DOI:
10.1039/C4FO00100A
(Review Article)
Food Funct., 2014,
5, 1320-1333
Excipient foods: designing food matrices that improve the oral bioavailability of pharmaceuticals and nutraceuticals
Received
11th February 2014
, Accepted 10th April 2014
First published on 24th April 2014
Abstract
The oral bioavailability of many lipophilic bioactive agents (pharmaceuticals and nutraceuticals) is limited due to various physicochemical and physiological processes: poor release from food or drug matrices; low solubility in gastrointestinal fluids; metabolism or chemical transformation within the gastrointestinal tract; low epithelium cell permeability. The bioavailability of these agents can be improved by specifically designing food matrices that control their release, solubilization, transport, metabolism, and absorption within the gastrointestinal tract. This article discusses the impact of food composition and structure on oral bioavailability, and how this knowledge can be used to design excipient foods for improving the oral bioavailability of lipophilic bioactives. Excipient foods contain ingredients or structures that may have no bioactivity themselves, but that are able to promote the bioactivity of co-ingested bioactives. These bioactives may be lipophilic drugs in pharmaceutical preparations (such as capsules, pills, or syrups) or nutraceuticals present within food matrices (such as natural or processed foods and beverages).
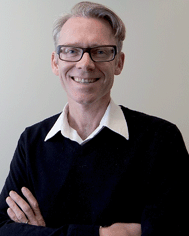 David Julian McClements | David Julian McClements is a Professor at the Department of Food Science at the University of Massachusetts, and adjunct Professor at the Department of Biochemistry at King Abdulaziz University in Saudi Arabia. He specializes in the areas of food biopolymers and colloids, and especially in the development of food-based structured delivery systems for bioactive components. Dr McClements is sole author of “Nanoparticle- and Microparticle-based Delivery Systems: Encapsulation, Protection and Release of Active Components” and “Food Emulsions: Principles, Practice and Techniques” and co-author or editor of numerous other books. He has published over 500 scientific articles in peer-reviewed journals (with an H-index of 62). Dr McClements has received awards from the American Chemical Society, American Oil Chemists Society, Society of Chemical Industry (U.K.), Institute of Food Technologists, and University of Massachusetts in recognition of his achievements. |
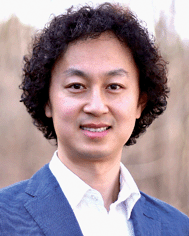 Hang Xiao | Hang Xiao is an Associate Professor at the Department of Food Science at the University of Massachusetts. His research is focused on biological activities and molecular mechanisms of bioactive food components in health promotion and disease prevention. He has published more than 70 manuscripts in peer-reviewed journals. Dr Xiao has received multiple research awards such as Institute of Food Technologist (IFT) Samuel Cate Prescott award, American Society for Nutrition (ASN) Mary Swartz Rose Investigator Award, and International Life Sciences Institute (ILSI) North America Future Leader Award. |
1. Introduction
Many bioactive agents present in foods (nutraceuticals) or drugs (pharmaceuticals) intended for oral ingestion are highly lipophilic molecules with low and/or variable bioavailability.1–6 The poor bioavailability characteristics of these bioactive agents may be the result of a number of physicochemical or physiological processes: restricted release from the product matrix;7 low solubility in gastrointestinal fluids;2,8 low permeability across intestinal epithelial cells;6,9 and/or, enzymatic or chemical transformations within the gastrointestinal tract (GIT).3,10,11 Research in the food, nutrition, and pharmaceutical disciplines has established that the bioavailability of many bioactive agents depends strongly on the nature of the foods ingested with them.2,3,5,8,12–16 Both the composition and the structural organization of the food matrix may influence the bioavailability of co-ingested bioactive agents.17 The dependence of the oral bioavailability of lipophilic bioactive agents on food matrix characteristics means there is considerable opportunity for designing food-based delivery systems to improve the efficacy of lipophilic pharmaceuticals and nutraceuticals.
2. Medical, functional and excipient foods
There is increasing convergence in the interests of the pharmaceutical and food industries in the development of products to prevent or treat human diseases (Fig. 1). The pharmaceutical industry is developing drug preparations to combat chronic or acute diseases, whereas the food industry is developing food and beverage products whose purpose is to promote human health and wellbeing through diet. In particular, there is a considerable overlap in the development of food-based approaches to improve the bioavailability of lipophilic bioactive agents, such as nutraceuticals and pharmaceuticals. These approaches are based on the design of the composition or structure of food matrices to increase bioavailability and have led to new classes of foods: functional foods; medical foods; and, excipient foods (Fig. 2).
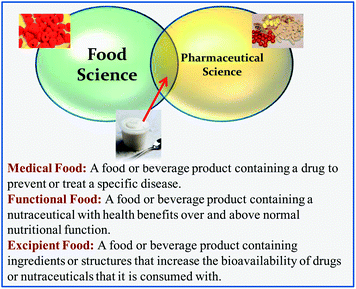 |
| Fig. 1 There is increasing convergence between the interests of the food and pharmaceutical industries in the development of products to prevent or treat diseases, particularly in the area of functional, medical, and excipient foods. | |
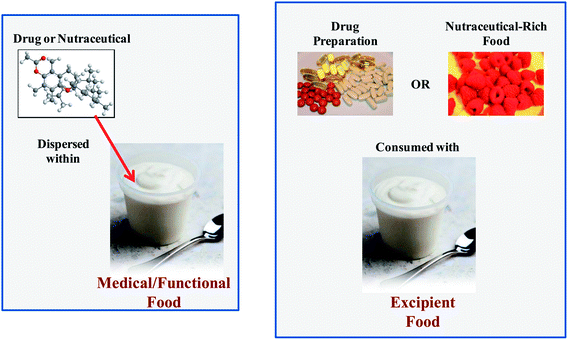 |
| Fig. 2 Schematic diagram of the difference between functional, medical, and excipient foods. The lipophilic bioactive component (pharmaceutical or nutraceutical) is usually encapsulated within the food matrix in medical and functional foods, but it is co-ingested with a different food matrix for excipient foods. | |
2.1. Functional foods
A functional food is fabricated from generally recognized as safe (GRAS) food ingredients, and typically contains one or more food-grade bioactive agents (“nutraceuticals”) dispersed within a food matrix. There are already many examples of functional food products that are commercially available, including milks fortified with vitamin D, yogurts fortified with probiotics, spreads fortified with phytosterols, and breakfast cereals fortified with ω-3 fatty acids, vitamins, and minerals. A great deal of research is currently being carried out on identifying other kinds of nutraceuticals, and it will be important for the food industry to clearly demonstrate their health benefits before they can be successfully incorporated into functional food products and obtain regulatory and consumer acceptance.
2.2. Medical foods
A medical food contains one or more pharmaceutical-grade bioactive agents (drugs) dispersed within a food matrix. This food matrix may be a traditional food type (such as a beverage, yogurt, or confectionary) or it may be a nutritional fluid that is fed to a patient through a tube. A medical food is usually administered to treat a particular disease under medical supervision. A number of medical foods are commercially available that are specifically designed to manage or treat various diseases, such as Alzheimer's, diarrhea, depression, diabetes, and osteoporosis.
2.3. Excipient foods
In this article, a new class of foods designed to improve the bioavailability of orally administered bioactive agents is introduced: excipient foods. An excipient is conventionally defined as a component that is not bioactive itself but is included in a pharmaceutical preparation to increase the efficacy of a drug.2,18–20 Some commonly used excipients in the pharmaceutical industry include lipids, surfactants, synthetic polymers, carbohydrates, proteins, cosolvents, and salts. By analogy, an excipient food may not have any bioactivity itself, but it may increase the efficacy of any nutraceuticals or pharmaceuticals that are co-ingested with it. Excipient foods are therefore meant to be consumed with a conventional pharmaceutical dosage form (e.g., capsule, pill, or syrup), a dietary supplement (e.g., capsule, pill, or syrup), or nutraceutical-rich food (e.g., fruits, vegetables, nuts, seeds, grains, meat, fish, and some processed foods). It is likely that different kinds of excipient foods will have to be designed for different types of bioactive agents or delivery matrices. Some examples of excipient foods that could be developed to increase the bioavailability of nutraceuticals in foods are shown in Table 1. For example, the bioaccessibility of carotenoids in a salad may be increased by consuming it with a specifically designed salad dressing. This dressing may contain various food components that increase the bioavailability of the nutraceuticals in the salad: lipids that increase intestinal solubility; antioxidants that inhibit chemical transformations; enzyme inhibitors that retard metabolism; permeation enhancers that increase absorption; efflux inhibitors. Indeed, previous studies have shown that the bioavailability of oil-soluble vitamins and carotenoids in salads can be increased by consuming them with dressings containing some fat,14 which supports the concept of excipient foods.
Table 1 Examples of excipient foods that could be designed to improve the bioactivity of nutraceuticals in foods
Nutraceuticals |
Food source |
Potential excipient foods |
Carotenoids |
Salad (lettuce, kale, carrot, tomato, peppers, …) |
Salad dressing |
Carotenoids |
Cooked vegetables (carrot, peppers, spinach, kale, …) |
Sauce |
Carotenoids, vitamins, phytosterols/stanols |
Nuts and seeds (almonds, peanuts, sunflower seeds, …) |
Edible coatings |
Flavonoids, vitamins |
Fruits and berries (blueberry, strawberry, raspberry, apple, pear, …) |
Cream, ice cream, yogurt |
Phytosterols/stanols |
Nuts |
Sauce, edible coatings |
CLA |
Meat and dairy products (beef, cheese, …) |
Sauce |
ω-3 oils |
Fish |
Sauce |
In principle, a wide variety of different food products could be used as excipients to increase the bioactivity of lipophilic bioactives, such as beverages, yogurts, dressings, desserts, sauces, soups, dips, spreads, candies, and baked goods. These excipient foods need to be selected so that they are economic, convenient, desirable, and effective, and that can be regularly incorporated into a daily diet.
In the remainder of this review, we initially consider the design of excipient foods, then we highlight the main factors limiting the bioavailability of lipophilic bioactive components, and then we discuss the impact of food matrix composition and structure on bioavailability and how this leads to the concept of excipient foods and excipient food ingredients.
3. Design of excipient foods
Excipient foods may be fluids, semi-solids, or solids that may be consumed by drinking (beverages) or eating (foods). A number of different factors must be considered when designing excipient foods. First, the composition and structure of the food matrix should be designed to increase the bioavailability of co-ingested bioactive agents. This depends on knowledge of the influence of specific food components and structures on the biological fate of the bioactives. Second, the food matrix should be designed so that the product is desirable to consumers or patients to ensure good compliance, e.g., the food should have a desirable appearance, texture, mouthfeel, and flavor.21 Third, foods or beverages should be chosen so that they can be consumed on a regular basis with drugs or foods containing nutraceutical agents (such as fruits and vegetables). This restricts the type of products suitable for use as excipient foods to those that can easily be incorporated into a daily diet. Fourth, the product should have a sufficiently long shelf life and not take up too much storage space, since it is impractical for consumers to purchase a product too frequently. Some potential candidates for excipient foods that meet most or all of these requirements are fruit drinks, teas, coffees, dairy beverages, creams, yogurts, margarine, butter, cheese spreads, desserts, confectionary, and crackers. The nature of the excipient food might depend on the type of drug or nutraceutical-rich food that is being consumed. For example, an excipient food suitable for increasing the bioavailability of the nutraceuticals in fruits (such as apples, pears, blueberries, strawberries, or raspberries) might consist of a specially designed cream, yogurt, or ice cream. On the other hand, an excipient food suitable for increasing the bioavailability of nutraceuticals in cooked or raw vegetables (such as carrots, broccoli, spinach, or kale) might consist of a specially designed pouring sauce or salad dressing.
3.1. Potential benefits of excipient foods
There are several potential benefits of developing excipient foods to increase the bioavailability of nutraceuticals and drugs. The long-term consumption of low levels of nutraceuticals may improve human performance, enhance wellbeing, or inhibit the onset of chronic diseases, such as heart disease, diabetes, hypertension, and cancer.5 This would increase the quality of life of the general population and reduce the costs of health care associated with treatment of these chronic diseases. At present, the bioavailability of the nutraceuticals in many natural sources, such as fruits and vegetables, is relatively low, and therefore their potential benefits on long-term human health are not being fully realized.3 In addition, it is well established that the oral bioavailability of many lipophilic drugs is relatively low and variable, which reduces their efficacy and can lead to undesirable side effects.2,22 The development of specially designed excipient foods that enhance bioavailability and bioactivity may be able to overcome these problems.
3.2. Limitations of excipient foods
The development of successful excipient foods faces a number of technical, legal, and commercial challenges. In particular, there are important differences in the ability to prove the impact of excipient foods on the bioactivity of nutraceuticals. Drugs can be administered in well-defined doses at specified times thereby enabling pharmaceutical researchers to carry out studies to establish their efficacy against specific disease symptoms or biomarkers. Thus the impact of excipient foods on drug bioactivity can be established using well-controlled experiments that involve taking the drug in the absence or presence of the excipient food. In contrast, nutraceuticals are typically consumed at relatively low levels as part of a complex diet over extended periods. Hence, it is often difficult to establish a strong correlation between the type and amount of nutraceutical consumed and a particular disease. This would make it challenging to prove the efficacy of excipient foods at improving human health and wellness since long-term studies would be needed with well-controlled diets. Consequently, it would be difficult for food manufacturers to provide the scientific evidence required by regulators to make specific health claims about an excipient food product in their advertising or labeling. In the absence of this kind of competitive advantage food companies may be reluctant to spend research funds on developing and testing the efficacy of excipient foods. Nevertheless, one might be able to make the simpler claim that excipient foods increase the bioavailability of specific food components, such as carotenoids or oil-soluble vitamins. Another unique challenge that the food industry faces is in controlling the dose and timing that a nutraceutical containing food and an excipient food are consumed. Pharmaceuticals are usually taken in well-defined doses at specific times, whereas nutraceutical agents may be present in various types of foods that are consumed in different amounts by different individuals as part of a complex diet that contains other components that could affect bioavailability. The time that an excipient food is consumed relative to a nutraceutical-rich food may also be important for the efficacy in enhancing bioavailability, e.g., before, during, or after consumption.
Another potential challenge is that an individual may consume a number of different kinds of foods containing nutraceuticals, or a patient may need to take more than one kind of drug per day. It may be necessary to design different kinds of food matrices in excipient foods for different kinds of nutraceutical-rich foods or drugs. In addition, different individuals or patients have different food preferences and so a range of different kinds of excipient product types may be required, e.g., fruit drinks, yogurts, candies, deserts, and spreads with different flavors.
Another potential issue with the development of excipient foods is their potential adverse side effects on human health. For example, the metabolizing enzymes and efflux transporters in epithelial cells usually protect the human body from the effects of any harmful substances that have been ingested.23 If bioactive agents are incorporated into foods that appreciably alter these mechanisms, then they might increase the uptake of harmful substances that could have adverse effects on health and wellness. For example, some excipient food ingredients could increase the bioavailability of toxic substances found in foods. In addition, certain bioactive components may be beneficial to human health in relatively low doses, but have adverse effects at relatively high levels. In this case, the ability of an excipient food to greatly enhance the bioavailability of a bioactive component could be detrimental.
Finally, if excipient foods are going to be marketed to consumers, it will be important to educate them about their potential risk and benefits, and to provide advice about which excipient food should be consumed with which nutraceutical-rich food. For example, a dessert cream may be marketed as an excipient food to be consumed with berries, whereas a salad dressing may be marketed as an excipient food to be consumed with salads and vegetables (Table 1).
4. Bioavailability of lipophilic bioactive agents
It is useful to highlight the major factors limiting the bioavailability of lipophilic bioactive agents since this information will aid in the successful development of efficacious excipient foods. The oral bioavailability of an ingested bioactive component depends on the fraction that reaches the target site-of-action in a biologically active form.5 The overall bioavailability (F) of a lipophilic bioactive component depends on numerous factors (Fig. 3):24,25 | F = FL × FA × FD × FM × FE | (1) |
FL is the fraction of bioactive agent liberated from its original environment, which may be a drug preparation or a food matrix, into the GIT so that it becomes bioaccessible i.e., in a form suitable for absorption (FL). FA is that fraction of the liberated bioactive agent that is absorbed by the epithelial cells within the GIT. FD is the fraction of absorbed bioactive agent that reaches the site of action after distribution amongst the various tissues of the body e.g., blood, liver, kidney, heart, brain, muscles, adipose tissue etc. FM is the fraction of bioactive component that reaches the site of action in a metabolically active form, which depends on any chemical or enzymatic transformations that take place after ingestion e.g., hydrolysis, oxidation, and conjugation. FE is the fraction of metabolically active bioactive component that remains at the site of action, i.e., has not been excreted. In reality, each of these parameters varies over time after a bioactive agent has been ingested to give a profile of bioavailability (F) versus time (t) at a specified site of action. Typically, the overall bioavailability increases sometime after ingestion, and then decreases as the bioactive agent is metabolized, stored, utilized, distributed, or excreted. Ultimately, the bioactivity of an ingested bioactive component depends on how its bioavailability changes over time in the target tissue. A number of physiological and physicochemical factors that influence the bioavailability of lipophilic bioactive components have been established,26–28 and are summarized in the following sections.
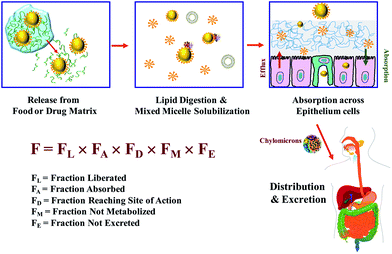 |
| Fig. 3 The oral bioavailability of a lipophilic bioactive agent depends on various liberation, absorption, distribution, metabolism, and excretion processes. Some of the key processes involved are shown schematically. | |
4.1. Liberation
A lipophilic bioactive agent must be liberated from a food matrix (e.g., fruit, vegetable, fish, meat, processed food) or drug preparation (e.g., pill or capsule) and then solubilized within mixed micelles in the small intestinal fluids before it becomes accessible for absorption (Fig. 3). Mixed micelles are assembled from bile salts and phospholipids secreted by the body, as well as any lipid digestion products such as monoacylglycerols and free fatty acids. It should be stressed that the expression “mixed micelles” actually refers to a compositionally, structurally, and dynamically complex mixture within the GIT that may contain various colloidal structures, such as micelles, vesicles, and liquid crystals that changes over time during the digestion and absorption processes.29 The fraction of an ingested lipophilic bioactive agent that is solubilized within the mixed micelle phase of the small intestine is usually taken to be a measure of the fraction that is liberated (FL) in a form suitable for absorption.
4.2. Absorption
Mixed micelles are able to transport solubilized lipophilic bioactive agents through the mucus layer and to the apical side of the intestinal epithelial cells (Fig. 3). The bioactives may then be incorporated into the epithelial cells through various passive or active transfer mechanisms.30 At present, it is not clear whether the bioactive agents are first released from the mixed micelles into the surrounding aqueous phase and then absorbed, or whether they are absorbed as part of the mixed micelles e.g., by fusion with the cell membranes. In addition, it is also possible for bioactive molecules trapped within other types of colloidal particles (such as engineered nanoparticles) to be directly absorbed by intestinal epithelial cells. Overall, the fraction of the bioactive agent that is transported into the epithelial cells is usually taken as a measure of the fraction absorbed (FA) by the body.
4.3. Metabolism
After ingestion, lipophilic bioactive agents may be transformed as they pass through the GIT or after they have been absorbed due to various chemical processes (such as acid hydrolysis or lipid oxidation)31 or biochemical processes (such as digestive or metabolic enzyme activity).2,5,23,32 The presence of digestive enzymes (such as lipases and phospholipases) may catalyze the breakdown of some lipophilic bioactive agents (such as triacylglycerols, phospholipids or vitamin E acetate).33 The presence of metabolic enzymes changes the chemical structures of some ingested lipophilic bioactive agents, thereby altering their physicochemical and physiological characteristics. The extent of metabolism often depends on the route that the bioactive agents are transported into the systemic circulation.22,34 Strongly hydrophobic agents tend to be transported via the lymphatic route, whereas less hydrophobic agents tend to be transported via the portal vein and liver.33 Lipophilic bioactives may be highly metabolized when they pass through the liver before reaching the systemic circulation, thereby altering their biological activity. In some cases, molecular transformations increase bioactivity, whereas in other cases they decrease it. The transformation of a lipophilic bioactive as it travels through the GIT and human body determine the fraction that arrives at the site of action in a metabolically active state (FM). The ability to alter the absorption pathway of bioactive agents by manipulating dietary composition or structure provides an important way of increasing the bioavailability of certain bioactives.
4.4. Distribution
After a lipophilic bioactive agent has been absorbed it is usually distributed amongst various tissues within the human body (Fig. 3), such as the systemic circulation, liver, kidney, muscles, adipose tissue, heart, lungs, brain, etc.35 The distribution of the bioactive agent depends on the molecular characteristics of the bioactive, as well as those of any co-ingested food components. The target tissue(s) for a bioactive agent depends on the nature of the biological response required, such as enhanced performance, maintenance of general wellbeing, prevention of chronic disease, or treatment of specific acute diseases.
4.5. Excretion
Lipophilic bioactives and there metabolites are eventually removed from the human body through a variety of mechanisms, and often end up within the feces, urine, sweat, or breath.36 It may therefore be possible to increase the bioavailability of an ingested bioactive by increasing its persistence within the human body. The rate of excretion determines the fraction of bioactive agent that remains at the site of action (FE) at a particular time.
4.6. Improving oral bioavailability
The oral bioavailability of ingested lipophilic agents can be improved by designing excipient foods that increase the fraction liberated (FL), absorbed (FA), and reaching the site of action (FD) in a metabolically active form (FM). This goal can be achieved by manipulating the composition and structure of food matrices based on knowledge of the impact of specific food matrix properties on the biological fate of ingested lipophilic bioactives (see Section 5).
5. Impact of food matrix on bioavailability
The oral bioavailability of lipophilic bioactives in drugs or foods may be increased by ingesting them with excipient foods with specifically designed compositions and structures. In this section, some of the major ways in which food components may alter the oral bioavailability of lipophilic bioactive agents is highlighted. It is assumed that an excipient food should be fabricated entirely from food-grade ingredients that are generally recognized as safe (GRAS). An excipient food could then be marketed and distributed as a conventional food product with additional health benefits.
5.1. Potential mechanisms of action
The components within an excipient food may alter the oral bioavailability of co-ingested lipophilic bioactives through various physicochemical or biochemical mechanisms, which are highlighted in this section.
5.1.1. Bioactive liberation.
Prior to ingestion, lipophilic bioactive agents are typically trapped within some kind of fluid, semi-solid, or solid matrix in pharmaceutical or drug products. For example, a lipophilic drug may be present within a pill or capsule, whereas a lipophilic nutraceutical may be trapped inside the cells of a fruit or vegetable or within the fat droplets in a processed food. The bioactive agents must therefore be liberated from their original location before they can be solubilized within intestinal fluids and absorbed by the body (Fig. 3). An excipient food may therefore be designed so that it contains specific ingredients that facilitate the release and solubilization of bioactive agents. The design of this type of food requires knowledge of the physicochemical and physiological processes that occur within the human gastrointestinal tract after ingestion (Fig. 4).
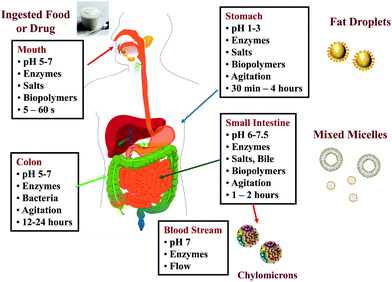 |
| Fig. 4 Schematic diagram of the physicochemical and physiological conditions in different regions of the human gastrointestinal tract that determine the liberation, absorption, metabolism and distribution of bioactives. | |
5.1.1.1. Release from food or drug matrix.
The breakdown of the matrix surrounding a bioactive agent within the human GIT is usually carried out by mechanical, chemical, and enzymatic means.37–39 Foods are usually masticated within the mouth to break them down into smaller fragments prior to swallowing, whereas pharmaceutical preparations (such as capsules and pills) are usually swallowed directly. After swallowing, pharmaceutical or drug matrices may be broken down in the stomach and small intestine due to the mechanical motions of the GIT, e.g., peristalsis or grinding.38,40,41 The high acidity and ionic strength of the stomach also facilitates the dissociation of certain structures, particularly those held together by electrostatic interactions.39,42 Some matrix dissociation may also occur due to the simple fact that the material is dissolved within an aqueous environment, e.g., pills, capsules, or powders formed from water-soluble substances such as carbohydrates or proteins. The activity of digestive enzymes (such as amylases, proteases, and lipases) stimulates the breakdown of major food components (such as starches, proteins, and lipids), which often play an important role in maintaining the matrix structure in foods and drug preparations. Secreted biological surfactants in the GIT, such as bile salts and phospholipids, may also facilitate the breakdown of matrix structures held together by hydrophobic interactions in foods and drug preparations, particularly those containing lipids or surface active agents.
Excipient foods may enhance one or more of these processes by numerous mechanisms. Ingestion of an excipient food may stimulate the release of hormones that promote the release of acids, enzymes, or bile salts within the GIT,43,44 thereby promoting the liberation of bioactive agents by facilitating the breakdown of matrix structures in foods or drug preparations. The co-ingestion of bioactive lipophilic agents with an excipient food may change their bioavailability by altering their transit time within the GIT. Food components that delay transit may lead to higher absorption of bioactive agents since then there is more time for them to be liberated and absorbed. The presence of fats within an excipient food may facilitate the release of lipophilic bioactive agents from co-ingested foods or pharmaceuticals by acting as an organic solvent. Salts, acids, bases, or chelating agents in an excipient food may contribute to the breakdown of matrix structures in foods or drug preparations by altering the molecular interactions between structural components. A number of food components may alter the intestinal pH due to their acidity, alkalinity or buffering capacity.45 For example, ingestion of high amounts of protein may lead to a higher gastric pH due to the strong buffering capacity of some protein molecules. Changes in pH may alter the rate and extent of breakdown of food or pharmaceutical matrix structures and therefore the liberation of bioactive components.
5.1.1.2. Solubilization in mixed micelles.
After a lipophilic bioactive agent is liberated from the original food or pharmaceutical matrix it needs to be solubilized within the mixed micelle phase so that it can be transported to the intestinal epithelial cells. It is well established that co-ingestion of lipophilic drugs or nutraceuticals with lipids can greatly increase their oral bioavailability, which can be attributed to a number of factors.2,13,22 First, ingestion of lipids stimulates the release of digestive enzymes and bile salts, as well as increasing GIT transit time. An increase in the bile salt levels increases the solubilization capacity of the intestinal fluids, whereas an increase in GIT transit time increases the time available for any ingested bioactive agents to be liberated, solubilized, and absorbed. Second, the digestion of co-ingested lipids (triglycerides) within the GIT leads to the formation of free fatty acids (FFA) and monoacylglycerols (MAG) that are incorporated into the mixed micelles in the small intestine thereby increasing their solubilization capacity for lipophilic bioactives (see later section). Third, ingestion of any surface active substances (such as phospholipids or surfactants) may also increase the solubilization capacity of the intestinal fluids due to their ability to be incorporated into mixed micelles.46–48
5.1.1.3. Alteration of mass transport processes.
The liberation of lipophilic bioactive agents within the GIT often depends on the mass transport of reactants, catalysts, and products from one location to another. Digestive enzymes must come into close proximity to their substrates before they can carry out their catalytic actions. Bioactive agents solubilized within mixed micelles must be transported through the lumen and across the mucous layer before they can be absorbed by epithelial cells (Fig. 5). The rate and extent of liberation of bioactive agents from food or drug matrices may therefore be controlled by incorporating food ingredients within excipient foods that alter mass transport processes within the lumen of the GIT. In general, mass transport may occur by convective or diffusive processes, depending on the structural and physicochemical properties of the intestinal fluids and the flow profile within the region of the GIT involved.49 The mechanical forces generated by the GIT mix components together and help move them from one location to another.40,50 Nevertheless, there are regions within the GIT where mass transport is primarily diffusion-limited, e.g., the movement of small molecules through gelled phases. Excipient food components may be able to alter diffusion-limited or convection-limited processes by various mechanisms: binding to bioactive agents; altering the microscopic or macroscopic rheology of the intestinal fluids; altering GIT motility. For example, some biopolymers are able to form viscous solutions or gels under simulated gastrointestinal conditions, and may therefore be able to alter mass transport and transit times, which in turn alter important events affecting the release and processing of bioactive agents.51,52 Cationic biopolymers, such as chitosan, are able to bind anionic bile salts and free fatty acids, and therefore alter their mass transport.53,54
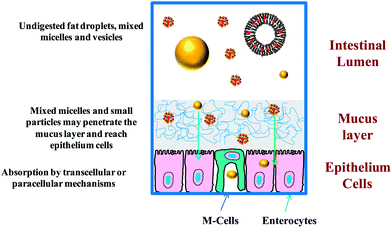 |
| Fig. 5 Bioactives in molecular form or trapped within small particles may penetrate through the mucus layer and be absorbed by epithelium cells by various mechanisms. | |
5.1.1.4. Alterations in gut motility.
Certain kinds of food components have been shown to alter the motility of the GIT, e.g., gastric emptying time or the mechanical actions of the stomach and small intestine.16,32,38 The co-ingestion of a bioactive agent with a meal often increases the length of time it spends within the stomach.16 Specific phytochemicals, such as piperine, have also been shown to inhibit gastric emptying.55 The longer a food spends within the stomach the greater time there is for the breakdown of any matrices that normally inhibit the liberation of the bioactive agents into the intestinal fluids (e.g., cell walls in plant tissues or solid drug forms). In addition, an increase in gastric emptying time may increase the amount of digestion, metabolism, or chemical transformation of a substance that occurs within the stomach. In some cases, this may increase the bioavailability of an ingested nutraceutical or pharmaceutical, e.g., if the transformed form has a higher bioavailability than the original form, or if some of the components released from the food matrix increase the subsequent solubilization or absorption of the bioactive agents. In other cases, an increase in gastric retention may decrease bioavailability, e.g., if the transformed form has a lower bioavailability than the original form, or if some of the components released from the food matrix inhibit the subsequent solubilization or absorption of the bioactive agents. Furthermore, an increase in the gastric emptying time also slows down the rate at which bioactive agents are transported to the small intestine, which may have a significant impact on their absorption and metabolism in the small intestine.
5.1.2. Bioactive absorption.
There are numerous physicochemical and physiological mechanisms by which food matrix components could alter the absorption of co-ingested lipophilic bioactive agents. A number of the most important mechanisms that might be used in the development of excipient foods are highlighted in this section.
5.1.2.1. Increase in membrane permeability.
The bioavailability of some lipophilic bioactive agents is limited by their transport across the layer of epithelial cells surrounding the GIT.56,57 When bioactive agents reach the apical side of the intestinal epithelial cells they may be transported into the systemic circulation by a number of passive or active transport processes (Fig. 5). The precise mechanism(s) involved depend on the molecular characteristics of the bioactive, the nature of any particles that the bioactive might be trapped within or bound with, the composition and structure of the surrounding intestinal fluids, and the region of the GIT where absorption occurs.
The two major types of epithelial cells that line the gastrointestinal tract in regions where the majority of absorption occurs are enterocytes and M-cells.58–61 Enterocytes are the most numerous type of cell lining the GIT, and they are where most of the absorption of molecular forms of drugs and nutraceuticals occur. Enterocytes also have ability to absorb certain types of particulate matter. Conversely, M-cells are much less numerous than enterocytes, typically occupying less than 1% of the epithelium surface, but they are much more efficient than enterocytes at absorbing particulate matter. M-cells are mainly found in specialized regions on the epithelium surface referred to as “Peyers patches”, which are primarily responsible for absorbing ingested antigens, such as macromolecules, microorganisms, and certain types of particles. The absorbed particles are then transported to the underlying lymphoid system where they promote immune responses.58,62
Molecules and particles reaching the epithelial cells may be absorbed through a number of mechanisms depending on their characteristics:61–63
Paracellular: Small molecules and particles are able to pass through the narrow gaps (“tight junctions”) that separate neighboring epithelial cells (Fig. 5). Typically, only substances that are smaller than a few nanometers are able to pass through the tight junctions. However, some substances found in foods have been shown to be capable of increasing the dimensions of the tight junctions and may therefore be able to enhance transport by this mechanism,64e.g., some surfactants,65,66 polymers,67,68 minerals,69 and chelating agents.70 Specific examples of food-grade substances that might be used to increase the permeability of epithelial cells by increasing the dimensions of the tight junctions include the surfactant Tween 80,65 the polymer chitosan,67,71 the mineral zinc,69 and the chelating agent EDTA.70
Transcellular: Molecules and particles may also be transported through epithelial cell membranes by passive or active transport mechanisms (Fig. 5). Many fairly lipophilic molecules are transferred across cell membranes by a passive mechanism. After encountering the epithelial cells, they are solubilized within the non-polar phospholipid tails that make up the phospholipid bilayer of the cell membrane. After moving across the cell membrane, they are incorporated into various vesicle-like structures on the other side, which then move them into the cell interior. Other types of molecules (particularly more hydrophilic ones) are transferred across the cell membrane by membrane protein-transporter systems. The absorption of particles that are small enough to travel through the mucus layer and reach the surface of the epithelial cells typically occurs by an “endocytosis” mechanism.61 In this case, particles come into contact with the outer wall of the cell membrane, the membrane then wraps itself around the particle, and then part of the membrane buds-off to form a vesicle-like structure with a particle trapped inside that moves into the interior of the cell. This process may occur in enterocyte cells, but is typically much more active in M-cells. The critical cut-off particle size for endocytosis has been estimated to be from less than 50 to around 100 nm for enterocyte cells, and to be from 20 to 500 nm for M-cells.
Certain types of molecules present in foods may be able to increase the transcellular uptake of lipophilic bioactive agents by epithelial cells by altering cell membrane permeability. Piperine (a compound found in black pepper) has been shown to be capable of increasing cell membrane permeability.32 Food grade surfactants (sucrose monoesters) have also been shown to increase membrane permeability to model drugs.72 Rhamnolipids have been shown to increase both transcellular and paracelluar transport of model drugs.73
Persorption: Molecules or particles may also be absorbed through temporary pores formed in the layer of epithelial cells lining the GIT due to gaps formed when some of the cells are shed and replaced.63
5.1.2.2. Inhibition of efflux mechanisms.
The bioavailability of certain types of lipophilic bioactive agents is limited due to the presence of efflux mechanisms in the membranes of the intestinal epithelial cells.57,74,75 After absorption by epithelial cells, some bioactives are transported back into the intestinal lumen by specific transports at the apical side of the cell membrane. For example, both P-glycoprotein (P-gp) and multidrug resistant protein (MRP) have been shown to pump out a wide range of lipophilic bioactives from epithelial cells lining the GIT.57,74 This efflux process can reduce the bioavailability of bioactive agents by two mechanisms: (i) decreasing the total amount absorbed; and, (ii) increasing the extent of metabolism within the GIT if the bioactive is pumped out and then reabsorbed, which increase exposure of the bioactive to metabolizing enzymes inside of the epithelial cells. Certain types of food-grade components have been shown to be able to block efflux mechanisms, and thereby increase the net absorption of lipophilic bioactive agents by epithelial cells, e.g., some surfactants, chelating agents, biopolymers, and phytochemicals.5,57,76,77 For example, resveratrol, quercetin and piperine have been shown to act as efflux inhibitors for certain kinds of drugs.76,78–81 In general, three different mechanisms have been proposed for the ability of these components to inhibit efflux processes: (i) blocking binding sites on the efflux protein surfaces; (ii) interference with ATP hydrolysis (which provides the energy needed for efflux protein action); (iii) alteration of cell membrane structure (which leads to alterations in efflux protein conformation and activity).57
5.1.3. Bioactive metabolism or chemical transformation.
Numerous molecules isolated from plant and animal sources have been shown to enhance the bioavailability of nutraceuticals or pharmaceuticals due to their ability to interfere with chemical transformations that normally occur within the GIT or after absorption.32 Some of these bioactivity enhancers act as antioxidants that retard the oxidation of nutraceuticals or pharmaceuticals, such as ω-3 fatty acids, carotenoids, or conjugated linoleic acid.82 For example, there are many natural and synthetic food-grade antioxidants that are effective at inhibiting oxidation reactions by mechanisms such as free radical scavenging, singlet oxygen quenching, and chelating, e.g., BHT, BHA, carotenoids, tocopherols, flavonoids, and grade seed extract.83 Other bioactivity enhancers may inhibit the normal functioning of metabolic or digestive enzymes within the GIT or body.23,32 For example, piperine has been shown to retard the metabolism of certain drugs and nutraceuticals, such as ibuprofen, curcumin, resveratrol, EGCG, carotenoids, vitamins, and amino acids.32 These affects have been partly attributed to its ability to inhibit metabolizing enzymes such as glucose dehydrogenase, cytochrome P450, and others (Table 2).32
Table 2 Examples of phytochemicals from natural sources that may increase the bioavailability of co-ingested lipophilic nutraceuticals and pharmaceuticals. Examples taken from various sources: Dudhatra,32 Shimizu,23 Choi,84 Jia77
Bioavailability enhancer |
Nutraceuticals enhanced |
Mechanism |
Piperine |
Vitamins A, D, E, K |
Metabolizing enzyme inhibition |
Carotenoids |
Modulation of gut motility |
Curcuminoids |
|
Coenzyme Q10 |
|
Hydrophobic drugs |
|
Gingerols |
Vitamins A and E |
Modulation of gut motility |
Carotenoids, curcumin |
|
Curcumin |
Hydrophobic drugs |
Metabolizing enzyme inhibition |
Efflux transporter inhibition |
Quercetin |
Hydrophobic drugs |
Efflux transporter inhibition |
5.2. Excipient food ingredients
In this section, the potential influence of common food components that may be incorporated into excipient foods on the oral bioavailability of lipophilic bioactive agents is discussed. Those ingredients that appreciably increase the bioavailability of nutraceuticals can be referred to as “excipient food ingredients”. An excipient food may contain one or more of these ingredients so as to increase the bioavailability of one or more nutraceuticals.
5.2.1. Lipids.
Studies by pharmaceutical researchers have shown that co-ingestion of lipophilic drugs with lipids improves theirs oral bioavailability by an amount that depends on the amount, type, and structure of the ingested lipids.2 Food and nutrition research has also shown that the bioavailability of lipophilic nutraceuticals can be increased by co-ingestion with lipids.85–87In vitro studies have reported that the bioaccessibility (micelle solubilization) and absorption (cell culture uptake) of lipophilic bioactive agents from fruits and vegetables is greatly increased in the presence of lipids.85,86 The extent of the increase in bioaccessibility and absorption depends on the amount and composition of the lipids used.86,88,89 Bioaccessibility was higher for lipids containing long chain triglycerides (LCT) than those containing short or medium chain triglycerides (SCT or MCT), presumably because of differences in the solubilization capacity of the mixed micelles formed.2,90 Lipophilic bioactives encapsulated within indigestible oils (flavor oils) have been shown to have low bioaccessibility using in vitro studies, which was attributed to the fact that some of them remained in the undigested oil droplets and there were fewer mixed micelles available to solubilize them.90,91 In addition to their composition, the liberation of bioactives from emulsified lipids also depends on their particle size, physical state, and interfacial characteristics.92,93 Typically, the release rate is faster for smaller particles, for liquid oils rather than solid fats, and for interfaces where bile salts and lipases can easily absorb.
Co-ingested lipids may also alter the bioavailability of lipophilic drugs or nutraceuticals through other mechanisms. When lipophilic bioactives are ingested with LCT they are packed into lipoprotein particles (chylomicrons) in the intestinal epithelial cells and then transported by the lymphatic route (thereby avoiding first pass metabolism in the liver), but when they are ingested with SCT or MCT they tend to be transported via the portal vein (where they must pass through the liver before entering the systemic blood circulation).34,94 Bioactives packaged in different vehicles (e.g., chylomicron vs. non-chylomicron) in the epithelial cells may have different metabolic fates due to differences in their exposure to metabolizing enzymes present in different body tissues.
5.2.2. Carbohydrates.
In general, food carbohydrates are classified as monosaccharides (n = 1), oligosaccharides (n = 2 to 20), or polysaccharides (n > 20) depending on the number of monomers present.95 Carbohydrates may also be classified as digestible or indigestible depending on their susceptibility to enzymatic hydrolysis in the upper GIT.96,97 Starch is the most abundant digestible polysaccharide in foods, whereas there are many types of indigestible polysaccharides, such as cellulose, hemicellulose, pectin, alginate, carrageenan, xanthan gum, locust bean gum, and agar. Indigestible polysaccharides are part of a class of polymers known as dietary fibers, which vary according to their monomer type, distribution, and bonding, as well as their electrical charge, hydrophobicity, molecular weight, degree of branching, and conformation.95,97 Co-ingested carbohydrates may influence the bioavailability of lipophilic bioactive drugs and nutraceuticals through various mechanisms. As mentioned earlier, many polysaccharides are able to increase the viscosity or form a gel within the GIT, thereby altering mass transport processes, e.g., diffusion of enzymes to substrates in food matrices, or digestion products/bioactives to epithelial cells. Some dietary fibers may be able to form impermeable coatings around food matrix components that inhibit their digestion and therefore the release of bioactive agents.92 Electrically charged polysaccharides are capable of binding oppositely charged molecular species in the GIT that may influence food matrix digestion and bioactive release. For example, cationic dietary fibers (such as chitosan) can bind anionic bile salts, fatty acids, or phospholipids, whereas anionic dietary fibers (such as alginate) can bind cationic calcium ions.27,98–100 Cationic dietary fibers have also been shown to inhibit lipase activity, and therefore reduce the rate of lipid digestion.101 Some dietary fibers have been shown to alter cell membrane permeability through their effect on tight junction dimensions, e.g., chitosan.67,68 Dietary fibers may also change the nature of the microbial population within the colon, which can alter the metabolism, activity, and absorption of lipophilic bioactives in the large intestine.102
5.2.3. Proteins.
Food proteins exhibit a wide range of different molecular structures, physicochemical properties, and physiological effects.103,104 Co-ingested proteins can potentially alter the bioavailability of lipophilic bioactive agents through a number of mechanisms. Many food proteins and peptides have strong antioxidant activity and may therefore be able to inhibit the chemical degradation of nutraceuticals or drugs that are susceptible to oxidation within the GIT, such as ω-3 fatty acids or carotenoids.105 Some nutraceuticals may bind to proteins within the GIT,106 which alters the location of their absorption within the GIT, e.g., anthocyanins bound to proteins have been shown to travel further down the gastrointestinal tract.107 Protein digestion within the gastrointestinal tract may generate hormonal responses that regulate food intake and processing,108 thereby altering the way that a food or pharmaceutical matrix is broken down in the GIT and therefore the release of any trapped bioactive agents. Proteins and their digestion products may interact with various molecular species involved in the digestion of food matrices and the release and transport of bioactive agents, such as bioactives, mixed micelles, phospholipids, and enzymes.109–112 For example, a recent study suggests that lactoferrin may reduce the bioavailability of β-carotene, which was attributed to the fact that it was positively charged and bound to negatively charged digestive components, such as bile salts or free fatty acids.113 Some protein digestion products, for example those from casein and whey proteins, have been shown to alter (close) tight junction permeability, and may therefore alter the uptake of any nutraceuticals absorbed by this mechanism.23
5.2.4. Surfactants.
Surfactants are commonly used in the food and pharmaceutical industries to form and stabilize colloidal delivery systems, such as microemulsions, nanoemulsions, emulsions, and solid lipid nanoparticles.114,115 Surfactants vary in the nature of their polar head groups and non-polar tail groups, which alters their behavior within foods and the GIT. The head group may be non-ionic, cationic, anionic, or zwitterionic, while the tail group may vary in the number, length and unsaturation of the non-polar chains. Synthetic or natural surfactants may be present within an ingested food e.g., non-ionic surfactants (e.g., Tweens, Spans, and sucrose esters), ionic surfactants (e.g., DATEM and CITREM), phospholipids (e.g., egg, soy, or sunflower lecithin), or monoacylglycerols.116 Alternatively, they may be generated from ingested food components as a result of the digestion process, e.g., monoacylglycerols from triacylglycerols or lysolecithin from phospholipids.117 Surfactants can alter the bioavailability of lipophilic bioactives through a number of mechanisms: some surfactants bind to digestive enzymes (such as lipase or protease) and alter their activity;118 surfactants may be incorporated into mixed micelles thereby increasing their solubilization capacity;119 surfactants may inhibit lipase absorption to lipid surfaces through competitive absorption;93,120 surfactants may alter the permeability of enterocytes by interacting with transporters on cell membranes;121 surfactants may increase cell permeability by increasing the dimensions of the tight junctions.23,65,122
5.2.5. Minerals.
Certain types of mineral ions also impact the liberation and absorption of lipophilic bioactives. For example, calcium ions may impact the rate and extent of lipid hydrolysis, which influences the release of bioactives from the lipid phase and their subsequent solubilization in the mixed micelle phase.123,124 In the absence of calcium, the digestion of triacylglycerols in the small intestine is inhibited by accumulation of long-chain fatty acids (LCFA) at the oil–water interface, since this restricts the access of lipase to the lipid substrate.27 Calcium ions precipitate accumulated LCFAs through complexation, thereby removing them from the interface and allowing the lipase to access the lipid substrate.123,125,126 Calcium ions are therefore able to increase the rate and extent of lipid digestion through this mechanism.127–130 Conversely, the formation of calcium–LCFA precipitates may reduce the solubilization capacity of the mixed micelle phase, thereby reducing the bioavailability of LCFAs and lipophilic bioactives.123,131–133 Calcium has also been shown to play an important role in the activity of pancreatic lipase, acting as a co-factor required for activity.134–137 Multivalent mineral ions may promote the aggregation of oppositely charged lipid droplets,114 thereby altering the surface area of lipid exposed to digestive enzymes. Mineral ions may also promote gelation of oppositely charged biopolymers (e.g., calcium ions promote alginate gelation), which will also influence the accessibility of lipid phases to enzyme digestion.138 Some minerals have been shown to influence the absorption of bioactive agents by altering cell membrane permeability, e.g., zinc.69
5.2.6. Chelating agents.
Metal ion chelators (such as EDTA) have been shown to inhibit efflux transporters in the GIT, and may therefore increase the bioavailability of bioactive molecules that are susceptible to removal from enterocytes by this mechanism.57 Metal ion chelators (such as EDTA and phosphates) may interfere with the various roles that calcium ions play in the digestion and release of lipids by complexing them – see Section 5.2.5.124
5.2.7. Phytochemicals.
A number of phytochemicals derived from edible plant materials have been shown to be able to promote the bioavailability of certain bioactive food agents. For example, some polyphenols affect absorption and efflux transporters in enterocyte membranes thus altering the accumulation of bioactive agents within the body e.g., quercetin, curcumin, piperine, and some catechins.139–142 Specific phytochemicals may also be able to inhibit chemical reactions (such as lipid oxidation) or biochemical reactions (such as digestion or metabolism) in the gastrointestinal tract.23,32 For example, it has been reported that piperine reduced the metabolism of curcumin in the GIT by inhibiting metabolizing enzymes, thereby increasing bioavailability32 (see Sections 5.1.2.2 and 5.1.3).
5.2.8. Excipient food ingredients.
Many of the food ingredients discussed in the previous sections have the ability to increase the oral bioavailability of co-ingested bioactive agents. These ingredients can therefore be used to construct excipient foods that are specifically designed to increase the overall oral bioavailability of one or more type of co-ingested bioactive agents. For example, an excipient food may contain lipids to increase the solubilization capacity of the intestinal fluids, a phytochemical to inhibit efflux mechanisms, and a surfactant to increase epithelium cell membrane permeability.
6. Conclusions
This article has introduced the concept of excipient foods that are specifically designed to enhance the oral bioavailability of lipophilic bioactive agents such as nutraceuticals in foods or drugs in pharmaceuticals. Knowledge of the influence of specific food components and structures on the bioavailability of specific lipophilic bioactive agents is increasing, which will facilitate the rational design of food matrices that can enhance the biological activity of nutraceuticals and drugs. A number of different approaches can be used, including increasing the release, solubilization, transport, and uptake of bioactive agents, while decreasing their metabolism or efflux.
There is a growing convergence in the interests of pharmaceutical and food companies. The food industry is increasingly focusing on the development of functional food and beverage products designed to improve performance, maintain wellbeing, and inhibit the onset of chronic diseases, such as osteoporosis, heart disease, cancer, hypertension, and obesity. The pharmaceutical industry continues to develop products to prevent, manage, and cure chronic and acute diseases. Many of the biologically active substances present in foods and drugs are highly lipophilic agents that normally have poor oral bioavailability. The availability of a range of excipient foods specifically designed to increase the oral bioavailability of lipophilic bioactive molecules would therefore be beneficial to both the pharmaceutical and food industries. Nevertheless, further research is required to better understand the role of specific excipient food ingredients on the bioavailability of specific lipophilic bioactive agents, and to establish the influence of ingredient interactions on bioavailability when excipient foods are consumed as part of a complex diet that contains many other components.
Acknowledgements
This material is based upon work supported by the Cooperative State Research, Extension, Education Service, United State Department of Agriculture, Massachusetts Agricultural Experiment Station and United States Department of Agriculture, NIFA grant and a USDA/EPA/NSF grant.
References
- J. S. Jingling Tang, Curr. Drug Ther., 2007, 2, 85–93 CrossRef.
- C. J. H. Porter, N. L. Trevaskis and W. N. Charman, Nat. Rev. Drug Discovery, 2007, 6, 231–248 CrossRef CAS PubMed.
- E. Fernandez-Garcia, I. Carvajal-Lerida, M. Jaren-Galan, J. Garrido-Fernandez, A. Perez-Galvez and D. Hornero-Mendez, Food Res. Int., 2012, 46, 438–450 CrossRef CAS PubMed.
- A. R. Patel and K. P. Velikov, LWT–Food Sci. Technol., 2011, 44, 1958–1964 CrossRef CAS PubMed.
- M. J. Rein, M. Renouf, C. Cruz-Hernandez, L. Actis-Goretta, S. K. Thakkar and M. D. Pinto, Br. J. Clin. Pharmacol., 2013, 75, 588–602 CAS.
- D. Fleisher, C. Li, Y. Zhou, L. H. Pao and A. Karim, Clin. Pharmacokinet., 1999, 36, 233–254 CrossRef CAS PubMed.
- K. R. N. Moelants, L. Lemmens, M. Vandebroeck, S. Van Buggenhout, A. M. Van Loey and M. E. Hendrickx, J. Agric. Food Chem., 2012, 60, 11995–12003 CrossRef CAS PubMed.
- C. W. Pouton and C. J. Porter, Adv. Drug Delivery Rev., 2008, 60, 625–637 CrossRef CAS PubMed.
- M. N. Martinez and G. L. Amidon, J. Clin. Pharmacol., 2002, 42, 620–643 CrossRef CAS PubMed.
- S. Hurst, C. M. Loi, J. Brodfuehrer and A. El-Kattan, Expert Opin. Drug Metab. Toxicol., 2007, 3, 469–489 CrossRef CAS.
- D. N. D'Ambrosio, R. D. Clugston and W. S. Blaner, Nutrients, 2011, 3, 63–103 CrossRef CAS PubMed.
- Y. Y. Yeap, N. L. Trevaskis, T. Quach, P. Tso, W. N. Charman and C. J. H. Porter, Mol. Pharm., 2013, 10, 1874–1889 CrossRef CAS PubMed.
- W. N. Charman, C. J. H. Porter, S. Mithani and J. B. Dressman, J. Pharm. Sci., 1997, 86, 269–282 CrossRef CAS PubMed.
- M. J. Brown, M. G. Ferruzzi, M. L. Nguyen, D. A. Cooper, A. L. Eldridge, S. J. Schwartz and W. S. White, Am. J. Clin. Nutr., 2004, 80, 396–403 CAS.
- A. Nagao, E. Kotake-Nara and M. Hase, Biosci., Biotechnol., Biochem., 2013, 77, 1055–1060 CrossRef CAS.
- F. J. O. Varum, G. B. Hatton and A. W. Basit, Int. J. Pharm., 2013, 457, 446–460 CrossRef CAS PubMed.
- L. Salvia-Trujillo, C. Qian, O. Martin-Belloso and D. J. McClements, Food Chem., 2013, 141, 1472–1480 CrossRef CAS PubMed.
- H. Kalasz and I. Antal, Curr. Med. Chem., 2006, 13, 2535–2563 CrossRef CAS.
- J. Hamman and J. Steenekamp, Expert Opin. Drug Delivery, 2012, 9, 219–230 CrossRef CAS PubMed.
-
A. T. Florence and D. Attwood, Physicochemical Principles of Pharmacy, Pharmaceutical Press, London, UK, 2011 Search PubMed.
- D. J. McClements, E. A. Decker, Y. Park and J. Weiss, Crit. Rev. Food Sci. Nutr., 2009, 49, 577–606 CrossRef CAS PubMed.
- H. D. Williams, N. L. Trevaskis, S. A. Charman, R. M. Shanker, W. N. Charman, C. W. Pouton and C. J. H. Porter, Pharmacol. Rev., 2013, 65, 315–499 CrossRef.
- M. Shimizu, Biosci., Biotechnol., Biochem., 2010, 74, 232–241 CrossRef CAS.
- J. A. Arnott and S. L. Planey, Expert Opin. Drug Delivery, 2012, 7, 863–875 CrossRef CAS PubMed.
- D. J. McClements, Expert Opin. Drug Delivery, 2013, 10, 1621–1632 CrossRef CAS PubMed.
- E. Bauer, S. Jakob and R. Mosenthin, Asian–Australas. J. Anim. Sci., 2005, 18, 282–295 CAS.
- G. Fave, T. C. Coste and M. Armand, Cell. Mol. Biol., 2004, 50, 815–831 CAS.
- B. Bermudez, Y. M. Pacheco, S. Lopez, R. Abia and F. J. G. Muriana, Grasas Aceites, 2004, 55, 1–10 CAS.
- A. Mullertz, D. G. Fatouros, J. R. Smith, M. Vertzoni and C. Reppas, Mol. Pharmaceutics, 2012, 9, 237–247 CrossRef CAS PubMed.
- H. Singh, A. Ye and D. Horne, Prog. Lipid Res., 2009, 48, 92–100 CrossRef CAS PubMed.
- K. Larsson, L. Cavonius, M. Alminger and I. Undeland, J. Agric. Food Chem., 2012, 60, 7556–7564 CrossRef CAS PubMed.
- G. B. Dudhatra, S. K. Mody, M. M. Awale, H. B. Patel, C. M. Modi, A. Kumar, D. R. Kamani and B. N. Chauhan, Sci. World J., 2012, 1–33 CrossRef PubMed.
- T. Y. Wang, M. Liu, P. Portincasa and D. Q. H. Wang, Eur. J. Clin. Invest., 2013, 43, 1203–1223 CAS.
- J. A. Yanez, S. W. J. Wang, I. W. Knemeyer, M. A. Wirth and K. B. Alton, Adv. Drug Delivery Rev., 2011, 63, 923–942 CrossRef CAS PubMed.
- J. C. Espin, M. T. Garcia-Conesa and F. A. Tomas-Barberan, Phytochemistry, 2007, 68, 2986–3008 CrossRef CAS PubMed.
- A. Ruiz-Garcia, M. Bermejo, A. Moss and V. G. Casabo, J. Pharm. Sci., 2008, 97, 654–690 CrossRef CAS PubMed.
- G. A. van Aken, Food Biophys., 2010, 5, 258–283 CrossRef.
- M. Koziolek, G. Garbacz, M. Neumann and W. Weitschies, Mol. Pharmaceutics, 2013, 10, 1610–1622 CrossRef CAS PubMed.
-
R. G. Lentle and W. M. J. Patrick, The Physical Processes of Digestion, Springer, New York, NY, 2011 Search PubMed.
- F. Kong and R. P. Singh, J. Food Sci., 2008, 73, R67–R80 CrossRef CAS PubMed.
- R. G. Lentle and P. W. M. Janssen, Crit. Rev. Food Sci. Nutr., 2010, 50, 130–145 CrossRef CAS PubMed.
- A. Matalanis and D. J. McClements, Food Biophys., 2012, 7, 145–154 CrossRef.
- J. L. Boyer, Comprehensive Physiology, 2013, 3, 1035–1078 Search PubMed.
- M. Covasa, Am. J. Physiol.: Regul., Integr. Comp. Physiol., 2010, 299, R1423–R1439 CrossRef CAS PubMed.
- L. Kalantzi, K. Goumas, V. Kalioras, B. Abrahamsson, J. B. Dressman and C. Reppas, Pharm. Res., 2006, 23, 165–176 CrossRef CAS PubMed.
- D. M. Cirin, M. M. Posa and V. S. Krstonosic, Ind. Eng. Chem. Res., 2012, 51, 3670–3676 CrossRef CAS.
- S. Rozner, D. E. Shalev, A. I. Shames, M. F. Ottaviani, A. Aserin and N. Garti, Colloids Surf., B, 2010, 77, 22–30 CrossRef CAS PubMed.
- C. Rupp, H. Steckel and B. W. Muller, Int. J. Pharm., 2010, 395, 272–280 CrossRef CAS PubMed.
- J. M. Rabanel, V. Aoun, I. Elkin, M. Mokhtar and P. Hildgen, Curr. Med. Chem., 2012, 19, 3070–3102 CrossRef CAS.
- M. J. S. Wickham, R. M. Faulks, J. Mann and G. Mandalari, Dissolution Technol., 2012, 19, 15–22 CAS.
- J. F. Bradbeer, R. Hancocks, F. Spyropoulos and I. T. Norton, Food Hydrocolloids, 2014, 35, 522–530 CrossRef CAS PubMed.
- T. J. Wooster, L. Day, M. Xu, M. Golding, S. Oiseth, J. Keogh and P. Clifton, Food Hydrocolloids, 2014, 36, 102–114 CrossRef CAS PubMed.
- T. Helgason, J. Weiss, D. J. McClements, J. Gislason, J. M. Einarsson, F. R. Thormodsson and K. Kristbergsson, J. Aquat. Food Prod. Technol., 2008, 17, 216–233 CrossRef CAS.
- M. Thongngam and D. J. McClements, Food Hydrocolloids, 2005, 19, 813–819 CrossRef CAS PubMed.
- S. Bajad, K. L. Bedi, A. K. Singla and R. K. Johri, Planta Med., 2001, 67, 176–179 CrossRef CAS PubMed.
- A. Dahan and J. M. Miller, AAPS J., 2012, 14, 244–251 CrossRef CAS PubMed.
- P. Fasinu, V. Pillay, V. M. K. Ndesendo, L. C. du Toit and Y. E. Choonara, Biopharm. Drug Dispos., 2011, 32, 185–209 CrossRef CAS PubMed.
- A. des Rieux, V. Fievez, M. Garinot, Y. J. Schneider and V. Preat, J. Controlled Release, 2006, 116, 1–27 CrossRef CAS PubMed.
- L. M. Ensign, R. Cone and J. Hanes, Adv. Drug Delivery Rev., 2012, 64, 557–570 CrossRef CAS PubMed.
-
G. J. Doherty and H. T. McMahon, in Annual Review of Biochemistry, Annual Reviews, Palo Alto, 2009, vol. 78, pp. 857–902 Search PubMed.
- M. Bohdanowicz and S. Grinstein, Physiol. Rev., 2013, 93, 69–106 CrossRef CAS PubMed.
- E. Frohlich and E. Roblegg, Toxicology, 2012, 291, 10–17 CrossRef PubMed.
- J. J. Powell, N. Faria, E. Thomas-McKay and L. C. Pele, J. Autoimmun., 2010, 34, J226–J233 CrossRef CAS PubMed.
- S. Maher, D. J. Brayden, L. Feighery and S. McClean, Crit. Rev. Ther. Drug Carrier Syst., 2008, 25, 117–168 CrossRef CAS.
- F. Buyukozturk, J. C. Benneyan and R. L. Carrier, J. Controlled Release, 2010, 142, 22–30 CrossRef CAS PubMed.
- V. Gupta, B. H. Hwang, N. Doshi and S. Mitragotri, J. Controlled Release, 2013, 172, 541–549 CrossRef CAS PubMed.
- M. C. Chen, F. L. Mi, Z. X. Liao, C. W. Hsiao, K. Sonaje, M. F. Chung, L. W. Hsu and H. W. Sung, Adv. Drug Delivery Rev., 2013, 65, 865–879 CrossRef CAS PubMed.
- V. Pillay, A. R. Hibbins, Y. E. Choonara, L. C. du Toit, P. Kumar and V. M. K. Ndesendo, Int. J. Pept. Res. Ther., 2012, 18, 259–280 CrossRef CAS.
- X. X. Wang, M. C. Valenzano, J. M. Mercado, E. P. Zurbach and J. M. Mullin, Dig. Dis. Sci., 2013, 58, 77–87 CrossRef CAS PubMed.
- H. J. R. Lemmer and J. H. Hamman, Expert Opin. Drug Delivery, 2013, 10, 103–114 CrossRef CAS PubMed.
- G. Di Colo, Y. Zambito and C. Zaino, J. Pharm. Sci., 2008, 97, 1652–1680 CrossRef CAS PubMed.
- A. Yamamoto, H. Katsumi, K. Kusamori and T. Sakane, J. Pharm. Soc. Jpn., 2014, 134, 47–53 CrossRef PubMed.
- L. F. Jiang, X. W. Long and Q. Meng, Int. J. Pharm., 2013, 446, 130–135 CrossRef CAS PubMed.
- P. P. Constantinides and K. M. Wasan, J. Pharm. Sci., 2007, 96, 235–248 CrossRef CAS PubMed.
- J. M. Planas, I. Alfaras, H. Colom and M. E. Juan, Arch. Biochem. Biophys., 2012, 527, 67–73 CrossRef CAS PubMed.
- M. J. Jin and H. K. Han, J. Food Sci., 2010, 75, H93–H96 CrossRef CAS PubMed.
- J. X. Jia and K. M. Wasan, J. Pharm. Pharm. Sci., 2008, 11, 45–62 CAS.
- Y. C. Chi, S. P. Lin and Y. C. Hou, Toxicol. Appl. Pharmacol., 2012, 263, 315–322 CrossRef CAS PubMed.
- J. S. Choi, B. C. Choi and K. W. Kang, Pharmazie, 2009, 64, 49–52 CAS.
- M. J. Kang, J. Y. Cho, B. H. Shim, D. K. Kim and J. Lee, J. Med. Plants Res., 2009, 3, 1204–1211 CAS.
- V. R. Challa, P. R. Babu, S. R. Challa, B. Johnson and C. Maheswari, Drug Dev. Ind. Pharm., 2013, 39, 865–872 CrossRef CAS PubMed.
- M. Tarvainen, A. Phuphusit, J. P. Suomela, A. Kuksis and H. Kallio, J. Agric. Food Chem., 2012, 60, 3564–3579 CrossRef CAS PubMed.
-
E. A. Decker, B. Chen, A. Panya and R. J. Elias, Understanding Mechanisms of Oxidation and Antioxidant Activity, in Oxidation in Foods and Beverages and Antioxidant Applications, ed. E. A. Decker, R. J. Elias and D. J. McClements, 2011, vol. 1, pp. 225–248 Search PubMed.
- J. S. Choi, Y. J. Piao and K. W. Kang, Arch. Pharmacal Res., 2011, 34, 607–613 CrossRef CAS PubMed.
- M. L. Failla, C. Chitchumroonchokchai and B. K. Ishida, J. Nutr., 2008, 138, 482–486 CAS.
-
M. L. Failla, T. Huo and S. K. Thakkar, In vitro screening of relative bioaccessibility of carotenoids from foods, Taipei, Taiwan, 2007 Search PubMed.
- V. Tyssandier, B. Lyan and P. Borel, Biochim. Biophys. Acta, Mol. Cell Biol. Lipids, 2001, 1533, 285–292 CrossRef CAS.
- T. Huo, M. G. Ferruzzi, S. J. Schwartz and M. L. Failla, J. Agric. Food Chem., 2007, 55, 8950–8957 CrossRef CAS PubMed.
- S. R. Goltz, W. W. Campbell, C. Chitchumroonchokchai, M. L. Failla and M. G. Ferruzzi, Mol. Nutr. Food Res., 2012, 56, 866–877 CAS.
- C. Qian, E. A. Decker, H. Xiao and D. J. McClements, Food Chem., 2012, 135, 1440–1447 CrossRef CAS PubMed.
- J. Rao, E. A. Decker, H. Xiao and D. J. McClements, J. Sci. Food Agric., 2013, 93, 3175–3183 CrossRef CAS PubMed.
- D. J. McClements and Y. Li, Adv. Colloid Interface Sci., 2010, 159, 213–228 CrossRef CAS PubMed.
- E. Troncoso, J. Miguel Aguilera and D. J. McClements, Food Hydrocolloids, 2012, 27, 355–363 CrossRef CAS PubMed.
- P. Borel, V. Tyssandier, N. Mekki, P. Grolier, Y. Rochette, M. C. Alexandre-Gouabau, D. Lairon and V. Azais-Braesco, J. Nutr., 1998, 128, 1361–1367 CAS.
-
S. W. Cui, Food Carbohydrates: Chemistry, Physical Properties and Applications, Taylor and Francis, Boca Raton, FL, 2005 Search PubMed.
-
J. N. BeMiller and K. C. Huber, in Food Chemistry, ed. S. Damodaran, K. L. Parkin and O. R. Fennema, CRC Press, Bocan Raton, FL, 4th edn, 2008, ch. 4, pp. 83–154 Search PubMed.
-
C. G. Biliaderis and M. S. Izydorczyk, Functional Food Carbohydrates, CRC Press, Bocan Raton, FL, 2007 Search PubMed.
- M. Armand, Sci. Aliments, 2008, 28, 84–98 CrossRef CAS PubMed.
- D. J. McClements, E. A. Decker and Y. Park, Crit. Rev. Food Sci. Nutr., 2009, 49, 48–67 CrossRef PubMed.
- M. V. Tzoumaki, T. Moschakis, E. Scholten and C. G. Biliaderis, Food Funct., 2013, 4, 121–129 CAS.
- T. Tsujita, H. Takaichi, T. Takaku, T. Sawai, N. Yoshida and J. Hiraki, J. Lipid Res., 2007, 48, 358–365 CrossRef CAS PubMed.
- F. Fava, J. A. Lovegrove, R. Gitau, K. G. Jackson and K. M. Tuohy, Curr. Med. Chem., 2006, 13, 3005–3021 CrossRef CAS.
-
S. Damodaran, in Food Chemistry, ed. S. Damodaran, K. L. Parkin and O. R. Fennema, CRC Press, Bocan Raton, FL, 4th edn, 2008, ch. 5, pp. 217–330 Search PubMed.
-
G. O. Phillips and P. A. Williams, Handbook of Food Proteins, Woodhead Publishing, Oxford, UK, 2011 Search PubMed.
- M. C. O. Delgado, V. A. Tironi and M. C. Anon, LWT–Food Sci. Technol., 2011, 44, 1752–1760 CrossRef PubMed.
- N. Bordenave, B. R. Hamaker and M. G. Ferruzzi, Food Funct., 2014, 5, 18–34 CAS.
- D. M. Ribnicky, D. E. Roopchand, A. Oren, M. Grace, A. Poulev, M. A. Lila, R. Havenaar and I. Raskin, Food Chem., 2014, 142, 349–357 CrossRef CAS PubMed.
- I. Depoortere, Gut, 2014, 63, 179–190 CrossRef CAS PubMed.
- H. Singh and A. Sarkar, Adv. Colloid Interface Sci., 2011, 165, 47–57 CrossRef CAS PubMed.
- H. Singh, A. Q. Ye and D. Horne, Prog. Lipid Res., 2009, 48, 92–100 CrossRef CAS PubMed.
- J. Maldonado-Valderrama, P. Wilde, A. Macierzanka and A. Mackie, Adv. Colloid Interface Sci., 2011, 165, 36–46 CrossRef CAS PubMed.
- H. L. Yu and Q. R. Huang, J. Agric. Food Chem., 2011, 59, 9120–9126 CrossRef CAS PubMed.
- T. Tokle, Y. Mao and D. J. McClements, Pharm. Res., 2013, 30, 3200–3213 CrossRef CAS PubMed.
- U. Lesmes, P. Baudot and D. J. McClements, J. Agric. Food Chem., 2010, 58, 7962–7969 CrossRef CAS PubMed.
- D. J. McClements, Curr. Opin. Colloid Interface Sci., 2012, 17, 235–245 CrossRef CAS PubMed.
- I. Kralova and J. Sjoblom, J. Dispersion Sci. Technol., 2009, 30, 1363–1383 CrossRef CAS.
- D. J. McClements, E. A. Decker and Y. Park, Crit. Rev. Food Sci. Nutr., 2009, 49, 48–67 CrossRef PubMed.
- V. Delorme, R. Dhouib, S. Canaan, F. Fotiadu, F. Carriere and J. F. Cavalier, Pharm. Res., 2011, 28, 1831–1842 CrossRef CAS PubMed.
- Z. Vinarov, S. Tcholakova, B. Damyanova, Y. Atanasov, N. D. Denkov, S. D. Stoyanov, E. Pelan and A. Lips, Langmuir, 2012, 28, 12140–12150 CrossRef CAS PubMed.
- Y. Li and D. J. McClements, Eur. J. Pharm. Biopharm., 2011, 79, 423–431 CrossRef CAS PubMed.
- E. Fernandez-Garcia, F. Rincon and A. Perez-Galvez, J. Agric. Food Chem., 2008, 56, 10384–10390 CrossRef CAS PubMed.
- B. Aspenstrom-Fagerlund, L. Ring, P. Aspenstrom, J. Tallkvist, N. G. Ilback and A. W. Glynn, Toxicology, 2007, 237, 12–23 CrossRef PubMed.
- R. Devraj, H. D. Williams, D. B. Warren, A. Mullertz, C. J. H. Porter and C. W. Pouton, Int. J. Pharm., 2013, 441, 323–333 CrossRef CAS PubMed.
- M. Hu, Y. Li, E. A. Decker and D. J. McClements, Food Hydrocolloids, 2010, 24, 719–725 CrossRef CAS PubMed.
- J. S. Patton and M. C. Carey, Science, 1979, 204, 145–148 CAS.
- J. S. Patton, R. D. Vetter, M. Hamosh, B. Borgstrom, M. Lindstrom and M. C. Carey, Food Microstruct., 1985, 4, 29–41 CAS.
- N. H. Zangenberg, A. Mullertz, H. G. Kristensen and L. Hovgaard, Eur. J. Pharm. Sci., 2001, 14, 237–244 CrossRef CAS.
- N. H. Zangenberg, A. Mullertz, H. G. Kristensen and L. Hovgaard, Eur. J. Pharm. Sci., 2001, 14, 115–122 CrossRef CAS.
- M. Armand, P. Borel, P. Ythier, G. Dutot, C. Melin, M. Senft, H. Lafont and D. Lairon, J. Nutr. Biochem., 1992, 3, 333–341 CrossRef CAS.
- S. Hwang, S. Lee, I. S. Ahn and J. K. Yung, Biocatal. Biotransform., 2009, 27, 290–295 CrossRef CAS.
- J. K. Lorenzen, S. Nielsen, J. J. Holst, I. Tetens, J. F. Rehfeld and A. Astrup, Am. J. Clin. Nutr., 2007, 85, 678–687 CAS.
- T. Karupaiah and K. Sundram, Nutr. Metab., 2007, 4, 1–17 Search PubMed.
- K. E. Scholz-Ahrens and J. Schrezenmeir, Int. Dairy J., 2006, 16, 1399–1407 CrossRef CAS PubMed.
- M. Mukherjee, J. Mol. Catal. B: Enzym., 2003, 22, 369–376 CrossRef CAS.
- T. F. Whayne and J. M. Felts, J. Am. Oil Chem. Soc., 1971, 48, A101 CrossRef.
- T. F. Whayne and J. M. Felts, Circ. Res., 1971, 28, 649–654 CrossRef CAS.
- H. Kimura, Y. Futami, S. Tarui and T. Shinomiya, J. Biochem., 1982, 92, 243–251 CAS.
- Y. Li and D. J. McClements, Food Hydrocolloids, 2011, 25, 1025–1033 CrossRef CAS PubMed.
- T. Ranheim, A. Gedde-Dahl, A. C. Rustan and C. A. Drevon, Biochem. J., 1994, 303(1), 155–161 CAS.
- F. Martel, R. Monteiro and C. Calhau, Nutr. Res. Rev., 2010, 23, 47–64 CrossRef CAS PubMed.
- K. Lohner, K. Schnabele, H. Daniel, D. Oesterle, G. Rechkemmer, M. Gottlicher and U. Wenzel, Mol. Nutr. Food Res., 2007, 51, 293–300 CAS.
- S. F. Zhou, L. Y. Lim and B. Chowbay, Drug Metab. Rev., 2004, 36, 57–104 CrossRef CAS PubMed.
|
This journal is © The Royal Society of Chemistry 2014 |