ± SD) of A. flavus in chicken pâté with the addition of L. sulphureus extract stored at +4 °C.
|
0 day |
0.00 |
0.00 |
0.00 |
0.00 |
0.00 |
0.00 |
7th day |
5.00 |
0.00 |
0.00 |
0.00 |
0.00 |
0.00 |
14th day |
11.00 |
0.00 |
0.00 |
0.00 |
0.00 |
0.00 |
21st day |
25.00 |
7.35 |
4.50 |
0.00 |
0.00 |
0.00 |
Meat products are widely consumed among humans and, therefore, the question of their toxicity is attracting considerable attention. The reason for this is the knowledge that moulds, which are widely present in meat products, can produce secondary metabolites, or mycotoxins, that are not subjected to heat treatment and are implemented throughout the food chain without any chemical change. Mycotoxins are known for their unfavorable effects: carcinogenesis, mutagenicity and high thermostability.41A. flavus is among the most common meat product pathogens, particularly in those which have ripened for a length of time. However, this number goes as high as 78 species of moulds, which have been isolated from various meat products. Moreover, it should be noted that the presence of mould alone does not mean the presence of mycotoxins. According to Mižáková et al.,41 several conditions have to be satisfied for moulds to produce mycotoxins, such as the presence of oxygen, temperatures between 4 °C and 40 °C, pH values between 2.5 and 8, minimum water activity of 0.80 and a maximum salt concentration of 14%. Spores of moulds are everywhere around us, and they enable the moulds to survive extreme conditions and wait for the favorable conditions to fully develop. This is the reason why moulds are practically impossible to eliminate from products even when maintaining a very high level of hygiene during the production and storage. The prevention of mould development is highly recommendable at all stages of meat production, from the slaughtering of animals to the packaging of the final product.41
Because consumers have greater awareness and higher concern regarding synthetic chemical additives, food products preserved with natural additives are becoming more popular.42 Because of that, we have chosen a mushroom with proven antimicrobial and antioxidant properties that could bring functional preservations methods to meat products, affecting their taste in a positive way and making them acceptable to the customer. In any case, when choosing a natural antioxidant that will be used for purposes of prolonging the shelf life of food, the impact on the sensory and taste qualities should also be taken into account to obtain a product with the desired properties.
Regarding preservation of meat pâté with natural substances, another study was carried out by Hayrapetyan et al.43 using pomegranate extract as an inhibitor of bacterial growth. This extract turned out to be an excellent food product preservative. Moreover, we think that mushrooms are also good candidates due to their exquisite taste.
Conclusion
Because of their medicinal properties, mushrooms traditionally could be used with great potential for therapeutic applications in the treatment of some diseases. In this study, we showed that mushrooms also could be a great source of natural bioactive compounds, including antioxidants, such as polyphenols, vitamins, unsaturated fatty acids and sugars, which can be useful for various applications, particularly as food additives and for promotion as healthy ingredients in the formulations of functional foods and nutraceuticals.
Conflict of interest
The authors declare no conflict of interest. This article does not contain any studies with human or animal subjects.
Acknowledgements
The authors are grateful to Foundation for Science and Technology (FCT, Portugal) and COMPETE/QREN/EU for financial support to this work (research project PTDC/AGR-ALI/110062/2009 and L. Barros researcher contract under “Programa Compromisso com Ciência-2008”) and to CIMO (strategic project PEst-OE/AGR/UI0690/2011). The authors also thank the Serbian Ministry of Education and Science for financial support (grant number 173032) and Mr Dragoljub Ratkovic for providing us with fresh material of the mushroom Laetiporus sulphureus.
References
- P. Kalač, A review of chemical composition and nutritional value of wild-growing and cultivated mushrooms, J. Sci. Food Agric., 2013, 93, 209–218 CrossRef PubMed.
- M. Carocho and I. C. F. R. Ferreira, A review on antioxidants, prooxidants and related controversy: Natural and synthetic compounds, screening and analysis methodologies and future perspectives, Food Chem. Toxicol., 2013, 51, 15–25 CrossRef CAS PubMed.
- I. C. F. R. Ferreira, L. Barros and R. M. V. Abreu, Antioxidants in wild mushrooms, Curr. Med. Chem., 2009, 16, 1543–1560 CrossRef CAS.
- U. Lindequist, J. H. Timo and W. D. Julich, The pharmacological potential of mushrooms, Evidence-Based Complementary and Alternative Medicine, 2005, 2, 285–299 CrossRef PubMed.
- M. D. Mignogna, S. Fedele and L. Lo Russo, The World Cancer Report and the burden of oral cancer, Eur. J. Cancer Prev., 2004, 13, 139–142 CrossRef CAS PubMed.
- V. Popović, J. Živković, S. Davidović, M. Stevanović and D. Stojković, Mycotherapy of cancer: an update on cytotoxic and antitumor activities of mushrooms, bioactive principles and molecular mechanisms of their action, Curr. Top. Med. Chem., 2013, 13, 2791–2806 CrossRef.
- J. Petrović, J. Glamočlija, D. S. Stojković, A. Ćirić, M. Nikolić, D. Bukvički, M. E. Guerzoni and M. D. Soković,
Laetiporus sulphureus, edible mushroom from Serbia: Investigation on volatile compounds, in vitro antimicrobial activity and in situ control of A. flavus in tomato paste, Food Chem. Toxicol., 2013, 59, 297–302 CrossRef PubMed.
- F. S. Reis, D. Stojković, M. Soković, J. Glamočlija, A. Cirić, L. Barros and I. C. F. R. Ferreira, Chemical characterization of Agaricus bohusii, antioxidant potential and antifungal preserving properties when incorporated in cream cheese, Food Res. Int., 2012, 48, 620–626 CrossRef CAS PubMed.
- D. Stojković, F. S. Reis, I. C. F. R. Ferreira, L. Barros, J. Glamočlija, A. Ćirić, M. Nikolić, T. Stević, A. Giweli and M. Soković,
Tirmania pinoyi: Chemical composition, in vitro antioxidant and antibacterial activities and in situ control of Staphylococcus aureus in chicken soup, Food Res. Int., 2013, 53, 56–62 CrossRef PubMed.
- M. K. Fasseas, K. C. Mountzouris, P. A. Tarantilis, M. Polissiou and G. Zervas, Antioxidant activity in meat treated with oregano and sage essential oils, Food Chem., 2007, 106, 1188–1194 CrossRef PubMed.
- V. Velasco and P. Williams, Improving meat quality through natural antioxidants, Chil. J. Agric. Res., 2011, 71, 313–322 CrossRef.
- D. Dave and A. E. Ghaly, Meat Spoilage Mechanisms and Preservation Techniques: A Critical Review, Am. J. Agric. Biol. Sci., 2011, 6, 486–510 CrossRef CAS.
- D. N. Olennikov, S. V. Agafonova, G. B. Borovskii, T. A. Penzina and A. V. Rokhin, Water-soluble endopolysaccharides from the fruiting bodies of L. sulphureus (Bull.:Fr.) Murr., Appl. Biochem. Microbiol., 2009, 45, 536–543 CrossRef CAS.
-
AOAC, Official methods of analysis, Association of Official Analytical Chemists, Arlington VA, USA, 16th edn, 1995 Search PubMed.
- S. A. Heleno, L. Barros, M. J. Sousa, A. Martins and I. C. F. R. Ferreira, Study and characterization of selected nutrients in wild mushrooms from Portugal by gas chromatography and high performance liquid chromatography, Microchem. J., 2009, 93, 195–199 CrossRef CAS PubMed.
- S. A. Heleno, L. Barros, M. J. Sousa, A. Martins and I. C. F. R. Ferreira, Tocopherols composition of Portuguese wild mushrooms with antioxidant capacity, Food Chem., 2010, 119, 1443–1450 CrossRef CAS PubMed.
- L. Barros, C. Pereira and I. C. F. R. Ferreira, Optimized analysis of organic acids in edible mushrooms from Portugal by ultra-fast liquid chromatography and photodiode array detection, Food Analytical Methods, 2013, 6, 309–316 CrossRef.
- L. Barros, M. Dueñas, I. C. F. R. Ferreira, P. Baptista and C. Santos-Buelga, Phenolic acids determination by HPLC-DAD-ESI/MS in sixteen different Portuguese wild mushrooms species, Food Chem. Toxicol., 2009, 47, 1076–1107 CrossRef CAS PubMed.
- A. J. Vaz, S. A. Heleno, A. Martins, M. G. Almeida, M. H. Vasconcelos and I. C. F. R. Ferreira, Wild mushrooms Clitocybe alexandri and Lepista inversa: In vitro antioxidant activity and growth inhibition of human tumour cell lines, Food Chem. Toxicol., 2010, 48, 2881–2884 CrossRef PubMed.
- F. S. Reis, S. A. Heleno, L. Barros, M. J. Sousa, A. Martins, C. Santos-Buelga and I. C. F. R. Ferreira, Toward the antioxidant and chemical characterization of mycorrhizal mushrooms from northeast Portugal, J. Food Sci., 2011, 76, 824–830 CrossRef PubMed.
- A. Monks, D. Scudiero, P. Skehan, R. Shoemaker, K. Paull, D. Vistica, C. Hose, J. Langley, P. Cronise and A. Vaigro-Wolff, Feasibility of a high-flux anticancer drug screen using a diverse panel of cultured human tumor cell lines, J. Natl. Cancer Inst., 1991, 83, 757–766 CrossRef CAS PubMed.
- R. M. V. Abreu, I. C. F. R. Ferreira, R. C. Calhelha, R. T. Lima, M. H. Vasconcelos, F. Adega, R. Chaves and M. J. R. P. Queiroz, Anti-hepatocellular carcinoma activity using human HepG2 cells and hepatotoxicity of 6-substituted methyl 3-aminothieno[3,2-b]pyridine-2-carboxylate derivatives: In vitro evaluation, cell cycle analysis and QSAR studies, Eur. J. Med. Chem., 2011, 46, 5800–5806 CrossRef CAS PubMed.
-
CLSI – Clinical and Laboratory Standards Institute, Methods for dilution antimicrobial susceptibility tests for bacteria that grow aerobically. Approved standard, CLSI publication M07-A8, Clinical and Laboratory Standards Institute, Wayne, PA, 8th edn, 2009 Search PubMed.
- T. Tsukatani, H. Suenaga, M. Shiga, K. Noguchi, M. Ishiyama, T. Ezoe and K. Matsumoto, Comparison of the WST-8 colorimetric method and the CLSI broth microdilution method for susceptibility testing against drug-resistant bacteria, J. Microbiol. Methods, 2012, 90, 160–166 CrossRef CAS PubMed.
-
C. Booth, Fungal culture media, in Methods in microbiology, ed. J. R. Norris and D. W. Ribbons, Academic Press, London and New York, 1971, pp. 49–94 Search PubMed.
- A. Espinel-Ingroff, Comparation of the E-test with the NCCLS M38-P method for antifungal susceptibility testing of common and emerging pathogenic filamentous fungi, J. Clin. Microbiol., 2001, 39, 1360–1367 CrossRef CAS PubMed.
- G. Mtui and R. Masalu, Extracellular enzymes from brown-rot fungus Laetiporus sulphureus isolated from mangrove forests of coastal Tanzania, Sci. Res. Essays, 2008, 3, 154–161 Search PubMed.
- R. W. S. Weber, A. Mucci and P. Davoli, Laetiporic acid, a new polyene pigment from the wood-rotting basidiomycete L. sulphureus (Polyporales, fungi), Tetrahedron Lett., 2003, 45, 1075–1078 CrossRef PubMed.
- A. M. Mshandete and J. Cuff, Proximate and nutrient composition of three types of indigenous edible wild mushrooms grown in Tanzania and their utilization prospects, Afr. J. Food, Agric., Nutr. Dev., 2007, 7, 1–6 Search PubMed.
- E. Bernas, G. Jaworska and Z. Lisiewska, Edible mushrooms as a source of valuable nutritive constituents, Acta Sci. Pol., Technol. Aliment., 2006, 5, 5–20 CAS.
- N. Yilmaz, M. Solmaz, T. Ibrahim and M. Elmastas, Fatty acid composition in some wild edible mushrooms growing in the middle Black Sea region of Turkey, Food Chem., 2006, 99, 168–174 CrossRef CAS PubMed.
- M. J. Alves, I. C. F. R. Ferreira, J. Dias, V. Teixeira, A. Martins and M. Pintado, A review on antimicrobial activity of mushroom (Basidiomycetes) extracts and isolated compounds, Planta Med., 2012, 78, 1707–1718 CrossRef CAS PubMed.
- M. Akao and K. Kuroda, Antitumor and anti-intoxication activities of fumaric acid in cultured cells, Gann, 1981, 72, 777–782 Search PubMed.
- M. Akao and K. Kuroda, Antifungal activity of fumaric acid in mice infected with Candida albicans, Chem. Pharm. Bull., 1991, 39, 3077–3078 CrossRef CAS.
- V. Vieira, A. Marques, L. Barros, J. C. M. Barreira and I. C. F. R. Ferreira, Insights in the antioxidant synergistic effects of combined edible mushrooms: phenolic and polysaccharidic extracts of Boletus edulis and Marasmius oreades, J. Food Nutr. Res., 2012, 51, 109–116 CAS.
- A. Turkoglu, M. E. Duru, N. Mercan, I. Kivrak and K. Gezer, Antioxidant and antimicrobial activities of Laetiporus sulphureus (Bull.) Murill, Food Chem., 2007, 101, 267–273 CrossRef CAS PubMed.
-
A. Klaus, Chemical characterisation, antimicrobial and antioxidative characteristics of lignicolic polysacharides from Ganoderma spp., Laetiporus sulphureus and Schizophyllum commune. PhD thesis, Faculty of Agriculture, University of Belgrade, 2011.
- A. Gismondi, M. Serio, L. Canuti and A. Canini, Biochemical, antioxidant and antineoplastic properties of Italian saffron (Crocus sativus L.), Am. J. Plant Sci., 2012, 3, 1573–1580 CrossRef.
- A. Gismondi, L. Canuti, M. Grispo and A. Canini, Biochemical composition and antioxidant properties of Lavandula angustifolia Miller essential oil are shielded by propolis against UV radiations, Photochem. Photobiol., 2014 DOI:10.1111/php.12229.
- A. Gismondi, L. Canuti, S. Impei, G. Di Marco, M. Kenzo, V. Colizzi and A. Canini, Antioxidant extracts of African medicinal plants induce cell cycle arrest and differentiation in B16F10 melanoma cells, Int. J. Oncol., 2013, 43, 956–964 Search PubMed.
- A. Mižáková, M. Pipová and P. Turek, The Occurrence of Moulds in Fermented Raw Meat Products, Czech J. Food Sci., 2002, 20, 89–94 Search PubMed.
- R. A. Hooley and D. Patel, Improvement in shelf-life and safety of perishable foods by plant essential oils and smoke antimicrobials, Food Microbiol., 2005, 22, 273–292 CrossRef PubMed.
- H. Hayrapetyan, W. Hazeleger and R. Beumer, Inhibition of Listeria monocytogenes by pomegranate (Punicagranatum) peel extract in meat paté at different temperatures, Food Control, 2012, 23, 66–72 CrossRef PubMed.
|
This journal is © The Royal Society of Chemistry 2014 |
Click here to see how this site uses Cookies. View our privacy policy here.